Visit the Endocrine Hypertension: From Basic Science to Clinical Practice , First Edition companion web site at: https://www.elsevier.com/books-and-journals/book-companion/9780323961202 .
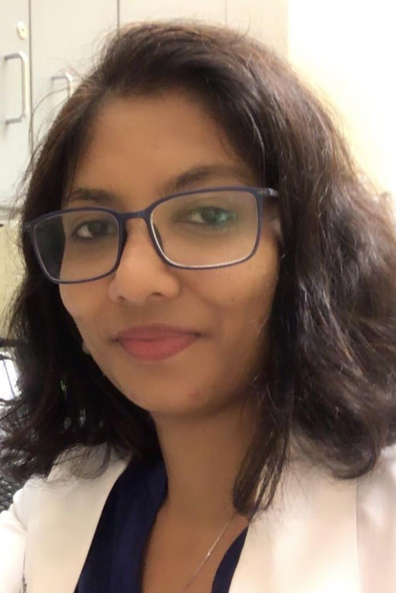
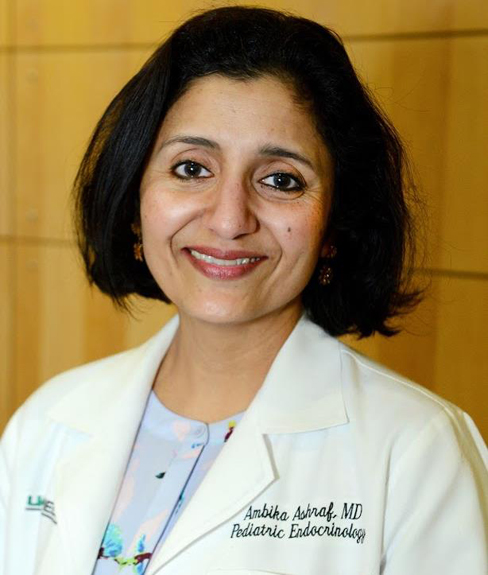
Introduction
The prevalence of childhood hypertension has seen an upward trend in the past 2 decades. In a recent systematic review and meta-analysis conducted by Song et al. the global prevalence of hypertension in children younger than 19 years was estimated to be 4%. In addition, the global prevalence of prehypertension in children was estimated to be 9.67% [ ]. The prevalence of hypertension in children is increasing likely related to the rise in prevalence of obesity. The prevalence has significantly increased from 1.26% in the 1990s to 6.02% in 2015 and has followed a trend very similar to that of global obesity prevalence among adults. As mentioned earlier, the increase in the body mass index (BMI) showed a proportionate increase in the blood pressure (BP), with the prevalence of hypertension being 15.27% among obese children, and 4.99% among overweight children, compared to only 1.90% in children who are normal weight. Similarly, puberty has a significant influence on the BP trajectory, with the prevalence increasing at the age of puberty, reaching a peak at the end of puberty, and finally starting to decline steadily until early adulthood. In 2015, the hypertension prevalence was found to be 4.32% at age 6 years, peaking at 7.89% around puberty at the age of 14 years and 3.28% among those aged 19 years [ ]. An elevated BP in childhood progresses to hypertension in young adulthood with the progression occurring at 5.9% over a 2-year period [ ].
Definition of hypertension : Hypertension in pediatric patients is defined as a blood pressure that is greater than the 95 th percentile for age, gender, and height. A new criterion for the diagnosis of hypertension in children has been proposed by the recent Hypertension Canada (2020) guideline, where a BP threshold of 120/80 mmHg were suggested for children aged 6–11 years and a threshold of 130/85 mmHg for children aged 12–17 years [ ]. In addition to these static cut offs, the Hypertension Canada guideline continued to follow the blood pressure percentile cut offs proposed by previous [ ] and current guidelines (systolic or diastolic blood pressure greater than or equal to the 95 th percentile for age, sex, and height) [ ]. However, they simplified the staging of hypertension in children, and classified hypertension in children as stage 1 if
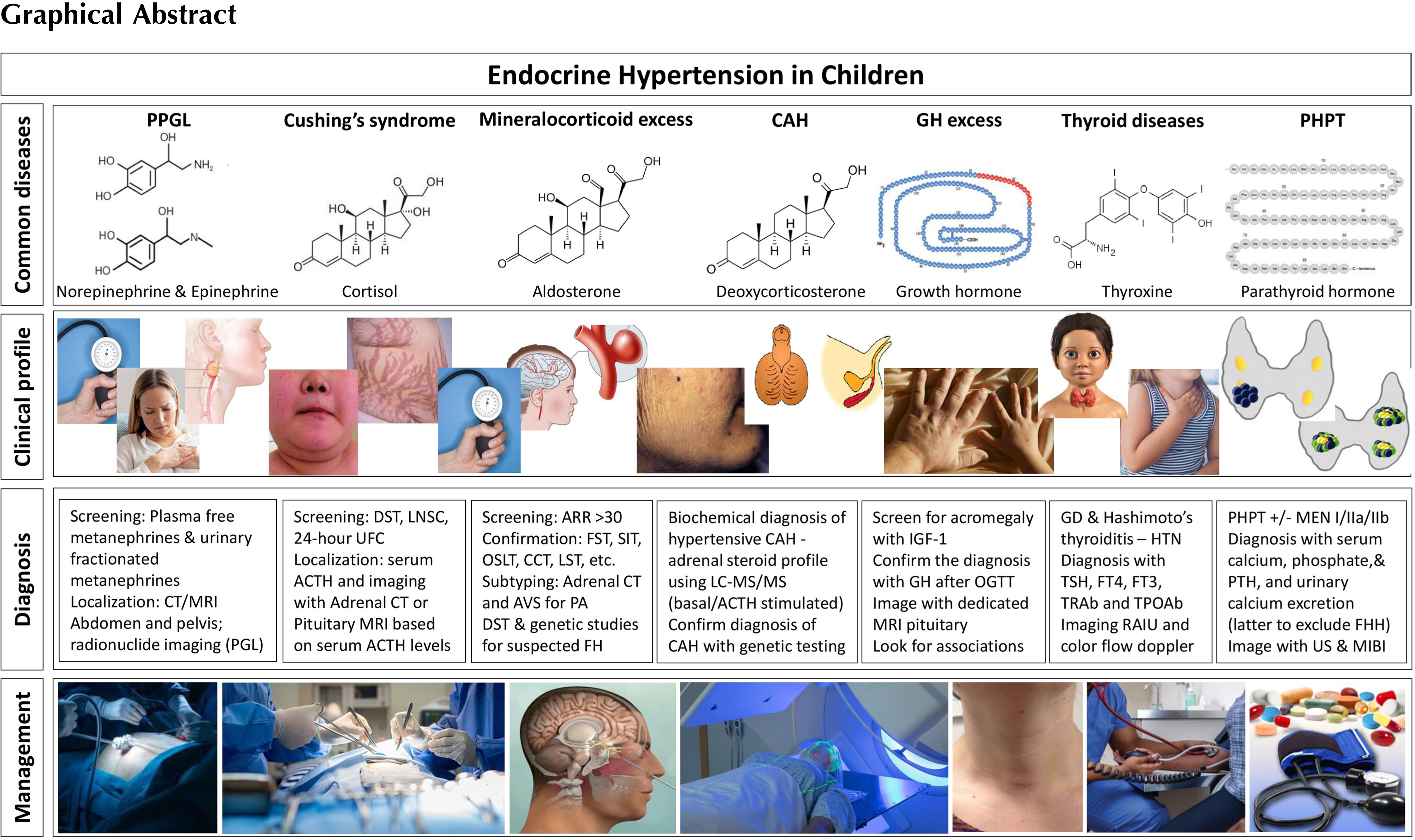
The longitudinal data suggested an improved identification and risk stratification of those children at increased risk for adult cardiovascular disease [ , ]. The United States Preventive Services Task Force stated that the current evidence is insufficient to recommend screening asymptomatic children and adolescents for essential hypertension [ ]. However, the recent Canadian and French guidelines recommend to regularly measure BP in children greater than 3 years [ , ]. Investigations for secondary hypertension should be done in children regardless of the age at presentation, as nearly two-thirds of the hypertension in this age group has secondary hypertension of renal or cardiac origin.
Primary versus secondary hypertension in children
Hypertension can be either primary or secondary. Primary hypertension is generally associated with obesity in genetically predisposed individuals [ ].
Among children presenting with a diagnosis of hypertension, more than 60% have a secondary cause. Renal and renovascular diseases are the leading causes of secondary hypertension in children with renal causes accounting for 34%–79% and renovascular diseases contributing to 12%–13% of childhood hypertension [ , ]. Endocrine causes are relatively rare and contribute to the etiology of pediatric hypertension at a rate of 0.05%–6% [ ].
Secondary hypertension presents more commonly among young children compared to adolescents and adults. Secondary forms of hypertension in children present as severe hypertension and are mostly treatable when the underlying cause is identified. Table 18.1 summarizes the myriad of disease conditions responsible for secondary hypertension in the pediatric age group [ ]. Unlike primary hypertension which peaks at puberty, secondary hypertension can either peak at the first 6 years of life due to congenital abnormalities or respiratory abnormalities due to premature birth and again peaks at an older age due to acquired conditions [ ].
Organ system | Disease conditions |
---|---|
Renal | Glomerulonephritis, congenital anomalies, nephrotic syndrome, hydronephrosis, nephropathy, renal artery stenosis, renal dysplasia, Wilms’ tumor, polycystic kidney disease, hemolytic uremic syndrome, nephrolithiasis, henoch-schonlein purpura, renal trauma |
Respiratory | Chronic lung disease, bronchopulmonary dysplasia |
Cardiac | Coarctation of aorta, patent ductus arteriosus |
Vascular | Renal artery stenosis, renal vein thrombosis, arteriovenous fistula |
Endocrine | Disorders of adrenal gland and abnormal synthesis of adrenal hormones: Cushing’s syndrome, pheochromocytoma, aldosterone producing adenoma, familial hyperaldosteronism, primary glucocorticoid resistance, adrenal neuroblastoma, congenital adrenal hyperplasia due to 17α-hydroxylase deficiency and 11β-hydroxylase deficiency, Liddle syndrome Others: Thyroid and parathyroid disorders (hyperthyroidism, hypothyroidism and primary hyperparathyroidism), excessive growth hormone secretion, diabetes mellitus, hypercalcemia |
Autoimmune | Systemic lupus erythematosus, juvenile ankylosing spondylitis, antineutrophil cytoplasmic antibody-associated vasculitis |
Hematological | Sickle cell disease |
Gastrointestinal | Gastroschisis |
Neurological | Severe intraventricular hemorrhage, brain tumor, hydrocephalus, atrioventricular malformation, neural tube defect, Arnold Chiari malformation, Guillain-Barre syndrome |
Sleep disorders | Obstructive sleep apnea, narcolepsy |
Medications | Steroids, adrenocorticotropic hormone (ACTH), oral contraceptives, anabolic steroids, nicotine, caffeine, sympathomimetics |
Others | Williams syndrome, Turner syndrome, Leigh syndrome, heavy metal exposure including cadmium, lead, and phthalates, following orthopedic procedures |
Endocrine hypertension in children
Endocrine hypertension could be due to an excessive production or enhanced responsiveness to hormones including catecholamines, mineralocorticoids, cortisol, and parathyroid hormones. Thyroid hormones and growth hormone disorders can lead to secondary hypertension when in excess or deficient. Other predominant cause of secondary hypertension is insulin resistance [ ]. Early identification and treatment of the underlying hormonal abnormality could prevent progression to organ damage from endocrine hypertension. A majority of cases of endocrine hypertension is related to adrenal gland pathology and an abnormal synthesis of adrenal hormones.
Clinical presentation of endocrine hypertension in children
Catecholamine producing tumors
Pheochromocytomas and paragangliomas in children
Catecholamine secreting tumors arising from chromaffin cells of the adrenal medulla are termed pheochromocytomas, whereas tumors arising outside the adrenals are called paragangliomas (PGLs) and they are usually grouped together as pheochromocytomas and paragangliomas (PPGL). Pheochromocytomas are the more common than PGLs. PGLs may arise anywhere along the sympathetic chain, near the aorta at the level of inferior mesenteric artery, peripheral adrenal area, urinary bladder, ureteral walls, thoracic cavity, or rarely in the cervical region. PPGLs are rare with an annual incidence of ∼1 in 300,000, and 20% of these cases occur in children and are more likely to be malignant [ , ]. PPGL accounts for ∼1% of pediatric hypertension.
Unlike adults who present with paroxysmal hypertension, children with PPGL usually present with sustained severe hypertension, which is seen in 60%–90% of cases [ ]. With markedly elevated BP, children often present with symptoms of hypertensive encephalopathy. Symptoms usually vary based on the type of catecholamine hormone secreted by the tumor. The classic triad of symptoms includes palpitations, diaphoresis, and headaches, which can be episodic in nature. Common symptoms include headache, palpitation, sweating, pallor, nausea, flushing, anxiety, weight loss, polyuria, polydipsia, and visual disturbance. In addition to these, children with epinephrine secreting tumors may present with hyperglycemia and hypertensive crisis due to catecholamine excess. Dopamine secreting tumors may be asymptomatic except for the mass effect. Head and neck PGLs can lead to dysphagia, hoarseness, and hearing disturbances from mass effect [ ] and are rarely associated with hypertension.
Germline mutations are found in 70% of children <10 years of age and 51% of children between ages 11–20 years [ , ]. PPGLs are commonly familial with 30%–40% of cases having a hereditary background with a multifocal origin involving mutations in several susceptibility genes which are divided into two cluster groups. Cluster group 1 include a noradrenergic phenotype (secreting norepinephrine and is associated with an increase in plasma or urinary normetanephrine), mostly located in extraadrenal areas and includes 2 subclusters: Cluster 1 A has gene mutations in the Krebs cycle related genes including mutations of the genes encoding the succinate dehydrogenase (SDH) complex including SDHA, SDHB, SDHC and SDHD , malate dehydrogenase 2 ( MDH2 ), fumarate hydratase ( FH ), and others . Cluster 1B is due to gene mutations in the hypoxia signaling pathway including Von Hippel-Lindau ( VHL ) tumor suppressor, Egl-9 prolyl hydroxylase one and 2 ( EGLN1/2 ), hypoxia-inducible factor 2 alpha ( HIF2A ), and others. Cluster group 2 includes an adrenergic phenotype (secreting epinephrine and is associated with an increase in plasma or urinary metanephrines) and comprises mutations in the rearranged-during-transfection ( RET ) proto-oncogene, neurofibromatosis type 1 tumor suppressor gene ( NF1 ), genes encoding transmembrane protein 127 ( TMEM127 ), MYC-associated factor X ( MAX ), and Harvey rat sarcoma viral oncogene homolog ( HRAS ), which leads to activation of tyrosine kinase associated signaling pathways. Genetic testing for the most common mutations should be considered to determine accurate diagnosis and prognosis.
Pheochromocytomas are commonly associated with von Hippel-Lindau disease (10%–20%), multiple endocrine neoplasia (MEN) syndromes (MEN-2A and MEN-2B), and rarely associated with other diseases like tuberous sclerosis, Sturge-Weber syndrome, ataxia-telangiectasia, and neurofibromatosis. Paragangliomas are commonly associated with Carney dyad and Carney triad syndromes where they are associated with gastrointestinal stromal tumors. The Carney triad and Carney dyad associations have heterogeneous genetic etiology and majority of patients with Carney dyad syndrome have mutations of the SDH complex. Mutations of the SDH complex are associated with paragangliomas as well as pheochromocytomas. Familial PGL syndromes are usually due to inactivating mutations in SDHx , leading to dysfunction of complex II in the electron transport chain. Germline mutations especially involving VHL and SDHB genes have been reported in majority of pediatric pheochromocytoma cases [ , ], and usually present with multifocal, recurrent, and malignant disease [ ]. VHL- and SDHx-associated tumors are typically noradrenergic, whereas those associated with MEN2, NF1, and sporadic tumors are often adrenergic.
Diagnosis
Twenty-four-hour urinary fractionated metanephrines (normetanephrine and metanephrine) and catecholamines (norepinephrine, epinephrine, and dopamine) : Most patients with pheochromocytoma or paraganglioma have increased urinary excretion of these substances. False-negative results are rare, and hence, this is the first-line investigation to exclude pheochromocytomas and catecholamine-secreting paragangliomas. The 24-h urine sample measurements are usually more stable. It is essential to measure the urinary creatinine in the sample to verify a complete 24-h urine collection [ ]. False-positive results can occur with use of medications such as acetominophen, α- and β-adrenergic blockers, tricyclic antidepressants, monoamine oxidase inhibitors, and sympathomimetic agents (nicotine, ephedrine, pseudoephedrine, cocaine).
Plasma fractionated metanephrines : This diagnostic test is helpful and convenient for patients with strong risk factors for a catecholamine-secreting tumor (i.e., those with known pathogenic variants for familial pheochromocytoma). This test is slightly more sensitive than 24-h urinary fractionated metanephrines, however, has a false-positive rate of 15%. It is ideal to draw the blood sample from an intravenous catheter with the patient supine for 30 min before the blood test, calm and relaxed, fasted and not had caffeine or strenuous physical activity for at least 8–12 h before testing.
Radiologic evaluation to locate the tumor : Once a biochemical diagnosis of pheochromocytoma or catecholamine-secreting paraganglioma is established, imaging is essential. Further evaluation by computed tomography (CT) or magnetic resonance imaging (MRI) of the abdomen and pelvis is recommended. Abdominal ultrasound is not recommended due to lack of imaging sensitivity to localize pheochromocytoma or paraganglioma. In those with positive biochemical tests, but with a negative CT or MRI scan of the abdomen and pelvis, radionuclide imaging (i.e., 123 I-MIBG or PET/CT or 68 Ga-DOTATOC scan) is indicated to evaluate for paragangliomas or those children with catecholamine-secreting extra-adrenal tumors.
Genetic testing is also recommended in children, since 40%–50% of pheochromocytomas and catecholamine-secreting paragangliomas are associated with underlying genetic abnormalities. Open surgery, or laparoscopic adrenalectomy is the treatment of choice for children with PPGLs. Preoperative correction of tachycardia with β-blockers following adequate α-blockade to control hypertension is required. For further reading on PPGL, please refer Chapter 11, Chapter 12 .
Cushing’s syndrome in children
Cushing’s syndrome results from excessive plasma cortisol due to exogenous sources or endogenous secretion, and is uncommon in pediatric clinical practice. Excessive production of cortisol from endogenous causes includes ACTH secreting pituitary adenoma causing Cushing’s disease (CD); adrenal lesions (adenoma, carcinoma, or bilateral adrenal hyperplasia) causing adrenal Cushing’s syndrome; ectopic ACTH secretion (EAS) from bronchial or thymic carcinoid, other neuroendocrine tumors of pancreas and gut; small cell carcinoma of lung, medullary carcinomas of thyroid, and pheochromocytoma [ ]. Exogenous administration of glucocorticoids for a prolonged period is the most common cause of Cushing’s syndrome [ , ].
In children less than 7 years, adrenal Cushing’s syndrome is the common cause, whereas in children more than 7 years, Cushing’s disease is the common cause (accounting for nearly 75% of cases). EAS caused by ACTH secretion from nonpituitary tissues is an unusual diagnosis in children. It accounts for less than 1% of all the Cushing’s syndrome cases in adolescent age group [ ].
EAS is more commonly associated with hypertension in the pediatric age group compared to other forms of endogenous hypercortisolemia. Almost half of the affected children present with hypertension with occasional progression to heart failure. Prolonged hypercortisolism results in increased plasma volume, increased peripheral vascular resistance, increased cardiac output, and increased pressor response to epinephrine and angiotensin II, resulting in hypertension. Glucocorticoids activate renin–angiotensin–aldosterone system (RAAS), leading to enhanced mineralocorticoid activity, excessive activation of vasoactive substances, and suppression of vasodilation. Markedly increased cortisol levels can activate the mineralocorticoid receptor (MR) directly as cortisol binds to, and activates the MR, and can increase the deoxycorticosterone synthesis. The increased cardiac output due to hypercortisolemic response results predominantly in an increase in systolic BP [ ]. Hypertension can also be caused by a central nervous system (CNS) dysregulation via glucocorticoid and mineralocorticoid receptors [ ]. Biochemically, hypernatremia and hypokalemic metabolic alkalosis can occur.
Clinical presentation of Cushing’s syndrome is more pronounced in infants than in older children [ , , ]. In growing children, one of the hallmarks of Cushing’s syndrome is the excessive weight gain accompanied by poor linear growth. Children with Cushing’s syndrome or Cushing’s disease present with round and flushed face with prominent cheeks (moon like face) and truncal obesity. Other clinical features are precipitous weight gain, muscle weakness, fatigue, immune suppression, irregular menses, depression, anxiety, osteoporosis, hyperglycemia, and headaches.
Almost 65% of Cushing’s syndrome in younger children are caused by an adrenal tumor, mostly due to adrenal carcinomas [ ]. Tumors may secrete androgens and mineralocorticoids in addition to cortisol, which manifest with virilization. Children with adrenal tumors can present with acne, hirsutism, pubic hair, deepening voice (in boys) and enlarged clitoris (in girls). Adrenal carcinomas often have rapid progression, while adenomas have an insidious course.
Diagnostic evaluations
An accurate and timely diagnosis is important for initiating appropriate treatment for patients with Cushing’s syndrome. Children with a normal hypothalamic-pituitary-adrenal (HPA) axis exhibits a normal diurnal profile for cortisol, i.e., higher levels of cortisol are seen early in the morning and lower levels in the evening. In contrast, patients with CS demonstrate an altered rhythm of HPA axis with a disrupted diurnal cortisol profile. Thus, lack of reduction in serum cortisol in the late evening is a diagnostic marker for CS in children. A midnight serum cortisol value >7 mcg/dL has a high sensitivity and specificity for diagnosis of CS, but not preferred due to the requirement for an inpatient stay.
Initial screening : Initial screening poses a significant clinical challenge, and is aimed at establishing hypercortisolism. The three recommended screening tests are, obtaining at least two urine collections for urinary free cortisol (UFC), or two salivary collections for late night salivary cortisol, and/or an overnight dexamethasone-suppression test (DST) [ ].
Urine free cortisol : A value greater than 4 times the upper limit of normal is typically associated with Cushing’s syndrome. UFC measurements are cumbersome with issues related to proper sampling and confounding factors such as variations in fluid intake and renal function. False-positive UFC can occur due to pseudo-Cushing states (e.g., stress, severe obesity, chronic exercise, depression, poor diabetes control, anorexia, narcotic use, anxiety, malnutrition, and high-water intake etc.). False-negatives can result due to inadequate sample collection of urine.
Salivary cortisol : Late-night salivary cortisol (two measurements) is an accepted initial screening test in adults with CS with excellent diagnostic sensitivity and specificity comparable to urine free cortisol. In children age- and gender-specific reference values for late night salivary cortisol and cortisone are lacking. A recent study has reported [ ] a bedtime salivary cortisol above 2.4 nmol/L (measured by LC-MS/MS) can be considered as a positive screening test for CS in children and does not require age- and gender-specific cut-off levels. According to Lodish et al. a salivary cortisol of >0.13 mcg/dL is considered a positive screening test [ ].
Overnight dexamethasone-suppression test ( O DST) : ODST involves administering 1 mg of dexamethasone overnight (at 11 p.m.) and drawing a blood sample for cortisol measurement at 8 a.m. Patients with Cushing’s syndrome will demonstrate inadequate cortisol suppression (i.e., 8 a.m. serum cortisol >1.8 mcg/dL).
Once the diagnosis of hypercortisolism is confirmed, the next step is to distinguish ACTH-dependent from the ACTH-independent disease. A morning plasma ACTH ≥29 pg/mL in children may indicate ACTH-dependent CS with a sensitivity of 70% [ ].
A high-dose dexamethasone suppression test is recommended to differentiate CD from ectopic ACTH secretion and adrenal causes of CS. Plasma cortisol is measured at 9 a.m. the morning before, followed by dexamethasone 120 μg/kg (maximum dose 8 mg) is administered at 11 p.m., and thereafter the plasma cortisol is again measured at 9 a.m. the morning following the dexamethasone. Patients with ectopic Cushing’s disease will not have ACTH suppression response to high-dose dexamethasone in contrast to patients with CD. In children, a 20% cortisol suppression from baseline had a sensitivity and specificity of 97.5% and 100% for differentiating CD from adrenal CS [ ]. Even though an ovine CRH stimulation test can also be used for the differentiation of CD from ectopic ACTH secretion, these are only available in specialized tertiary care centers [ ].
Patients with certain conditions such as depression, obesity, or poorly controlled diabetes can present with pseudo-Cushing’s syndrome characterized by some of the symptoms and mildly abnormal cortisol levels that overlap with those seen in Cushing’s syndrome [ ]. With increasing prevalence of obesity, diagnosis of Cushing’s syndrome poses challenges as most patients with obesity present very similar to Cushing’s phenotype [ ]. Cushing’s syndrome should be suspected in children who have hypertension, poor linear growth, obesity, and purplish striae [ , ].
Once the biochemical workup is confirmatory for hypercortisolism, the next step is localization of the tumor by diagnostic imaging: Adrenal CT scan is used in localizing adrenal causes of Cushing’s syndrome. In the case of CD, pituitary MRI in thin sections (1–2 mm) with high resolution, and with contrast (gadolinium) is performed. Bilateral inferior petrosal sinus sampling (IPSS) has been used for the localization of a pituitary source of hypercortisolism, especially in cases where a lesion is not visible on pituitary MRI. High-resolution flourodeoxy glucose positron emission tomography (FDG PET) can be used for locating small functioning corticotroph adenomas. In the case of ectopic CS, a CT or MRI scan of the neck, chest, abdomen, and pelvis may be needed. Labeled octreotide scanning, positron-emission tomography (PET), and/or 68 Ga-DOTATATE PET/CT are used in specialized centers for localization of an ectopic ACTH source.
Transsphenoidal surgery (TSS) of the pituitary adenoma remains the treatment of choice for CD. These children are at risk for complications involving syndrome of inappropriate antidiuretic hormone secretion, hypopituitarism (i.e., diabetes insipidus, central hypothyroidism, hypogonadism, growth hormone deficiency), bleeding, infection, and pituitary apoplexy. Hence, it is essential to consult a surgeon with experience in management of these potential postoperative complications. Medical therapy after an unsuccessful pituitary surgery for CD is very limited in children and involves off label use of some drugs. Children with adrenal Cushing’s syndrome often require surgical resection of the adrenal lesion. However, children with Cushing’s syndrome often run a risk of residual hypertension even after a significant improvement following surgical correction. For further reading on Cushing’s syndrome, please refer Chapter 13, Chapter 14 .
Biochemical phenotype of mineralocorticoid excess in children
The RAAS plays an essential role in maintaining normal blood pressure. Renin is released from the juxtaglomerular apparatus in response to hypovolemia and hyponatremia, subsequently resulting in angiotensin II-mediated secretion of aldosterone from the zona glomerulosa of the adrenal gland. Aldosterone secretion can be regulated by the RAAS or directly by a rise in serum potassium. Aldosterone binds to the MR and activates specific amiloride-sensitive epithelial sodium channels (ENaC) and the sodium-potassium ATPase pump (Na + -K + -ATPase) to promote the sodium reabsorption, water retention, increased intravascular volume, and potassium excretion. Therefore, the phenotypic presentation of mineralocorticoid excess can result from overproduction of mineralocorticoids, overstimulation of mineralocorticoid receptors, or overactivity of the epithelial sodium channels.
See Table 18.2 for an approach to interpreting renin and aldosterone levels. Patients can present with low-renin and high-aldosterone hypertension, the main differential diagnoses include primary aldosteronism caused by aldosterone-producing adenomas and familial aldosteronism as in glucocorticoid-remediable aldosteronism (GRA). Patients can also present with low-renin and low-aldosterone hypertension, the main differential diagnoses include apparent mineralocorticoid excess, primary generalized glucocorticoid resistance (Chrousos syndrome), Liddle syndrome, Geller syndrome, and congenital adrenal hyperplasia (CAH) due to 17α-hydroxylase and 11β-hydroxylase deficiency [ , ]. In the case of Gordon syndrome, patients can present with normal plasma aldosterone and low renin.
Phase 1: Ensure the diagnosis of true hypertension by ruling out white coat hypertension |
|
PHASE 2 : Screen for appropriate underlying cause, end-organ damage, and associated comorbidities |
|
PHASE 3 : Define/identify the abnormalities identified in phase 2 with extensive and focused evaluation |
Abdominal CT scan or ultrasound—if clinical presentation warrants |
If suspect ed secondary hypertension: Do FT4, and TSH. Do evaluation of the RAAS by renin level or plasma renin activity, and aldosterone concentration in patients with resistant hypertension and hypokalemic alkalosis
|
Catecholamine assessment if clinical presentation warrants: Plasma-free metanephrines and/or urinary fractionated metanephrines |
Noninvasive renal imaging: VCUG, renovascular CT or MRA |
PHASE 4 : Arrive at a definitive diagnosis to offer medical and/or surgical cure |
Renal biopsy, renal angiography, genetic testing for specific mutation, MIBG scan, renal vein renin collection – depending on the condition |
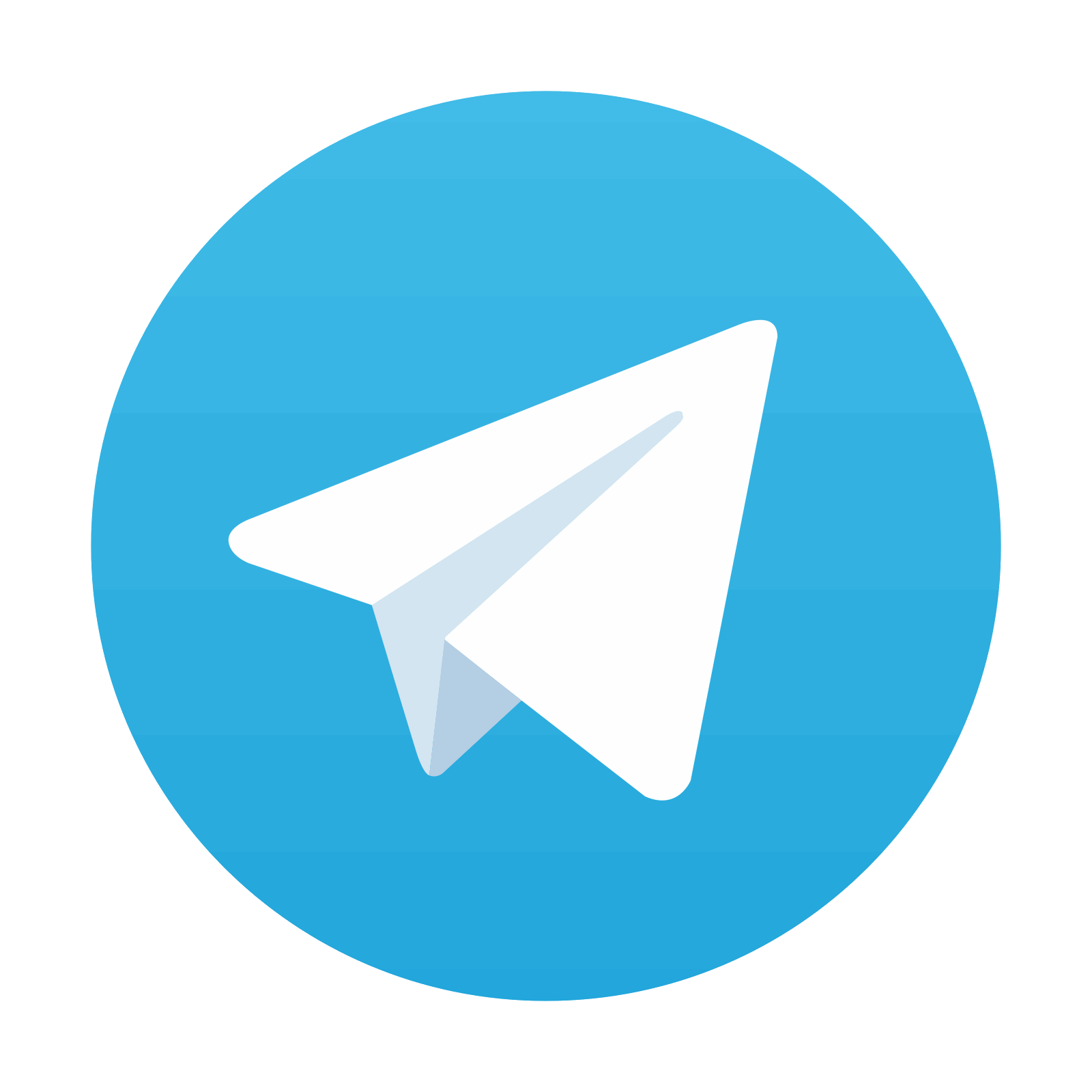
Stay updated, free articles. Join our Telegram channel

Full access? Get Clinical Tree
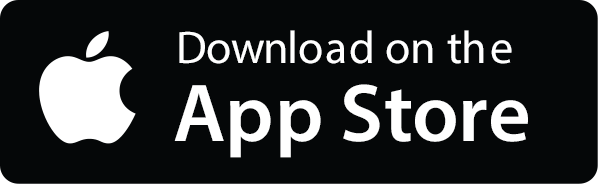
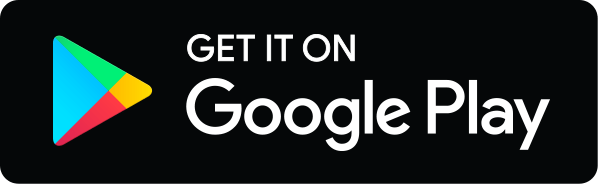
