Source: Adapted from Ruppert, Fox, and Barnes [7].
The majority of EDC studies has centered on the role of contaminants on steroid hormone function in vertebrate animals and has begun to examine the effects in molluscs only within the past decade. In order to fully understand the breadth of these effects in molluscs, we must first understand the structure, function, and relatedness of the mollusc endocrine system. The biochemical and physiological processes responsible for reproduction in molluscs is a complex series of secretions dependent on the interaction between various neuropeptide hormones and steroid hormones.
Physical factors such as nutrition, photoperiod, temperature, and tidal cycle (for marine species) all influence the regulation of endocrine systems in molluscs [8–10]. In addition, there is some evidence that conspecifics play an initiating or stimulatory role in reproduction for select species [11,12]. The endocrine system of molluscs involves a complex aggregation of chemical messengers that are secreted by both specialized neurosecretory cells and endocrine glands including the gonads. The types of hormones that are active include catecholamines, glycoproteins, modified amino acids, peptides, polypeptides, prostaglandins, retinoic acid, and steroid hormones. These varied hormones are dependent on one another for function and signaling capacities as well as feedback regulation of downstream processes (see Figure 6.1). Their end function is carried out either through the direct action of these compounds as signaling molecules or through their interaction with nuclear receptor proteins and/or alteration in reproductive or developmentally related gene transcription.
FIGURE 6.1 Model of mollusc endocrine regulation, metabolic, and physiological processes. Abbreviations include: ASO = accessory sex organ; SMF = sexual morphogenic factor; ELH = egg laying hormone.
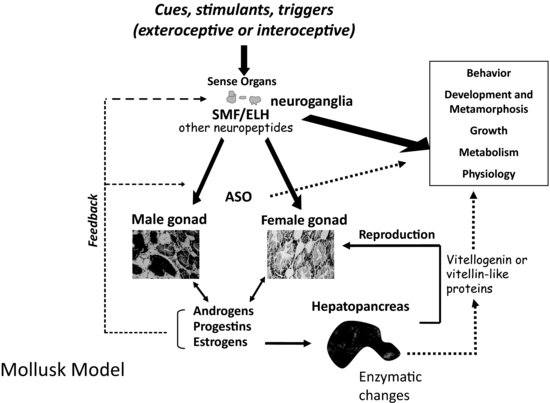
The peptide and polypeptide hormones are the most common within the phylum [3]. These hormones function mostly in regulating gender-specific reproductive behavior and are produced by neurosecretory cells or ganglia. In Aplysia (see Figure 6.2), there are at least five types of cells or organs that regulate ovulation and egg laying. These include the cerebral, pleural, and pedal ganglia of the neural head ring; the bag cells found in the abdominal ganglia; the ovotestis, the hermaphroditic duct; and the accessory sex organs (including male and female gonad structures). In contrast, Lymnaea has at least six types of cells or organs involved in egg laying. They include the dorsal bodies, the caudodorsal cells (CDCs), the lateral lobes, the hermaphroditic gonad, the hermaphroditic duct, and the accessory sex organs [8].
FIGURE 6.2 Internal organs and neural ganglia of Aplysia californica [Opisthobranchia]. (Ag = abdominal ganglia; AG = albumen gland; Cg = cerebral ganglia; H = heart; Mg = mucous gland; Ot = ovotestis; Plg = pleural ganglia; Peg = pedal ganglia; S = spermatheca; RH = red hemiduct.) Source: Photographs P. McClellan-Green.
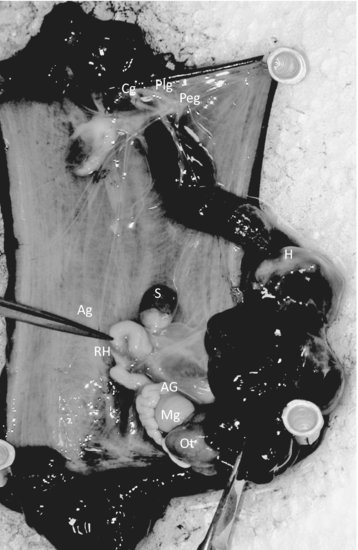
Secretions produced by the cerebral, pedal, pleural, and abdominal ganglia include bioactive peptides (neuropeptide hormones) that control numerous aspects of reproduction, including sexual differentiation, the growth of accessory sex organs, the initiation of reproduction, and the development of the gametes. Some of the neuropeptide hormones found in molluscs are also found in vertebrates. For instance, gonadotropin-releasing hormone (GnRH) is a decapeptide hormone secreted in vertebrates by cells within the hypothalamus. GnRH regulates the secretion of gonadotropin from the pituitary and hence plays a critical role in the initiation and maintenance of reproductive function. This peptidic messenger and/or its analogs have been identified as a dodecapeptide in numerous mollusc species, including the octopus (Octopus vulgaris) and several gastropods, including Aplysia californica, Helisoma trivolvis, Lymnaea stagnalis, and Lottia gigantean [13,14]. Meanwhile, the GnRH receptor has been identified in the bivalves Crassostrea gigas and Mytilus edulis [15–17]. The GnRH receptor is expressed in the gonad of C. gigas during reproductive recrudescence but not during sexual quiescence, indicating a possible function in reproduction. It has also been identified within the neurons at various stages of larval development; however, the presence of the hormone ligand has not been reported.
Other peptides identified in gastropods play a role in stimulating growth or function during reproduction. For instance, insulin-like peptides, which are involved in the control of body growth and associated metabolic processes, have been identified in both Lymnaea stagnalis and Aplysia californica [18]. These peptides stimulate adenylyl cyclase and guanosine 5′-triphosphate (GTP)-binding activity of G-proteins similar to that of insulin and insulin-related factor 1 [19,20]. Conopressin, which is in the vasopressin/oxytocin family of peptides, stimulates contractions of the vas deferens and may also inhibit the firing rate and duration of the caudodorsal cell hormones (CDCH-I, CDCH-II) involved with egg laying [17,21]. The CDCHs are 36 amino acid peptides that induce ovulation and egg-mass formation and act on neuronal circuits that control egg laying in Lymnaea [22]. A similar and related neuropeptide is the egg-laying hormone, which functions to modulate reproduction in Aplysia [23]. Homologs of these egg-laying neurohormones have been identified in prosobranchs, including Busycon canaliculatum, Concholepas concholepas, and Tegula atra [24].
Other mollusc neurohormones involved in reproduction include FMRFamide, neuropeptide Y, pedal peptide, the FMRFamide copeptide SEEPLY, the Lymnaea form of neuropeptide tyrosine, and 5-hydroxytryptamine (5HT, serotonin). Each of these hormones has a function in male copulatory behavior [25–27]. The neuropeptide APGWamide also plays a role in male copulation activity but additionally is expressed in abdominal tissue of imposex female Ilyanassa obsoleta, indicating other functions within the organism possibly related to the development of secondary sexual characteristics [28–30]. This neuropeptide has been identified in several gastropod species while the highly related peptides, RPGWamide, KPGWamide, TPGWamide, YFMRFamide, nLRFamide, MNYLAFPRMamide, EDPFLFamide, and APGWamide-like peptides have been identified in numerous bivalves. In addition, the related peptide GWamide has been identified in cephalopods [27,31–33]. In each case these neuropeptide hormones exert some control over male reproduction via developmental regulation or signaling.
While not neurohormones, an entire series of chemical signaling molecules known as pheromones have been identified over the past decade. These pheromones regulate mating behavior in molluscs, and have been identified specifically in A. californica and A. brasiliana. They include the proteins attractin, enticin, seductin, and temptin [34–37]. These pheromones are secreted or eluted not only from the albumen gland of breeding animals but also from their eggs and egg capsules. Buresch et al. [38] identified similar compounds in squid, Loligo pealeii. Since these are not considered hormones, they would not necessarily be examined during studies of EDC exposure; however, we must remember that they are responsible for mating and the formation of egg-laying aggregates or clusters of mating organisms.
The steroid hormones comprise the other major component of the endocrine system. As with most sexually reproductive organisms, the gonads of molluscs function as the major source of steroids. These hormones control physiological activities, including gamete production, synchronization of gonadal and accessory sex gland activities, feedback control of gonadotropic center(s), and control of reproductive behavior. Many vertebrate-type steroids, including estradiol, testosterone, dihydroxytestosterone, pregnenolone, progesterone, and cortisol, have been identified in molluscs (see Figure 6.3) [39].
FIGURE 6.3 Steroid pathways and metabolites in molluscs. Source: Adapted from Gottfried and Dorfman [44].
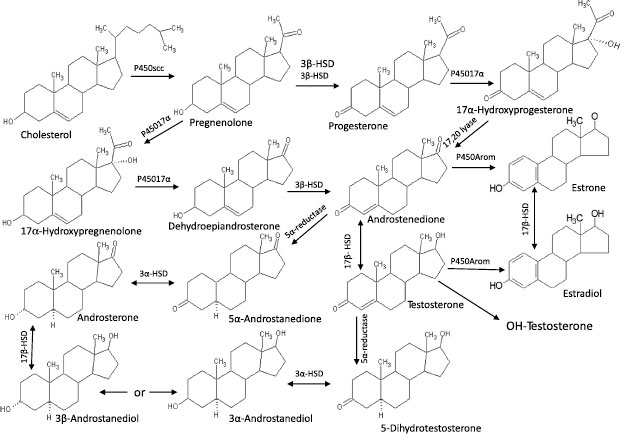
One of the earliest studies suggesting hormonal control of reproduction in molluscs was an abstract published in 1929 by Enrico Sereni [40], in which he hypothesized the presence of unknown endocrine compounds that controlled the production of the hectocotylus in Octopus. In 1930, Steidle [41] suggested the presence of female sex hormones in molluscs, and Hagerman et al. [42] reported in 1956 on the occurrence of estrogens in marine invertebrates. Decades later the in vitro synthesis of vertebrate-type steroids from substrate precursors was reported using tissue homogenates from the black slug [43], giant banana slug [44], oyster [45], and sea scallop [46]. These studies all supported the capacity of molluscs to produce vertebrate-like steroids through the function of specific enzymes. Since that time the isolation of vertebrate-type steroids and the presence of steroid biosynthetic enzymes have been identified in each of the major classes of Mollusca, including bivalves [47–51], cephalopods [52–54], and gastropods [55–57]. These steroids and, more recently, the enzymes responsible for their production have been identified in a variety of molluscs (see Table 6.3). The structures of mollusc steroids are analogous to those of vertebrate steroids and presumably their roles in reproduction are comparable.
Table 6.3 Sex steroids synthesized in molluscs
The role of sex steroids in mollusc was clouded for many years. In the 1980s, aquaculture operators used immersion of oyster spat in either methyltestosterone or 17β-estradiol to produce unisex clutches of oysters [58]. This process along with changes in the endogenous levels of estrogens observed during the reproductive cycle of oysters and scallops and variable levels of both estradiol and progesterone in mussels provided strong evidence for the role of steroid hormones in reproduction and development. While the major steroid forms, such as progesterone, pregnenolone, androstenedione, testosterone, and estradiol, were all identified in the tissues, there was contrasting evidence for the presence of functional steroid-hormone receptors. In an effort to prove the presence and function of steroid receptors in molluscs, investigators have conducted ligand-binding assays, immunochemical staining of receptor or receptor-like proteins, and cloning and sequencing of receptor genes.
Gagné et al. [59] was one of the first to report the presence of an estrogen receptor (ER) in mollusc specifically within the tissues of Elliptio complanata. Since then, the presence of an ER or estrogen-like receptor has been reported in other bivalves, including scallops (Patinopecten yessoensis, Placopecten magellanicus), mussels (Mytilus edulis, Mytilus galloprovincialis, Elliptio complanata), and oysters (Crassostrea gigas) [59–64]. In fact, two separate ERs, ERα and ERβ, were identified in protein extracts of Mytilus galloprovincialis, and an ERβ-like protein was identified in both Elliptio complanata and Mytilus edulis. The identity of these proteins as ERs was confirmed through high-affinity estradiol binding assays, the use of known receptor antagonists including tamoxifen, flutamine, and RU486 [65], as well as the immunoreactivity of anti–human ER antibodies to target proteins in bivalve tissues. Stefano et al. [61] also cloned a small (266 bp) gene fragment from the pedal ganglia of Mytilus edulis that possessed 100 percent identity with the human ERβ gene.
Discerning the presence of steroid receptors in other molluscs including gastropods is slightly more complex. Thornton et al. [66] was the first to describe a molluscan ER ortholog using the sea hare Aplysia californica. This receptor, while closely aligned with the human ERα, based on its sequence, did not bind the substrate 17β-estradiol (E2). In fact, this putative ER acted as a strong constitutive transcriptional activator in the absence of E2 and other steroid hormones. Constitutive ER activation presumably occurs due to the evolution of structural modifications in the receptor that stabilize the active conformation of the protein. A similar relationship was observed following the determination of the octopus ER.
Keay et al. [67] showed that the octopus ER was nonresponsive to estradiol and other steroid hormones. The octopus ER also exhibited a high constitutive activity similar to that observed in Aplysia, portending a common physiological yet nonnuclear function in molluscs. This hypothesis was supported through further studies of the estrogen levels and ER expression in other gastropods, including Thais clavigera, Marisa cornuarietis, Nucella lapillus, and Ilyanassa obsoleta [68–71]. In each species, sequence identity confirmed the presence of an ER ortholog, but estradiol and other steroid assays confirmed the lack of ligand binding, especially in Thais clavigera and Marisa cornuarietis. Matsumoto et al. [72] and Croll and Wang [65] performed analogous studies using the Pacific oyster, Crassostrea gigas, and the scallop, Placopecten magellanicus, respectively. Both laboratories reported sequence similarity but a lack of steroid ligand binding.
Demonstration of the presence of an androgen receptor (AR) in molluscs has thus far eluded investigators. Administration of testosterone to molluscs, including Nucella lapillus, Hinia reticulatus, Ilyanassa obsoleta and Marisa cornuarietis, results in the production of imposex and an increased vas deferens sequence index and/or increased penis length indicating a physiological function for this androgen. Differences in free testosterone levels have been observed during the various reproductive stages in both I. obsoleta and M. arenaria [49,71]. These data point toward a role for androgen signaling during mollusc reproduction; however, no AR has been identified from any species.
Generally, analysis of the functional activity of nuclear receptors across multiple taxa relies heavily on the identification of contact residues that are highly conserved within receptors. These contact points identify critical regions, such as the DNA binding domain or the ligand-binding domain. Unlike the ER studies, attempts to use amplification primers based on the sequence of the DNA binding domain or the ligand-binding domain of mammalian, teleost, or other species has proven unsuccessful in the isolation of the molluscan AR. The latter region, the AR ligand-binding domain, varies widely between species in respect to its relative affinity for steroidal androgens. Primary physiological ligands identified thus far include dihydrotestosterone in mammals, 11-ketotestosterone and testosterone in fishes, and androstenedione in the sea lamprey [73–75].
It is highly probable that the molluscan androgen ligand, if it exists, differs still; therefore, designing DNA amplification primers based on the sequence from other species is futile. Attempts to identify the AR using primers based on the DNA binding region also have been unsuccessful. This contrasts with the identification of the ER, where the use of consensus and degenerate primers based on higher-order species has been quite successful. Thus, evidence in the study of the AR points toward the lack of an AR within the phyla and instead toward a nonnuclear or non–receptor-mediated role for these steroids in molluscs.
Another receptor recently discovered that may play a key role in molluscan endocrine disruption is the retinoid X receptor (RXR). Unlike the ER and AR, which bind steroid hormones in vertebrates, the RXR binds 9-cis-retinoic acid [76]. While loosely considered a natural hormone, 9-cis-retinoic acid lacks the multiringed structure typical of steroid hormones. Physiologically, retinoic acids are involved in the processes of cell proliferation, differentiation, homeostasis, and regeneration of tissues. In vertebrates, the RXRs carry out these functions through gene activation by forming heterodimers with various receptors, including retinoic acid receptor, vitamin D receptor, thyroid hormone receptor, peroxisome proliferator-activated receptor, liver-x receptor, and the xenobiotic receptor [77].
In 2004, Nishikawa et al. [78] reported the identification of the RXR from Thais clavigera and described its capacity to bind both 9-cis-retinoic acid and organotins. Organotin compounds are well known as environmental contaminants responsible for the development of imposex in gastropods, leading to the assumption that RXR may be involved in male sexual differentiation in molluscs. Subsequently, the RXR and various RXR isoforms have been characterized in Thais clavigera, Biomphalaria glabrata, Nucella lapillus, and Ilyanassa obsoleta [71,77,79,80]. Expression of this receptor, like the ER, varies throughout the reproductive season, but in this instance higher levels occurred in reproductive males and organotin-exposed females. It was also evident that RXR expression was greater in the regenerating reproductive tissues and accessory sex organs than in senescent tissues. This finding lends support for the theory that the RXR ligand/receptor complex is somehow involved with the development of male secondary sex characteristics, perhaps through its involvement in the regeneration/differentiation of reproductive tissues.
6.3 END POINTS AND BIOMARKERS OF ENDOCRINE DISRUPTION
A “biomarker” is defined as a molecular or cellular event that links exposure to environmental chemicals to a specific health outcome, such as disease or increased risk for disease. Most often biomarkers are used in the elucidation of chronic human diseases, but they also can be applied to the health of terrestrial, estuarine, or marine systems. The concept of biomarkers can be separated into four types. The first type, biomarkers of disease, include characterization of endogenous substances or indicators of a disease through pharmacodynamic processes or genetic end points that subsequently aid in the formulation of disease treatments. Next we have biomarkers of exposure, which include the identification and quantification of the internal concentration of a drug or contaminant. This is followed by biomarkers of response, which include the quantitative determination of pharmacodynamic and toxicodynamic processes. Last are biomarkers of susceptibility, which include the identification of genetic factors that alter the susceptibility of an individual or organism to the adverse effects of drugs or contaminants [81].
Great progress has been made over the past two decades in the identification, development, and validation of biomarkers for both human and ecosystem assessment. The EDC testing paradigm routinely employs biomarker end points established in bacteria, rodent, fish and in vitro cell culture models. These include changes in gonad or reproductive pathology, alterations in reproductive capacity and in steroid receptor binding and changes in hormone titers, protein expression, and/or enzyme activity. The application of biomarkers to the investigation of EDC exposure in molluscs has followed a similar path. With the exception of the lack of steroid receptor binding, many of the measured end points following exposure to EDCs in molluscs are similar to those used as biomarkers in higher organisms (see Table 6.4).
Table 6.4 Effects of endocrine-disrupting compounds in molluscs
6.3.1 Reproductive Pathology
Imposex and the highly related condition of intersex serve as pathological end points that can be used as a biomarker of exposure to xenoandrogens. First reported by Blaber [82] and Smith [83] as masculinization of female neogastropod snails, the term “imposex” was used to describe the “superimposition of male sex organs onto females” in Nucella lapillus and Nassarius (Ilyanassa) obsoletus. It was more than a decade later that this condition was linked with tributyltin (TBT) pollution. Imposex and/or intersex have since been identified in more than 150 different species of molluscs (an example of imposex in Ilyanassa obsoleta is shown in Figure 6.4) [84,85]. Although imposex development has largely been reported in field and laboratory studies following organotin exposure, there have been reports of androgens and androgen-like compounds stimulating this morphological differentiation of male accessory sex organs (a penis and vas deferens) in female molluscs [29,80,86].
FIGURE 6.4 Reproductive tissues and secondary sex organs of imposex, male, and female Ilyanassa obsoleta. (AG = albumen gland; ECG = egg capsule gland; O = ovary; P = penis; PG = prostate gland; PO = posterior oviduct; SV = seminal vesicle; T = testis; VD = vas deferens.) Source: Photographs P. McClellan-Green.
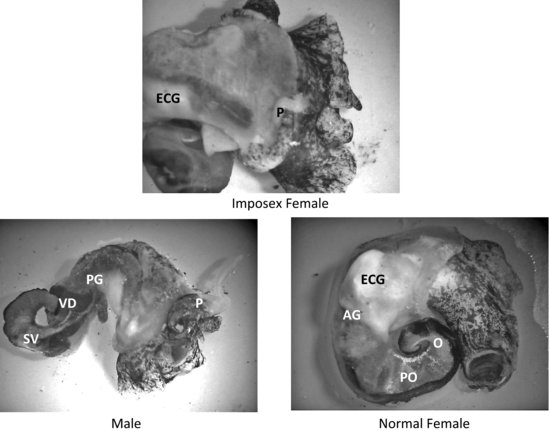
There is also evidence that estrogens and estrogen-like compounds influence the morphological development of molluscs. Oehlmann et al. [87] first demonstrated the production of “superfemales” in the freshwater snail Marisa cornuarietis and the marine prosobranchs Nucella lapillus and Hinia reticulata following exposure to the xenoestrogens bisphenol A (BPA) and octylphenol. Effects included formation of an additional vagina and enlargement of the accessory pallial sex gland. They also reported a reduction in penis length and prostate gland in male Nucella. In later studies, Andrew et al. [88] demonstrated the production of ovotestis and delayed spermatogenic development in the Sydney rock oyster Saccostrea glomerata exposed to 17α-ethynylestradiol and 4-nonylphenol (NP). Likewise, Lehmann et al. [89] noted increased gondal atrophy in freshwater clams, Corbicula fluminea, exposed to a mixture of estrogenic polychlorinated biphenyls.
The biological/physiological mechanisms responsible for endocrine disruption in molluscs are still unknown. One controversial area centers on the mechanisms responsible for the development of imposex/intersex in gastropods resulting from organotin exposure. The abundance of evidence involving androgenic compounds and some estrogenic compounds tends to indicate the involvement of steroid receptors. However, because ARs have not been identified in gastropods, multiple theories abound. Initially, imposex was thought to occur as a result of increases in testosterone levels within the tissues of organotin-exposed females. This was largely based on the inhibition of cytochrome P450, specifically P450 aromatase, by organotins. Other theories for the production of imposex center on changes in regulation of the RXR [71,76], production of a penis morphogenic factor [29,30], and inhibition of fatty acid esterification of testosterone [90].
The controversy surrounding the feminization of gastropods has also spawned a lively debate. Oehlmann et al. [87] and Duft et al. [91] reported the superfeminization of prosobranch gastropods following BPA exposure. This superfeminization, in Marisa cornuarietis and Potamopyrgus antipodarum respectively, included the formation of additional female reproductive organs, such as accessory sex glands, and malformations of the pallial oviduct as well as increases in egg production. Oehlmann et al. [92] and Benstead [93] also noted increases in reproduction in Viviparus viviparous, Bithynia tentaculata, and Planorbarius corneus following estradiol exposure. The endocrine-regulated mechanism responsible for these reproductive increases and morphological changes are unknown; however, Oehlmann et al. [92] did report the presence of steroid-specific binding by cytosolic proteins portending the presence of sex hormone receptors. Their research also identified temperature-specific requirements and the use of organisms within a specific annual (morphological) life stage to elicit these effects, thus indicating a possible role for seasonal biorhythms or seasonally regulated gene expression.
6.3.2 Reproductive Capacity
Molluscs that exhibit altered gonad pathology following exposure to endocrine disruptors usually experience a change in spawning activity, gametogenesis, or embryo production. Many environmental contaminants, such as BPA, hexachlorobenzene, triclocarban, and octylphenol, educe an estrogenic response similar to the steroids 17α-ethynylestradiol and 17β-estradiol resulting in increased spawning and embryo production [87,91–94]. (Note: Exposure of Placopecten magellanicus to testosterone resulted in increased spawning in males [60].) The physiological actions of BPA remain controversial, as Forbes et al. [95] reported no effect by BPA on Marisa reproduction. However, this group used a slightly different exposure regime and did not examine the animals for morphological changes in their reproductive organs as described by Oehlmann et al. [87].
The mechanism responsible for increased reproductive output remains a mystery. As discussed previously, binding of steroids or steroid mimics to hormone receptors has not been demonstrated in gastropods (with the exception of the Oehlmann et al. [92] study). Yet exposure of many different types of molluscs to either steroids or xenosteroids influences receptor-mediated outcomes. Rather than interacting directly with the receptor, it is possible that these compounds amplify the binding affinity of naturally occurring steroids to their receptor, thus increasing transcriptional activity and subsequent reproductive output. An example of this process was observed in laboratory studies using triclocarban, an antimicrobial agent added to cosmetics and personal care products. Triclocarban does not interact with steroid hormone receptors but was shown to increase transcriptional activity of steroid sex hormones for both the estrogen and ARs [96,97]. In these studies, the investigators showed a dose-dependent increase in androgen-mediated transcriptional activity following administration of triclocarban to testosterone-exposed PCDNA6-human AR transfected human embryonic kidney 293 cells. Giudice and Young [94] provided evidence for a similar process occurring in molluscs. They exposed the freshwater mudsnail Potamopyrgus antipodarum to increasing concentrations of triclocarban for up to eight weeks. A significant increase in the total number of embryos produced was noted for both unshelled (younger) and shelled (older) embryos. This adds further support to the concept of altered steroid hormone function in molluscs.
6.3.3 Protein Expression and/or Enzyme Activity
Several biomarkers adapted from use in higher species can be employed in the analysis of EDCs in molluscs. One of these, determination of vitellogenin (VTG) protein or mRNA induction, represents a well-accepted biomarker for the estrogenic effects of environmental contaminants [98–100] and is a good candidate for monitoring EDC effects in bivalves. Antibodies to VTG or VTG-like proteins have been developed for clams (Ruditapes philippinarum), mussels (Mya arenaria), oysters (Crassostrea gigas), and scallops (Platinopecten yessoensis) [101–105]. Other investigators have used the measurement of phosphoproteins, via the alkali-labile phosphate (ALP) method [106], in the absence of specific antibodies, as a proxy for VTG determination. VTG is a heavily phosphorylated protein that is induced by estrogen exposure and comprises a large component of plasma in vertebrates following the onset of vitellogenesis. Synthesis of VTG or VTG-like proteins in bivalves occurs via heterosynthetic and autosynthetic pathways within the gonads, but the mechanisms responsible for production of these proteins are still unknown.
As mentioned previously, estrogen levels vary throughout the spawning cycle, and exogenous administration of E2 or other estrogenic compounds increases the development of gametes in many bivalves [107]. This has been demonstrated multiple times with many species using both individual steroids as well as environmental mixtures (see Table 6.4 and references therein). Osada et al. [63] theorized that a neuroendocrine factor along with estrogen is needed for gamete formation. They partially isolated a vitellogenesis-promoting factor (<10,000 Da) from the cerebral and pedal ganglion of the scallop Patinopecten yessoensis. This promoting factor, when given in conjunction with E2, resulted in the accumulation of VTG in the ovarian tissues. The presence of this promoting factor could explain some of the controversial results observed in mussels. For example, injection of E2 into the oyster Crassostrea gigas, the soft shell clam Mya arenaria, and the freshwater mussel Elliptio complanata results in increased expression of VTG mRNA or ALP levels in their gonads [59,102,108,109]. These results contrast with the lack of estrogen-mediated VTG induction in the blue mussel Mytilus edulis reported by Riffeser and Hock [110].
Two research laboratories recently examined this species difference in VTG induction. Matozzo and Marin [99] exposed the clams Tapes philippinarum to increasing concentrations of 17β-estradiol for seven or 14 days. When the clams were exposed for seven days during their resting phase, a significant increase in ALP was observed in the hemolymph at all doses in females. No increase was observed in the digestive gland. There were no discernible trends in the 14-day exposure. When the clams were exposed during their pre-spawning phase, a decrease in female hemolymph VTG was observed at seven days and an increase at 14 days. Digestive gland VTG increased at 14 days for both sexes at all doses. These results are difficult to understand; indeed, the authors admit there is no clear explanation—except for the need to synchronize the reproductive status of test organisms. In a similar study, Ciocan et al. [111] exposed Mytilus edulis to estradiol, ethynylestradiol, and estradiol benzoate at different periods of their yearly reproductive cycle. A significant increase was noted in both VTG levels and ER2 mRNA levels when the mussels were exposed during an early stage of gametogenesis. No changes in VTG or mRNA expression were observed if the mussels were exposed to the compounds once sexually mature.
VTG-like proteins have also been measured in gastropods. Gagnaire et al. [112] developed methods for measuring VTG-like proteins in three different species of gastropods, the asexual species Potamopyrgus antipodarum, the hermaphrodite Valvata piscinalis, and the sexually reproductive Lithoglyphus naticoides. Two of these, Potamopyrgus antipodarum and Valvata piscinali, were later exposed to increasing concentrations of BPA, octylphenol, or TBT. Potamopyrgus showed an increase in VTG-like protein after 14 days exposure to 100 μg/L BPA and 1 μg/L octylphenol. A decrease of VTG-like protein was observed in this species following exposure to 5± ng/L TBT or high concentration of octylphenol (100 μg/L). In Valvata, a decrease in VTG-like proteins was observed at 14 days, but by 28 days a significant increase was observed in VTG-like electrophoretic proteins. Unfortunately, this study did not examine the effects of EDC exposure on VTG-like proteins in the sexually reproductive Lithoglyphus. Thus, it appears that the timing of exposure is critical when examining biomarkers of exposure or response.
6.3.4 Behavior
Recently, many vertebrate toxicologists have begun to use behavioral end points as biomarkers of endocrine disruption [113,114]. This includes predator avoidance in fishes, courting in songbirds, and mounting behavior in rodents. Behavioral changes are also relevant when measuring ecotoxicological end points in invertebrates. For instance, an absence of antipredator response was reported in freshwater snails, Physella columbiana, collected from lakes contaminated with heavy metals [115]. Likewise, behavioral changes have been reported for other species of snails exposed to heavy metals [116,117]. These examples tend to indicate a change in the perception of a defined chemical cue by the exposed animal; however, studies relating exposure to EDCs and behavior changes have been limited.
A classic example of behavioral changes following exposure to EDCs include the study by Straw and Rittschof [118] in which they observed a lack of reproductive behavioral dimorphism in mudsnails collected from harbor sites having high TBT levels. Other EDC-related behavioral changes observed in gastropods include alteration of locomotor activity following exposure to polycyclic aromatic hydrocarbons [119–121] and feeding and reproductive changes resulting from triazine herbicide exposure [122]. For example, in a recent study mudsnails, Ilyanassa obsoleta, were acutely exposed to Irgarol 1051 (0.375–1.5 mg/L) for 96 hours. These animals experienced a significant decrease in their chemosensory response to food and predator cues indicating a possible neuroendocrine or pheromone inhibition [123].
It is therefore evident that biomarkers of EDC exposure are useful in molluscs, but more information is needed regarding the mechanisms of action of both natural hormones and xenosteroids.
6.4 CURRENT TEST METHODS USING MOLLUSCS
No standardized methods for measuring endocrine disruption in molluscs are currently available [124]. Tests included in the EPA Tier 1 Battery (see Table 6.1
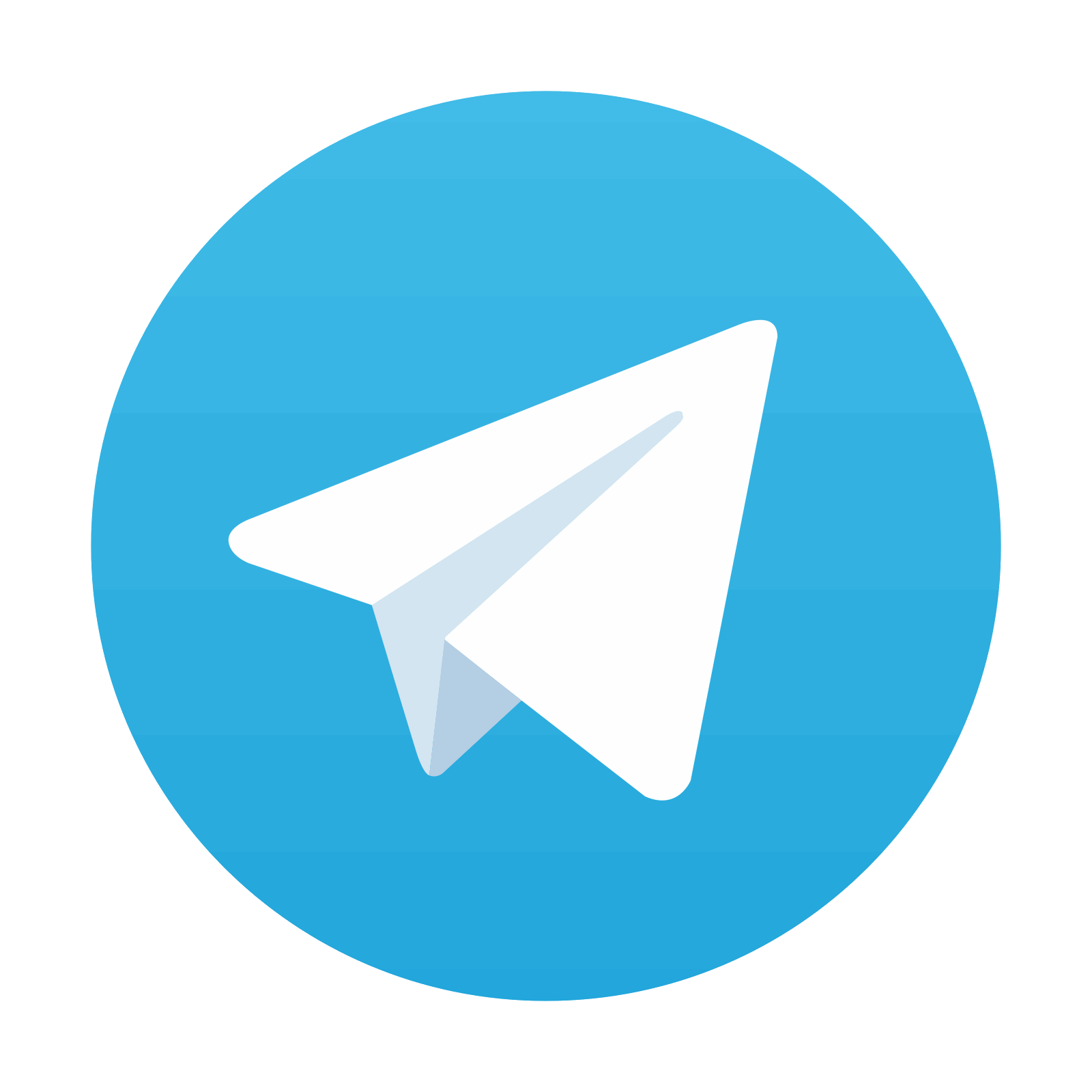
Stay updated, free articles. Join our Telegram channel

Full access? Get Clinical Tree
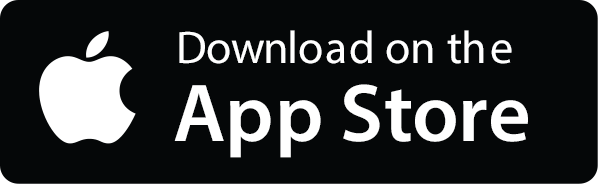
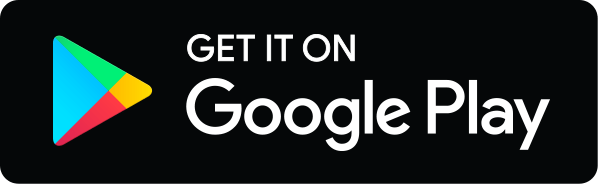