Lifestyle
Optimize glycemic control in people with diabetes
Weight loss if overweight or obese
Physical exercise
Nonsmoking
Healthy diet
Plant sterol-supplemented foods
Predominantly LDL-lowering treatments
HMG-CoA reductase inhibitors
e.g., rosuvastatin, lovastatin, pravastatin, fluvastatin, simvastatin
Cholesterol absorption inhibitors
Bile acid-binding resins
e.g., cholestyramine, colestipol
Ezetimibe
LDL (and Lp(a)) apheresis
Predominantly triglyceride-lowering agents
Fibrates
e.g., fenofibrate, gemfibrozil
Nicotinic acid
Fish oils
Combination tablets
Simvastatin and ezetimibe
Pravastatin and nicotinic acid
Clinical benefits of lipid-targeting drugs may be related to both improvements in the lipid profile and also an array of potential pleiotropic effects [26–28], summarized in Table 23.2. Additional as yet unknown pleiotropic effects may also exist. The relative importance and dose relatedness of the pleiotropic effects of these drug classes are not fully elucidated in either diabetic or nondiabetic subjects. Some of the novel agents in development are targeting lipid levels and also have pleiotropic effect(s), and it remains to be seen if this approach provides additional vasoprotection. Greater knowledge of the beneficial pleiotropic effects of lipid drugs on hemostasis, inflammation, vasoreactivity, and on the cardiovascular, retinal, renal, and neural systems and their mechanisms may facilitate the development of new drug classes to ameliorate the chronic complications of diabetes.
Table 23.2
Potential pleiotropic effects of lipid drugs
Improve endothelial function |
e.g., statins and fibrates induce NOS |
Stabilize atherosclerotic plaques |
Antioxidant |
Anti-inflammatory |
Antithrombotic effects |
Antiplatelet effects |
Angiogenesis related |
e.g., statins may have pro-angiogenic effects, and fibrates are anti-angiogenic in the eye |
Cell signaling effects |
e.g., fibrates activate PPARα and AMP kinase and suppress Wnt signaling pathways |
Neuroprotective effects (e.g., of fibrates) |
Protection against telomere shortening (e.g., of statins) |
Skin protective effects (of fibrates via keratinocyte differentiation and epidermal effects) |
While many people with type 2 diabetes and with type 1 diabetes are prescribed lipid drugs, in particular a statin, some have contraindications to their use, for example, severe renal or liver disease, some do not meet the desired lipid goal even when at maximum tolerated drug dose, some are intolerant of the prescribed medication, and some are reluctant to or cannot afford to take them. Even in this era of evidence-based medicine, not all available lipid drugs and lipid drug combinations have been tested in major vascular end-point clinical trials in people with diabetes, especially those with type 1 diabetes and younger type 2 diabetes patients. The latter group, which is becoming more common [29], often includes obese youth from high-risk ethnic groups [29–31] and is of major concern, as they often have dyslipidemia and are at particularly high risk of vascular disease [31, 32]. Indeed their vascular complication rates are higher than that of young people with type 1 diabetes [32]. As we also now recognize that atheroma begins in childhood, particularly in those on a western diet, and in those who are obese, dyslipidemic, or dysglycemic [33, 34], the safety and efficacy of lipoprotein treatments in youth must be assessed.
In this chapter we will discuss emerging treatments closely related to approved lipid drugs and lipid treatments in development, relating them to their primary lipid target, such as LDL lowering, triglyceride lowering, and HDL elevating. These categories are summarized in Table 23.3. Some of the agents are in or have been in clinical trials, while others are in earlier phases of development.
Table 23.3
Emerging lipid therapeutic agents
Combination lipid drugs |
New “statins” |
New “fibrates” |
New fish oil-like agents |
New LDL-lowering agents |
Inhibitors of proprotein convertase subtilin kexin 9 (PCSK9) |
Thyromimetic agents |
Inhibitors of ApoB-containing lipoproteins: antisense oligonucleotides and siRNAs inhibitors of (early and late) glycation |
HDL-elevating drugs |
ApoA1 mimetics |
Modulators of lipoprotein-related enzymes, e.g., CETP inhibitors |
Triglyceride-lowering drugs |
Modulators of lipoprotein-related enzymes, e.g., inhibitors of CETP, DGAT2, ACAT, and MTP |
ApoC-III ASO |
Miscellaneous |
Inhibitors of lipoprotein glycation and AGE modification |
Inhibitors of lipoprotein immune complex formation |
Inhibitors of lipoprotein and matrix interactions |
Inhibitors of foam cell formation |
Antioxidants |
Anti-inflammatory agents |
Strategies Aimed at Lowering LDL
New Statins. Since the advent of statins over 20 years ago, this class of drugs has been the mainstay of lipid-lowering therapy. While early studies with relatively weaker statins showed modest effects, more recent studies using powerful statins in very high-risk individuals indicate that a reduction of 30–40 % in relative risk might be expected from statin monotherapy [1–12]. With the statins in use today, doubling their daily dose only results in only about 6 % further reduction in LDL-C levels and a greater risk of side effects such as myalgia/myositis [35]. The development and trials of even more potent statins are in progress. One such novel statin, which is licensed for use in some countries is pitavastatin [36–38]. As shown by large phases 3 and 4 studies, pitavastatin can lower LDL-C levels by 40–65 % and lower triglyceride levels by about 20 % and has more marked HDL-C-elevating effects (up to 14 %) than current commonly used statins [36–38]. In vitro studies demonstrate potent stimulation of ApoA1 production by hepatocyte-like cells [39]. Pitavastatin has minimal metabolism via cytochrome P450 (CYP) enzymes, which may benefit patients requiring multiple drugs, as is common in people with type 2 diabetes [40]. About 10 % of subjects experienced adverse events of a similar nature to that of other statins. In a large (n ~ 20,000) Japanese long-term prospective post-marketing surveillance LIVALO Effectiveness and Safety (LIVES) study, 2-year pitavastatin use was associated with 29 % lower LDL-C levels and 5.9 % higher HDL-C levels, with a 24.6 % HDL-C rise in those with low (<40 mg/dL) HDL-C levels at baseline [41]. In a LIVES substudy, HDL-C levels rose in subjects changing from other statins to pitavastatin [42]. This potent statin has shown longer-term safety and efficacy in acute myocardial infarction patients, including diabetes subjects [43], can improve insulin resistance, and has shown no deleterious effects on glycemia in people with diabetes [44]. As shown by the Japanese Assessment of Pitavastatin and Atorvastatin in Acute Coronary Syndrome (JAPAN-ACS) study [45] and other longitudinal trials with intermediate coronary and carotid vascular end points [46–48], pitavastatin significantly improves atheroma volume and quality.
Interestingly in animal studies, including diabetic rodents [49, 50], and in some human studies, this new statin improves renal function in chronic renal disease subjects [51], including lowering albuminuria in type 2 diabetic patients [52]. The results of long-term studies with mortality and clinical cardiovascular, renal, and retinal end points in diabetes are awaited with interest.
Another statin in development is NCX6560 (NicOx, Sophia-Antipolis, France), which combines a statin with a nitric oxide (NO) donor to enhance vasodilatory effects [53]. As diabetes is associated with impaired vascular endothelial function and reduced NO bioavailability [54], this is of particular interest in diabetes.
Combination Therapies. As greater LDL lowering is associated with greater risk reduction [1, 2] and statin monotherapy alone leaves many patients with LDL-C levels above target, in which case combination therapy is often recommended. As well as increased efficacy in improving the lipid profile and reducing vascular events combination tablets are often helpful in increasing adherence and reducing drug costs to individuals.
Statins can be used in combination with bile acid-binding resins to achieve additional ≈10 % LDL-C lowering, but gastrointestinal side effects are common. Resins bind bile acids in the gut and induce secondary upregulation of hepatic LDL receptors, thus lowering LDL-C levels. Resins do not significantly alter HDL-C levels and can elevate serum triglyceride levels (via effects on the liver X receptor (LXR) [55, 56]. Bile acid sequestrants can also lower blood glucose levels via a farnesoid-X-receptor action, and colesevelam is approved for both lipid- and glucose-improving effects [57–59].
A better tolerated (than resins) combination is that of a statin with the cholesterol absorption inhibitor [60], ezetimibe, which acts by inhibiting the intestinal cholesterol transporter, NPC1-L1 and usually lowers LDL-C by an additional 20 % [60–62]. There are ongoing trials of statins plus ezetimibe, with the recently reported SHARP study finding benefit of 20 mg simvastatin plus 10 mg ezetimibe vs. placebo in a RCT of 9,270 chronic kidney disease patients, including approximately 20 % with diabetes, with a 17 % reduction in risk of a first major atherosclerotic event over a 4.9-year follow-up [63].
The combination of a statin with fish oils can address the combined dyslipidemia that is common in type 2 diabetes. In the COMBination of Omega-3 preparation with Simvastatin (COMBOS) study, 4 g daily prescription omega-3 fatty acids or placebo was added to simvastatin 40 mg daily in 254 patients with hypertriglyceridemia. Relative to the placebo group, the fish oil group demonstrated significantly lower triglycerides (29.5 vs. 6.3 %) and higher HDL-C (3.4 vs. −1.2 %), which was well tolerated and sustained for 2 years [64–66]. There are no clinical end-point trials in diabetes yet.
The effects of most of these combination therapies have not been tested in major clinical end-point outcome trials with large numbers of people with diabetes. In the future additional combination therapies may also become available. Diabetes-specific trials or adequately sized subgroups of patients with diabetes and with macrovascular and microvascular end points are desirable.
Preprotein convertase subtilin kexin 9 (PCSK-9) is a secreted protein that degrades the LDL receptor in hepatocytes and increases LDL-C levels [67, 68]. Genetic mutations in PCSK-9 cause FH, and loss of function mutations of this protein is associated with modest reductions in LDL-C levels, yet considerable protection from cardiovascular disease [69, 70]. The extent of protection is much greater than that seen with comparable degrees of LDL lowering in clinical trials [71, 72]. In human populations, PCSK-9 levels in blood are usually correlated with BMI and lipid levels (triglycerides, total and LDL-C) and are affected by sex hormones and growth hormone [73]. Some of the LDL-C-lowering effects of fibrates are thought to be mediated by PCSK-9 [74]. In several prospective studies in statin-treated type 2 diabetic patients, fenofibrate effects on PCSK-9 levels are divergent, with some finding lowering effects of fenofibrate [75] and others finding elevated PCSK-9 levels [76]. PCSK-9 is upregulated by statins [72, 77], thus potentially limiting the full potential of statins to lower LDL-C levels. Ezetimibe intervention is also associated with increased circulating PCSK-9 levels [77]. Thus, the combination of a statin and/or ezetimibe with a PSCK-9 inhibitor might prove to be particularly effective at lowering LDL-C levels and vascular events. The challenge is how best to inhibit PSCK9 in vivo. At present, the most promising response appears to be by the administration of antibodies, although these would need to be administered parenterally. Human monoclonal antibodies to PCSK-9 (e.g., RGEN-727 (Regeneron, Tarrytown, NY)) given parenterally have lowered LDL-C levels in statin-treated heterozygous FH patients and in nonfamilial hyperlipidemic subjects [78].
Thyromimetic Agents. D-Thyroxine was evaluated in the Coronary Drug Project many years ago but resulted in cardiac arrhythmias and in increased mortality [79, 80], an effect that has been attributed to contamination of the medications used with small amounts of L-thyroxine. Thyroid hormone administration can lower LDL-C levels by increasing hepatic LDL receptor expression via activation of thyroid hormone receptor, but has adverse effects on the heart and bone via activation of thyroid hormone receptor [81, 82]. These effects are highly undesirable in people with diabetes who are at higher risk of osteopenia and osteoporosis [83] and for those with type 1 diabetes who are also at increased risk of (autoimmune) thyroid disease [84]. With the development of selective activators of thyroid hormone receptor-β, there has been renewed interest in the use of selective thyromimetics for lowering LDL levels without having an adverse effect on the heart and bones. Selective thyroid hormone receptor-β agonists appear to deplete hepatocytes of cholesterol in addition to activating LDL receptors directly [85, 86], so they may have a somewhat different mechanism of action to statins. Unlike statins, thyromimetics can also lower Lp(a) levels [87]. They have been shown to reduce atherosclerosis in an animal model [88] and are beginning to be tested for their lipid-lowering effects in human subjects. Studies in people with diabetes will be of interest.
Antisense Oligonucleotides (ASOs) and Small Interfering RNA
ASO and siRNA constructs that target ApoB are in development and may, with time, prove useful in the management of certain patients with increased LDL levels and/or some forms of hypertriglyceridemia. ASOs are single-stranded RNA that bind to mRNA, whereas siRNAs are short duplexes of RNA that contain a sequence identical to that in the target RNA. Both lead to destruction of the mRNA target and inhibition of protein synthesis [88]. A downside of both is that they need to be administered by injection, which to date has been associated with marked injections site reactions and also with hepatic steatosis, so they are likely to only be useful for very specific patients in whom adequate LDL lowering cannot be readily achieved by other means. The most well developed of these agents is the ASO Mipomersen (Isis, Carlsbad, CA) which targets ApoB and is in phase 3 trials in FH [89]. An additional potential advantage of these agents is that they may also reduce Lp(a) levels, although there currently is no information as to whether or not that would be beneficial for cardiovascular disease prevention [87].
Other Drugs. Several other drug classes that lower LDL-C levels by modulating lipoprotein metabolism are being investigated but have been limited by adverse effects. Squalene synthase inhibitors, such as lapaquistat (Takeda, Osaka, Japan), act in the endoplasmic reticulum to limit synthesis of cholesterol, but not of geranyl pyrophosphate-derived compounds (such as coenzyme Q10). LDL-C reductions were relatively small (about 15 %), and there were hepatic side effects [90]. Similarly microsomal transfer protein (MTP) inhibitors, such as lomitapide (Aegerion Pharmaceuticals, Cambridge, MA) which lower LDL-C by up to 80 % and triglycerides by 40 %, also caused hepatic dysfunction (fatty liver and elevated transaminases) [91, 92]. Acyl–cholesterol acyltransferase (ACAT) inhibitors which block lipoprotein synthesis and foam cell formation have reached clinical trials [93–95] but did not reduce coronary atheroma burden in IVUS studies [93, 94] or slow carotid IMT increase in familial hypercholesterolemia (FH) patients [95].
Addressing Residual Risk Beyond Statin-Mediated LDL Lowering
Despite the success with statin therapy in all types of high-risk individuals, a considerable residual risk nonetheless exists in many individuals even after appropriate statin therapy. Some of this residual might be reduced by even more aggressive LDL-C lowering, which potentially could be achieved by using even more powerful statins, by the use of the combination therapies, or by using some of the newer strategies that are undergoing clinical testing and are not yet available for routine use (described above).
Much of the residual cardiovascular risk in both the nondiabetic and diabetic population appears to be related to low HDL cholesterol (HDL-C) and hypertriglyceridemia [97, 98], which often is associated with the metabolic syndrome and with type 2 diabetes [99]. Much less data is available regarding specific approaches to lower triglycerides and increase HDL-C levels than is available for LDL-C lowering. Also, much less is known concerning mechanism of atherosclerosis with these lipoproteins than for LDL. For example, although triglyceride-rich lipoproteins and their remnants can bind to and be retained by vascular proteoglycans [100–103], triglycerides do not accumulate to any major extent in atherosclerotic lesions, and it is not clear whether triglyceride-rich lipoproteins are directly atherogenic, or whether their effect is indirect, or both [104–106]. Hypertriglyceridemia is often accompanied by low HDL levels, an accumulation of remnant lipoproteins, and the presence of small, dense LDL particles, which appear to be particularly atherogenic [107, 108]. HDL is believed to exert its atheroprotective effect largely by facilitating reverse cholesterol transport. However, HDL also has anti-inflammatory, antioxidant, and antithrombotic properties and vasodilatory effects [109–113], all of which may play a role in reducing atherosclerosis risk and potentially also diabetic retinopathy and nephropathy [114].
HDL Raising and TG Lowering as Targets
Approaches to Lowering Triglycerides and Raising HDL
High triglycerides and low HDL levels are amenable to therapeutic approaches other than statins. Although statins in general result in modest elevations of HDL-C and modest reductions in triglycerides, other drugs more specifically target HDL and triglycerides. The two most widely used classes of drugs that target both hypertriglyceridemia and low HDL-C levels are fibrates and niacin [115–120]. However, clinical trials with fibrates, discussed in other chapters in this book, have yielded mixed and sometimes disappointing results with regard to cardiovascular disease [13–15, 17]. Fibrates and specifically fenofibrate have shown cardiovascular benefit in type 2 diabetes patients with high triglyceride and low HDL-C levels [15–17, 120] and microvascular benefit [18–22]. As yet there are no known clinical end-point trials of fibrates in type 1 diabetes, and there is also a paucity of data concerning the vascular effects of niacin in diabetes. New versions of both fibrates and niacin are undergoing investigation.
Fibrates and Other PPAR Agonists. The PPARα agonists such as fenofibrate lower VLDL and increase HDL by increasing expression of lipoprotein lipase and apoA1 and apoA2 and also have pleiotropic effects including related to blood clotting and inflammation (Table 23.2). Fibrates have shown clinical benefit in RCTs in type 2 diabetes patients [15–22], but many of these favorable responses do not correlate strongly with the changes in lipid levels [18–22]. While fenofibrate was well tolerated in the large FIELD and ACCORD-Lipid studies [15–22] and is used in clinical practice for hypertriglyceridemia, more specific PPARα agonists have not progressed due to side effects such as muscle toxicity. Agonists to other PPAR ligands may also be of interest in the management of lipids in diabetes.
PPARγ agonists (such as the thiazolidinediones) also lower triglycerides and increase HDL as do PPARα agonists, and they also improve glycemia, lowering HbA1c levels up to 1 % [121]. While they reached clinical practice, they caused excessive fluid retention and increased the risk of bone fractures and in some cases (rosiglitazone) of myocardial infarction [122] and concerns about bladder cancer (pioglitazone) [123]. In clinical trials the combined PPARα and PPARγ agonists such as muraglitazar and ragaglitazar also had serious adverse effects related to bladder cancer and excess cardiovascular events [124, 125]. Of this class aleglitazar, which improves the lipid profile and HbA1c levels in type 2 diabetes patients, is still undergoing assessment in clinical trials. The phase 2 SYNCHRONY trial demonstrated adverse effects such as fluid retention with high-dose (600 μg) aleglitazar [126], and using this dose, the SESTA R trial demonstrated a reversible 19 % reduction in eGFR levels in type 2 diabetes patients [127]. The ALECARDIO phase 3 study of cardiovascular outcomes in 6,000 type 2 diabetes patients with acute coronary syndromes is in progress (as of 2012) [128].
PPARγ and PPARδ co-agonists and PPARα and PPARγ co-agonist drugs are also undergoing testing [129].
Niacin–Related. Like the fibrates, nicotinic acid (niacin)-related drugs also effectively lower triglyceride levels [130, 131], whereas niacin is more effective in raising HDL-C levels than fibrates [132, 133]. As well as reducing LDL-C levels to a modest extent, niacin is the most effective drug currently clinically available for reducing Lp(a) levels [134]. In the old Coronary Drug Project, niacin monotherapy was associated with risk reduction [135] and reduced mortality several years after the conclusion of the study [136]. Niacin has been available for many years, but its widespread use has been limited by nuisance side effects, particularly flushing, which is believed to be due to niacin-induced production of prostaglandin D2 and other prostaglandins in skin immune cells [137], by hepatotoxicity and impaired glucose tolerance [138, 139]. Since the advent of relatively effective slow-release forms of niacin and the approval in some countries of niacin combined with a prostaglandin D2 type 1 receptor inhibitor, laropiprant, that reduces flushing by up to 80 % [140], there has been a renewed interest in this old drug. In a 64-week study of 949 hyperlipidemic subjects on simvastatin and ezetimibe, the addition of slow-release niacin increased blood glucose levels and new-onset diabetes which often improved or remitted without specific treatment [141]. Although use of niacin plus a statin led to lesion regression and improved clinical outcomes in small angiographic studies [142], the large AIM-HIGH study of 3,414 patients with low HDL-C levels on intensive statin therapy, including about one third with type 1 or type 2 diabetes, the clinical cardiovascular benefit of added slow-release niacin (1,500–2,000 μg daily) was tested, but the trial was stopped after 3 years due to lack of clinical efficacy [143]. The HPS2/THRIVE study is also testing the clinical vascular effects of niacin/laropiprant [144]. Other non-flushing niacin variants have been developed and at least one has reached clinical trial phase.
Newer Targets for Increasing HDL
Several new targets to raise HDL are being tested. These include the CETP inhibitors and apoA mimetic peptides.
CETP Inhibitors
The initial enthusiasm for CETP inhibitors has been dampened considerably by the negative experience with torcetrapib, trials of which led to a predicted increase in blood pressure, an unpredicted increase in cardiovascular events, cancer deaths, and deaths due to infections [145, 146]. These events occurred despite very favorable changes in plasma lipid and lipoproteins, i.e., a marked increase (up to 150 %) in HDL-C levels, a respectable 25 % decrease in LDL-C levels, and a highly favorable change in the LDL-C/HDL-C ratio [147]. In lipoprotein kinetic studies, torcetrapib did not improve cholesterol efflux to fecal sterols [148]. Therefore, the relationship between HDL and cardiovascular disease appears to be a lot more complex than is apparent from the epidemiology.
It has been suggested that the increase in cardiovascular disease was due to an off-target effect of torcetrapib to increase blood pressure by increasing aldosterone levels [149, 150]. However, concern has been raised that the adverse effect of these inhibitors on cardiovascular outcomes might be related to their ability to block part of the reverse cholesterol pathway [145]. Moreover, the increase in cancer and infective deaths raises concern that CETP inhibitors might be interfering with HDL’s role in innate immunity. Other CETP inhibitors that do not have this effect on the renin-angiotensin system are currently being tested in large outcome studies.
Dalcetrapib, which increases HDL-C by 33 %, including levels of pre-beta HDL does increase fecal sterol excretion and does not cause hypertension [151]. In intermediate end-point studies, dalcetrapib did not improve endothelial function [152] or plaque composition (DAL-PLAQUE study) [153], and recently (May 2012) due to lack of efficacy, but not safety concerns, the company ceased its clinical end-point studies (DAL-ACUTE and DAL-OUTCOMES).
Another CETP inhibitor in clinical trial phase is anacetrapib. Anacetrapib is structurally closer to torcetrapib than to dalcetrapib and unlike dalcetrapib decreases pre-beta HDL-1. In the 3-month DEFINE study of coronary heart disease (CHD) or CHD-risk equivalents on a statin background, 100 mg anacetrapib increased HDL levels by 138 %, decreased LDL by 40 %, and also decreased Lp(a) levels, with no effects on blood pressure or the renin-angiotensin-aldosterone system [154]. Anacetrapib is, as of 2012, in a large 4-year atorvastatin background placebo-controlled clinical trial (n ≈ 30,0000 subjects, including people with diabetes) (REVEAL) with a primary end point of cardiac death, myocardial infarction, or coronary revascularization [155].
If they too have adverse effects similar to what was observed with torcetrapib or lack clinical efficacy as with dalcetrapib, this might severely dampen enthusiasm for developing drugs that increase HDL levels. The torcetrapib experience also points out the need to differentiate HDL levels from HDL functionality and points out the need to develop relatively simple, widely applicable, and reproducible assays of HDL function that can be correlated with outcomes in clinical trials.
ApoA-I Mimetic Agents
Many of the beneficial effects of HDL, particularly its role in reverse cholesterol transport, are likely to be largely due to the major apolipoprotein in HDL, apoA-I. Since apoA-I has to be administered by intravenous injection, attempts have been made to create small peptides that mimic the effect of the intact apolipoprotein. Several small apoA-I mimetic agents have been shown to have anti-inflammatory and antioxidant effects in vitro [156–158] and anti-atherogenic effects in animal studies [159, 160]. More recently they have begun to be tested in human subjects.
Many of the beneficial effects of apoA-I have been attributed to the ability of the class A amphipathic helices of this apolipoprotein to bind oxidized lipids, particularly oxidized phospholipids [161]. These mimetic peptides were designed to contain class A amphipathic helices and have been shown to have high affinity for oxidized phospholipids [161]. They are believed to work by tightly binding these toxic lipids, thereby preventing them from having adverse effects on vascular cells. While intact apoA-I has to be administered by intravenous infusion and has a relatively short half-life, an advantage of some of these smaller apoA-I mimetic peptides is that they can be administered orally. Future studies will determine whether they will have a role to play in the prevention and treatment of atherosclerosis, including in people with diabetes.
An ideal approach to raising HDL would be to stimulate the synthesis of endogenous apoA-I in a more potent manner than fibrates. Indeed, initial testing on one such small molecule that stimulates apoA-I synthesis has been reported [162]. Further studies using this approach are awaited with interest.
Apheresis. Both plasmapheresis and LDL apheresis, which involve weekly or biweekly invasive and costly procedures, substantially lower both LDL-C and Lp(a) levels with only slight HDL-C increases. Such treatments prolong life in homozygous FH patients and are now used in children and pregnant women with FH and increasingly for heterozygous FH subjects and other patients with aggressive coronary artery disease on or intolerant of maximal lipid drug therapy [163]. Apheresis can also be used to selectively remove ApoA1-containing HDL particles, which can then be delipidated and reinfused. This has shown promise in reducing atheroma in nonhuman primates [164] and (IVUS determined) carotid plaque in acute coronary syndrome patients [165]. In the future these types of apheresis therapies may be of clinical benefit to a limited subset of people with diabetes.
Newer Targets for Triglyceride Lowering
Similar to the situation with increasing HDL levels by pharmacologic means, there are no data that definitely show that lowering triglyceride levels leads to a reduction in clinical events. As noted earlier, many of the fibrate trials have been handicapped by the fact that they were not performed in hypertriglyceridemic subjects. In the FIELD study, many of the vascular benefits, such as the retinopathy benefit [20], did not correlate with reduction in the standard lipid measures. Even if the ongoing niacin trials are positive, they are unlikely to show a definitive role for triglyceride lowering because of the multiple other effects of niacin. Nonetheless, there have been considerable efforts over the past several years to develop hypotriglyceridemic drugs that work by reducing hepatic triglyceride production. These include inhibitors of DGAT2, ACAT, and MTP, discussed above, which lower both VLDL and LDL levels. None have been very successful to date, with lipid accumulation in the liver, skin, and other tissues being the biggest problems with these agents.
Fish Oils
High doses of fish oils, which contain docosahexaenoic acid (DHA) and eicosapentaenoic acid (EPA), greater than that usually achievable by the regular consumption of oily fish meals, are effective at lowering triglyceride levels, usually by 10–30 %, in a dose-dependent manner. Other effects such as antiplatelet, antiarrhythmic, and anti-inflammatory effects may also contribute to clinical benefit. The wide range of fish oil capsules available in the supermarkets, health food stores, and in pharmacies (without a prescription) attest to the public interest in the use of fish oil supplements. In a recent analysis of 20 fish oil trials of at least 1 year between 1982 and 2012 including 68,860 subjects, there was no statistically significant association between all deaths, cardiac-related deaths, sudden deaths, heart attacks, and strokes among people taking the supplements [166].
In the GISSI-Prevenzione study (using DHA and EPA) [166] and the Japan EPA Lipid Intervention Study (JELIS) (using 1.8 g EPA daily) in statin-treated postmenopausal women and men [168], a prescribed fish oil supplement significantly reduced cardiovascular events in people, including subjects with diabetes, though did not specifically enrol hypertriglyceridemic subjects. Unfortunately in the recent ORIGIN trial of dysglycemic people with or at high risk of type 2 diabetes, 1 g of n-3 fatty acids daily for a median of 6.2 years reduced triglyceride levels (0.16 mmol/L) but did not demonstrate any clinical benefit re cardiovascular or arrhythmia-related death or cardiovascular event reduction [169].
AMR101, a purified fish oil EPA, is in human clinical trials. In the 12-week ANCHOR study (on a statin background) [170] and in the MARINE study [171] of high cardiovascular disease risk subjects with hypertriglyceridemia, 2–4 g a day of AMR101 was well tolerated and was associated with lower triglycerides (up to 21 %), VLDL-C, total cholesterol, ApoB, lipoprotein-associated phospholipase A(2), and CRP levels. LDL-C levels did not change significantly.
A large 6-year clinical end-point study of AMR101 in 8,000 patients with hypertriglyceridemia—the Reduction of Cardiovascular Events with EPA-Intervention Trial (REDUCE-IT)—is in progress.
Other drugs in development may target the recently identified omega-3 receptor which mediates lipid, anti-inflammatory, and insulin-sensitizing effects [172].
Apo C-III ASO
ApoC-III, an apolipoprotein that is transported on both VLDL and HDL, is an inhibitor of lipoprotein lipase [173, 174]. High circulating levels of apoC-III associate with hypertriglyceridemia and increased risk of cardiovascular disease. Recently it was shown that a null mutation in the apoC-III gene was associated with reduced triglyceride levels, a favorable lipid profile, and reduced risk of cardiovascular disease [175]. This has prompted a renewed search for approaches to lower apoC-III levels. One specific approach that might have promise in special circumstances is the use of an ASO directed specifically at apoC-III. Since ASOs need to be administered parenterally, this would obviously not have widespread use and is unlikely to be tested in a large outcomes study. Nonetheless, it demonstrates the potential use of a novel molecular approach based on molecular epidemiological findings.
Other Novel Agents
As well as quantitative changes, qualitative changes in lipoproteins are also implicated in the lipoprotein-mediated vascular complications of diabetes. Such changes, including lipoprotein glycation, oxidation, immune complex formation, and matrix-binding effects, have been discussed in other chapters in this book. Novel therapies that ameliorate these processes and vascular responses to native and modified lipoproteins could also contribute to reducing vascular damage and improving clinical outcomes in people with diabetes.
Drugs to Reduce Common Lipid Drug Side Effects
A common clinical problem in general practice and in specialist lipid clinics is that people with dyslipidemia are appropriately prescribed lipid-modifying drugs and they cannot tolerate them due to side effects. Myalgia related to statins is one such example. Sometimes these problems can be overcome by finding and (where possible) treating the alternate cause of the problematic symptom (such as a myopathy, hypothyroidism, low vitamin D levels, or drug interactions causing muscle-related problems [176]), by dosage reduction and slow up-titration of the lipid drug, or by changing to an alternate lipid drug. Other agents that are often suggested to reduce statin-related muscle symptoms, thought to be related to mitochondrial effects in muscle, include vitamin D, coenzyme Q10, and magnesium supplements [176, 177]. Randomized controlled placebo-controlled trials are desirable.
Nicotinic acid is another lipid drug whose side effects, including glucose intolerance and flushing, can limit its tolerability and clinical usefulness. Attempts to ameliorate these problems include the development of slow-release preparations and coadministration with aspirin and with laropiprant (discussed above). Similar challenges may also occur with the emerging treatments.
Conclusions
Dyslipoproteinemia contributes to the macrovascular and microvascular complications of diabetes. Improving lipid levels with oral agents such as statins and fibrates has improved cardiovascular outcomes in the general population and also in people with diabetes. Some lipid drugs can also protect the microvasculature in people with type 2 diabetes, but as yet this is an off-label effect (of fenofibrate). These important clinical benefits may relate to the direct effects of the improved lipid profile or to pleiotropic effects. Emerging lipid agents include more potent agents of the currently available lipid drug classes, combinations thereof (which may increase patient adherence and reduce cost), and more novel modulators of genes and enzymes related to lipoprotein metabolism, such as antisense therapeutics and enzyme inhibitors or activators. Drugs that safely alter lipoprotein interactions with matrix and cells or relevant cell signaling pathways may also prove useful, as could agents that mimic and amplify the pleiotropic effects of lipid drugs.
The development and testing of even more effective lipoprotein-targeted therapies will be a costly endeavor, but there are many millions of people with diabetes, and also people without diabetes, who may benefit by reduced rates of vascular damage and by improved quality and quantity of life. Avoidance of costly clinical events by primary or secondary prevention may also be cost-effective for those agencies that fund the treatments. This major and costly pharmaceutical and academic endeavor should also be complemented by clinical research to identify who requires such treatment(s), when they should take them, and for how long. Post-marketing surveillance and oversight by regulatory bodies will help ensure the safety of these new treatments as their use spreads beyond randomized controlled trials. Treatment algorithms, health economics analyses, and health-care policy should ensure translation of this evolving area of research into optimal clinical practice.
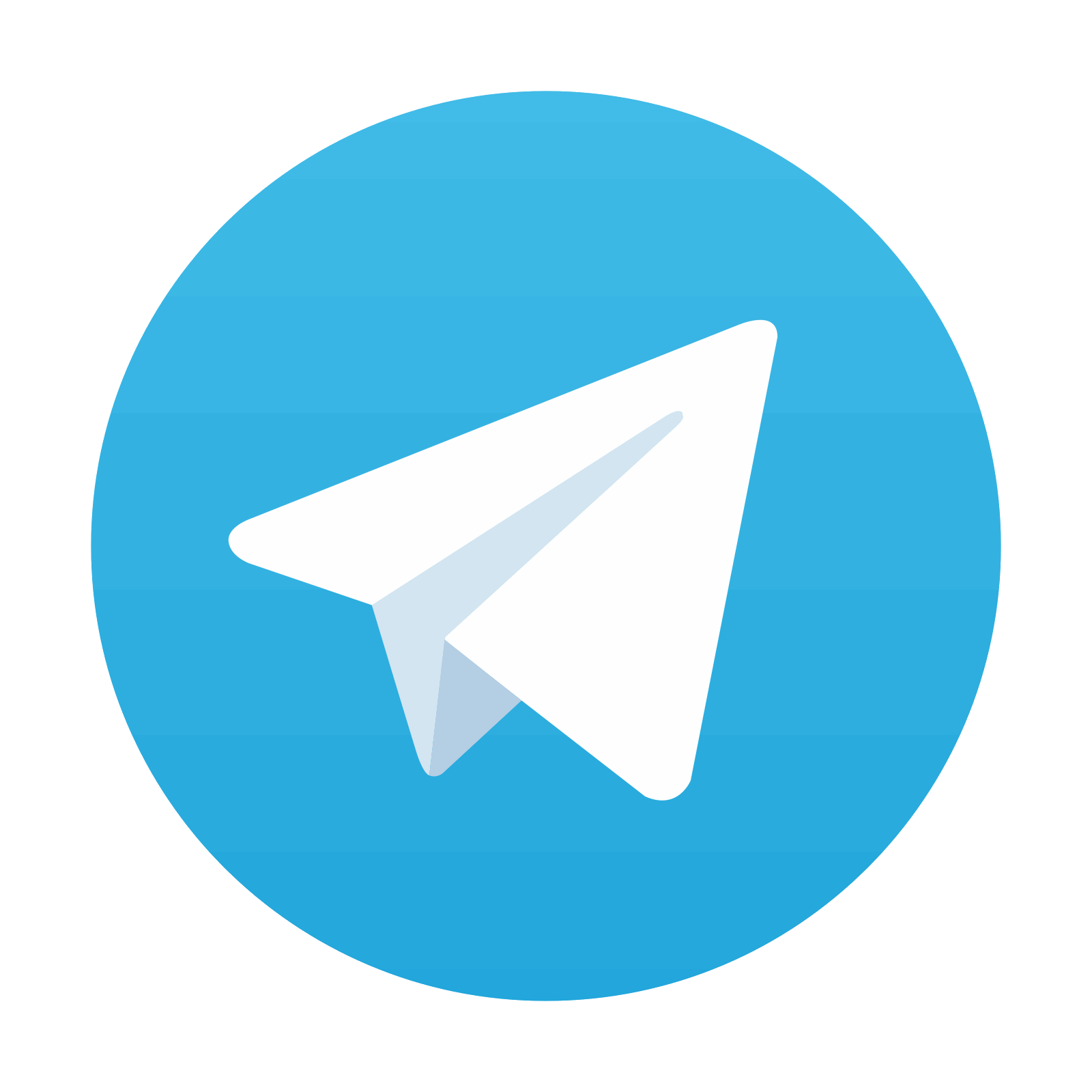
Stay updated, free articles. Join our Telegram channel

Full access? Get Clinical Tree
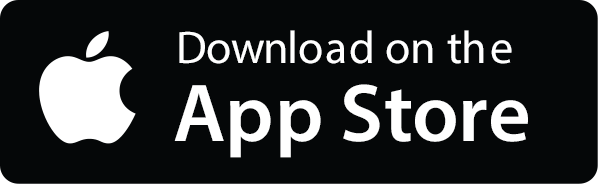
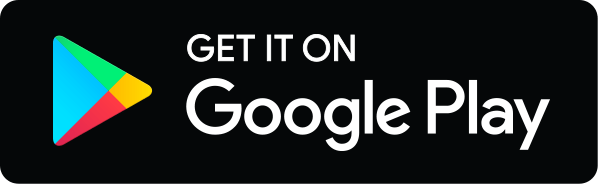