Effects of Drugs on TSH Secretion, Thyroid Hormones Absorption, Synthesis, Metabolism, and Action
Sébastien Thalmann
Christoph A. Meier
Introduction
Various drugs and other substances are known to interfere with thyroid hormone homeostasis. Although the actions of some compounds on thyroid hormone secretion and metabolism are considered as adverse effects, the inhibitory effect of certain molecules (e.g., thionamides, perchlorate, and iodinated compounds) is exploited clinically for the treatment of hyperthyroidism.
Pharmacologic agents may influence thyroid hormone homeostasis at different levels (Fig. 11B.1): At the pituitary or hypothalamic level they may alter thyrotropin (TSH) secretion; in the gut, thyroid hormone absorption may be reduced; at the thyroid, the synthesis and/or secretion of thyroid hormones may be altered by pharmacological agents and these agents may change the serum concentrations of thyroid hormones by influencing either the level of binding proteins or by competing for their hormone-binding sites. Drugs may also modify the cellular uptake and metabolism of thyroid hormones. Lastly, pharmacologic agents may interfere with hormone action at the target tissue level. Some agents interfere simultaneously at different levels.
Although most drug-induced changes in thyroid hormone homeostasis are transient, they may hinder the interpretation of thyroid function tests. However, with improvements in the quality of routine measurements of serum-free thyroid hormone and thyroid-stimulating hormone (TSH; thyrotropin) concentrations, the latter difficulties have decreased in importance (Table 11B.1).
Drugs that suppress serum TSH levels
Dopamine/Dopamine Agonist
Dopamine, which is mostly used in intermediate or critical care units, and dopamine agonists, such as bromocriptine or cabergoline, which are used in patients with hyperprolactinemia, can suppress serum TSH through their action on dopamine D2 receptor (DR2) (1). Interestingly, dopamine appears to have opposite effects on the hypothalamus; for example, dopamine infusion in healthy volunteers has been shown to reduce only TSH amplitude, but not frequency (2). In rats, the secretion of thyrotropin-releasing hormone (TRH) was increased at the hypothalamus, also via DR2, but the overall dominating effect was inhibitory on the pituitary and the TSH levels were reduced (3). As in non-thyroidal illness, dopamine infusion in critical care patients may cause central hypothyroidism (4,5). Whether hormonal replacement therapy in these patients is needed remains unknown.
Somatostatine Analogs
Somatostatin analogs are used clinically in thyroid disease due to a TSH-secreting pituitary adenoma that may not have been cured by surgery (6) as well as for the treatment of neuroendocrine tumors and variceal bleeding from the esophagus
(7). Contrary to dopamine agonist, both the frequency and amplitude of TSH secretion were reduced during administration of somatostatin and this effect appears to be a direct inhibtion of TSH secretion (2). In patients with acromegaly, after 1 month of octreotide therapy, TSH levels were reduced and TRH-stimulation of TSH was lowered (8); however, after 6 months of therapy with octreotide, basal TSH and free T4 levels were in the normal range. In diabetic patients treated for 1 year with octreotide infusion for diabetic retinopathy, TSH levels were modestly reduced, but without clinical hypothyroidism (9). Even if there seems to be a direct effect of somatostatin on TSH secretion, this effect is only transient and does not appear to lead to overt hypothyroidism.
(7). Contrary to dopamine agonist, both the frequency and amplitude of TSH secretion were reduced during administration of somatostatin and this effect appears to be a direct inhibtion of TSH secretion (2). In patients with acromegaly, after 1 month of octreotide therapy, TSH levels were reduced and TRH-stimulation of TSH was lowered (8); however, after 6 months of therapy with octreotide, basal TSH and free T4 levels were in the normal range. In diabetic patients treated for 1 year with octreotide infusion for diabetic retinopathy, TSH levels were modestly reduced, but without clinical hypothyroidism (9). Even if there seems to be a direct effect of somatostatin on TSH secretion, this effect is only transient and does not appear to lead to overt hypothyroidism.
Rexinoids
Rexinoids are a subclass of retinoids, which bind specifically to the retinoid X receptors (RXR), a nuclear hormone receptor subfamily playing an important role in cell regulation, proliferation, apoptosis, and differentiation. RXR binds with other nuclear transcription factors, including thyroid hormone receptor, retinoic acid receptor, vitamin D receptor, and peroxisome proliferator-activated receptor (PPAR), a heterodimer which influences transcription of many different target genes. These agents have been studied for the treatment of lung, breast, and thyroid cancers. Only bexarotene has been approved and is used for cutaneous T-cell lymphoma (10). In one study of 27 patients treated with bexarotene for cutaneous T-cell lymphoma, TSH was reversibly reduced in almost all patients associated with clinical signs of hypothyroidism in 19 of 27 subjects (11). However, as this effect was seen in advanced tumor patients, another study examined the effect of single doses of bexarotene in healthy subjects, which showed only a selective reduction of TSH without affecting other pituitary hormones (12). It has also been speculated that rexinoids may alter thyroid hormone metabolism through deiodination, sulfonation, and possibly glucuronidation. As these agents may be used more frequently in the future, one should be alert to possible development of thyroid dysfunction in these patients.
Other Possible Drugs that Suppress TSH Secretion
Certain antiepileptic drugs such as carbamezipine, oxcaremazepine, valproic acid, and phenytoin, which are known to increase the metabolism of thyroid hormones through the P450 hepatic system and which will be discussed later, may also alter pituitary TSH secretion and cause central hypothyroidism (13). However, this remains controversial except for phenytoin as other groups did not show any alteration in TSH secretion (14).
Metformin, the first-line recommended antidiabetic agent in type 2 diabetes, reduces TSH levels in patients with hypothyroidism (treated or not) without altering free T4 levels, but
not in diabetics without concomitant hypothyroidism (15). The exact mechanism remains unknown.
not in diabetics without concomitant hypothyroidism (15). The exact mechanism remains unknown.
Table 11B.1 Compounds Interfering with Thyroid Function | ||||||||||||||||||||||||||||||||||||||||||||||||||||||||||||||||||||||||||||||||||||||||||||||||||||||||||||||||||||||||||||||||||||||||||||||||||||||||||||||||||||||||||||||||||||||||||||||||||||||||||||||||||||||||||||||||||||||||||||||||||||||||||||||||||||||||||||||
---|---|---|---|---|---|---|---|---|---|---|---|---|---|---|---|---|---|---|---|---|---|---|---|---|---|---|---|---|---|---|---|---|---|---|---|---|---|---|---|---|---|---|---|---|---|---|---|---|---|---|---|---|---|---|---|---|---|---|---|---|---|---|---|---|---|---|---|---|---|---|---|---|---|---|---|---|---|---|---|---|---|---|---|---|---|---|---|---|---|---|---|---|---|---|---|---|---|---|---|---|---|---|---|---|---|---|---|---|---|---|---|---|---|---|---|---|---|---|---|---|---|---|---|---|---|---|---|---|---|---|---|---|---|---|---|---|---|---|---|---|---|---|---|---|---|---|---|---|---|---|---|---|---|---|---|---|---|---|---|---|---|---|---|---|---|---|---|---|---|---|---|---|---|---|---|---|---|---|---|---|---|---|---|---|---|---|---|---|---|---|---|---|---|---|---|---|---|---|---|---|---|---|---|---|---|---|---|---|---|---|---|---|---|---|---|---|---|---|---|---|---|---|---|---|---|---|---|---|---|---|---|---|---|---|---|---|---|---|---|---|---|---|---|---|---|---|---|---|---|---|---|---|---|---|---|---|---|---|---|---|---|---|---|---|---|---|---|---|---|---|
|
Thyroid hormone absorption
Normally about 80% of a given dose of levothyroxine is absorbed within 6 hours, mainly in the jejunum and ileum. Several drugs are known to markedly reduce the absorption of levothyroxine such as cholestyramine, calcium carbonate, aluminum hydroxide, ferrous sulfate, and sucralfate, which results from either the formation of an unabsorbable complex or from a direct inhibition of absorption (16).
Bile Acid Sequestrants
Bile acid sequestrants are known to reduce intestinal absorption of thyroid hormones (17). In rats, absorption was reduced by colestipol; however, this effect could not be reproduced in humans (18). Cholestyramine, another member of this class of drugs, has been shown to dramatically reduce intestinal absorption of radiolabeled levothyroxine (17). The authors
concluded that about 50 mg cholestyramine could bind up to 3,000 μg of levothyroxine. In rats, the level of absorption was only 2.3% compared with 74% without cholestyramine. In human studies, a minimal interval of 4 to 5 hours was necessary to obtain near normal free T4 plasma values.
concluded that about 50 mg cholestyramine could bind up to 3,000 μg of levothyroxine. In rats, the level of absorption was only 2.3% compared with 74% without cholestyramine. In human studies, a minimal interval of 4 to 5 hours was necessary to obtain near normal free T4 plasma values.
Ferrous Sulfate
The intake of iron in the form of oral ferrous sulfate can lead to diminished intestinal absorption, resulting in increased TSH levels. In vitro experiments show that one atom of Fe3+ binds to three T4 molecules to form an insoluble complex, which may not be absorbed from the intestinal lumen (19). Pregnant women and older people taking levothyroxine with iron-containing medications should be instructed to differ the time of intake of these two drugs.
Table 11B.2 Pharmacokinetics of Antithyroid Drugs | ||||||||||||||||||||||||||||||||
---|---|---|---|---|---|---|---|---|---|---|---|---|---|---|---|---|---|---|---|---|---|---|---|---|---|---|---|---|---|---|---|---|
|
Sucralfate and Aluminum-containing Antacids
Sucralfate is an aluminum-containing salt of sucrose sulfate, which decreases thyroid hormone absorption up to 23%, resulting in lower T4 and higher TSH serum levels (20). This problem can be circumvented by separating the intake of both drugs by 8 hours. The malabsorption of levothyroxine in the presence of sucralfate may be due to either the formation of an insoluble complex or the inhibition of hormone transport by intestinal cells. Similar to sucralfate, the bioavailability of levothyroxine may be reduced by aluminum-containing antacids, where the cationic aluminum may also complex with levothyroxine. In a small study where hypothyroid participants received 2,256 mg of aluminum hydroxide in four divided doses, the mean levels of TSH increased from 2.6±0.8 mU/L (mean±SD) to 7.2±1.3 mU/L (21).
Calcium Carbonate and Other Phosphate Binders
Calcium carbonate, which is used in chronic renal failure as a phosphate binder, may also interfere with thyroid hormone absorption. Other phosphate binders such as calcium acetate or the calcium-free cationic binder, sevelamer hydrochloride, also interfere as shown in a study of 67 hemodialysis patients treated with levothyroxine (22). Intake of either calcium carbonate or sevelamer was associated with TSH elevation, which further increased over time. In a prospective study of 20 patients on stable levothyroxine substitution, concomitant intake of 1,200 mg of calcium carbonate lead to a significant, but reversible, increase in TSH (23). In healthy volunteers taking 1 mg of levothyroxine together with 2 g of calcium or placebo, the maximal total T4 absorption was only 579 μg with coadministration of calcium, as compared to 837 μg with placebo (24). The new phosphate binder lanthanum carbonate also reduces the area under the curve of T4 absorption (25).
Taken together, all these data indicate that a class-effect may be possible and that TSH controls are necessary when introducing one of these medications; moreover, patients on T4 therapy should be advised not to take their medication simultaneously with any other drugs.
Other Medications that May Reduce T4 Intestinal Absorption
Raloxifene, a selective estrogen receptor modulator (SERM), may influence the absorption of T4, as described in case reports. Proton-pump inhibitors, which are widely used in western countries, have shown conflicting data on intestinal levothyroxine absorption. In patients with multinodular goiter receiving levothyroxine therapy, patients taking omeprazole showed an elevation of TSH and consequently needed a 37% increase in their levothyroxine doses (26). Lansoprazole showed similar effects in a retrospective study (27). However, studies investigating the acute effect of proton-pump inhibitors on T4 absorption did not show any change in the area under the curve of T4. However, it is prudent to look for symptoms of hypothyroidism in the follow-up of patients chronically taking proton-pump inhibitors (28,29). Recently, it has been described that orlistat can reversibly interfere with the absorption of many drugs, including levothyroxine, as reported in a recent case (30); however, so far no other cases have been reported with orlistat.
Synthesis and secretion of thyroid hormones
Thionamides (Antithyroid Drugs)
Three compounds of this class are the main therapeutic arsenal for treating hyperthyroidism. In the United States, propylthiouracil (PTU) and methimazole are the most frequently used antithyroid drugs, whereas in Europe carbimazole is the main antithyroid drug. Carbimazole differs from methimazole by a carboxy side chain, which is cleaved off during the first liver passage, converting carbimazole to methimazole.
All three compounds are completely absorbed, and their metabolism is barely affected by liver or kidney disease (31). The thyroid avidly concentrates these compounds. This uptake is more pronounced in very active glands, for instance in iodine deficiency or hyperthyroidism. The thyroid also plays a major role in the degradation of these drugs (32,33). In the rat, S35-labeled metabolites of PTU can be detected up to 40 hours after injection. In vitro studies indicate that the intrathyroidal degradation of PTU and methimazole is strongly influenced by the intrathyroidal iodide content. However, the serum half-life is less than 6 hours for methimazole and less than 2 hours for PTU (Table 11B.2). Clinical studies have shown effects of methimazole lasting as long as 24 to 36 hours, and a single dose of PTU was shown to be effective for more than 12 hours. This correlates well with the intrathyroidal presence of S35-labeled metabolites of these drugs. The intrathyroidal mechanism of action of the three compounds is similar. They inhibit hormone synthesis by thyroid peroxidase (TPO). This synthesis can be separated into two steps, whereby initially a tyrosine of thyroglobulin (Tg) is iodinated followed by a coupling reaction, also under the control of TPO, resulting in the formation of an ether bond between two diiodotyrosines of Tg and the formation of T4 and to a minor extent, the formation of T3.
Both reactions are inhibited by thionamides. There is a marked competition between iodide and the thionamides for the active site of TPO. The mechanism of this competition is complex. There is competitive inhibition for the active site, and the residence time of PTU or methimazole is inversely related to the intrathyroidal iodide concentration (32,33). In vitro it was possible to demonstrate that the degradation of S35-labeled PTU was extremely slow in iodine deficiency and accelerated with increasing iodide concentrations. In situations of severe iodine contamination, these are the two major mechanisms leading to the loss of efficiency of these drugs. It is also likely that iodine contamination reduces the capacity of the thyroid to concentrate the thionamides.
Both reactions are inhibited by thionamides. There is a marked competition between iodide and the thionamides for the active site of TPO. The mechanism of this competition is complex. There is competitive inhibition for the active site, and the residence time of PTU or methimazole is inversely related to the intrathyroidal iodide concentration (32,33). In vitro it was possible to demonstrate that the degradation of S35-labeled PTU was extremely slow in iodine deficiency and accelerated with increasing iodide concentrations. In situations of severe iodine contamination, these are the two major mechanisms leading to the loss of efficiency of these drugs. It is also likely that iodine contamination reduces the capacity of the thyroid to concentrate the thionamides.
Side Effects of Thionamides
The side effects for all three thionamide drugs are similar. At least 5% of patients initially complain of pruritus and more rarely of an urticarial rash and drug fever. These symptoms usually appear during early treatment and are more likely at high doses. Reducing the dose is in general helpful, but in an occasional patient one has to stop treatment or may have to switch to methimazole or vice versa. Occasionally, the drugs, and PTU in particular, can be responsible for a bitter taste. This is a congenital anomaly and does not disappear with time. Carbimazole and methimazole may produce cholestatic jaundice, whereas PTU occasionally increases serum transaminases. Fulminant hepatic failure has been reported (34). Even though the milder hepatic side effects can be alleviated by reducing the dose, one will often prefer in such situations to treat with iodine 131 (131I) or thyroidectomy. See Chapter 32 for a more detailed use of the antithyroid drugs.
The major serious complication of these drugs is agranulocytosis (<500 neutrophils/mm3). It is a rare complication and probably occurs with all three drugs in less than 0.25% of cases. Its onset is abrupt and should not be confused with the mild decrease in the white blood cell counts seen during the first few days of treatment. Due to the rapid appearance of agranulocytosis, monitoring of the white blood cell count is generally not helpful. Patients must, however, seek medical care in case of a sore throat and fever and stop treatment until the white blood cell count is analyzed. Stopping antithyroid drug treatment is sufficient to permit complete recovery in almost all patients, although treatment with granulocyte growth factors may be indicated in patients with infections.
Special Indications and Differences Between Propylthiouracil and Methimazole
PTU differs in two clinically relevant aspects from methimazole and carbimazole. Probably due to the very short plasma half-life of PTU, its transplacental effects are minor, and it is excreted less in maternal milk. Therefore, PTU has been for long time the drug of choice in pregnancy (38,39,40), as methimazole has been associated with gut defects in the first trimester (41). However, more recent data suggest a rare but potentially fatal PTU hepatotoxicity (42). The 2011 guideline from the ATA and AACE recommend the use of PTU in the first trimester and then to switch to methimazole (43). In the postpartum period, methimazole is also very safe and its tolerance is even better documented than for PTU (44).
In cases of severe iodine contamination, PTU has probably an advantage over methimazole because it offers a second site of action, since only PTU has an inhibitory effect on monodeiodinase type 1 activity. However, in humans this effect can only be achieved with large doses of PTU and it results in only a 30% reduction of serum T3 levels; in practice, therefore, the therapeutic advantage is small.
In vitro, methimazole and later PTU were shown to have an immunosuppressive effect. The relevance of this effect on remission rates is debated, but a difference has not been detected in the cure and relapse rates of Graves’ hyperthyroidism treated with high or appropriate doses of methimazole (45).
Ionic Inhibitors (Perchlorate, Thiocyanate, and Nitrate)
The molecular mechanism of iodide trapping by the thyroid has been elucidated through the cloning of the thyroid sodium iodide (Na+/I–) symporter (NIS) (46). Two anionic compounds, perchlorate and thiocyanate, are of clinical relevance. These are bulky anions, which sterically resemble iodide. Their main mode of action is to compete with iodide for the symporter, while perchlorate is a far more potent inhibitor
than thiocyanate. Perchlorate is also capable of discharging intrathyroidal iodide rapidly and may have additional points of impact, such as the transport of iodide from the cell into the lumen of the follicles (47). Perchlorate can accumulate in the follicular cell; as for iodide, its accumulation is dependent on TSH stimulation. The trapping of iodide is closely linked to the Na+/K+ ATPase-dependent countertransport of Na+, and here, too, perchlorate could have an inhibitory function (48). The peak blood levels occur approximately 3 hours after oral intake, and its intrathyroidal concentration peaks at 4 to 6 hours. Its actual duration of action has not been studied in detail, but it is believed to have a short duration of action and is therefore preferably given two to four times daily.
than thiocyanate. Perchlorate is also capable of discharging intrathyroidal iodide rapidly and may have additional points of impact, such as the transport of iodide from the cell into the lumen of the follicles (47). Perchlorate can accumulate in the follicular cell; as for iodide, its accumulation is dependent on TSH stimulation. The trapping of iodide is closely linked to the Na+/K+ ATPase-dependent countertransport of Na+, and here, too, perchlorate could have an inhibitory function (48). The peak blood levels occur approximately 3 hours after oral intake, and its intrathyroidal concentration peaks at 4 to 6 hours. Its actual duration of action has not been studied in detail, but it is believed to have a short duration of action and is therefore preferably given two to four times daily.
The first reports of the clinical use of perchlorate date to the 1950s. Its initial popularity for treatment of hyperthyroidism was brought to a halt when irreversible aplastic bone marrow suppression was reported. Other side effects are rashes, drug fever, lymphadenopathy, and agranulocytosis, but these complications are likely to be less frequent than with thionamides; moreover, this risk is very small or even absent with doses of 500 mg b.i.d. or lower. Some rare cases of nephrotic syndrome also have been reported. The appearance of aplastic anemia has limited the use of this drug to few and specific clinical conditions. In case of congenital goiters due to genetic defects of thyroid hormone synthesis, the perchlorate discharge test is the diagnostic standard for revealing an abnormally large pool of intracellular iodide that has not been incorporated into thyroglobulin. This metabolic block can be mimicked by thionamides, which induce a functional block at the level of the TPO. There are two variations of the perchlorate discharge test. The classical test uses only 1 g perchlorate, which is given 2 hours after administration of iodine 123 (123I). Uptake of 123I is measured just before administrating perchlorate. The decrease of thyroidal 123I is measured 1 and 2 hours later. In normal thyroids the discharge should not exceed 30% of the accumulated thyroidal dose. A modification of this test has been introduced to reveal small partial organification defects. In this case, 500 mg iodide is administrated at the same time as the 123I. This leads to a transient increase in intracellular free iodide, and perchlorate can reveal small defects that are not seen with the classical test. This modification has been applied to demonstrate mild organification defects in autoimmune thyroiditis.
Perchlorate is widely used for short-term inhibition of thyroidal uptake of radioactive iodide or Tc99m-perchlorate in diagnostic nuclear medicine. However, the use of 123I- or 131I-labeled substances in diagnostic procedures in nuclear medicine has markedly decreased. Moreover, perchlorate can also be used for decreasing thyroidal iodine contamination due to iodinated contrast media used for angiographic studies or CT scans. It is also indicated for the treatment of severe cases of amiodarone- or iodine-induced hyperthyroidism, which can be a life-threatening situation. The addition of 0.5 g perchlorate twice daily to high-dose PTU treatment has shortened the period of hyperthyroidism (see Chapter 32). In contrast to perchlorate, thiocyanate is not used in clinical medicine. Perchlorate is no longer available commercially in the United States, but can be compounded by pharmacists using reagent grade perchlorate.
Nitrate is about 240 times less potent than perchlorate as a competitive inhibitor of the sodium/iodine symporter and is found in soil and groundwater (49). Its action on thyroid glands will be discussed further in Chapter 57.
Lithium
Lithium, used in the treatment of bipolar depression, is associated with subclinical and overt hypothyroidism in up to 34% and 15% of patients, respectively, which can appear abruptly even after many years of treatment. Therefore, patients should be regularly examined for symptoms and signs of thyroid dysfunction, and thyroid function tests should be performed once or twice a year (50,51). The inhibitory effect of lithium occurs mainly at the level of hormone secretion, although effects on iodine trapping, release, and coupling have been described. However, no significant interference of lithium on thyroid hormone metabolism or action has been described in humans.
In contrast to lithium-induced hypothyroidism, lithium-associated thyrotoxicosis is rare and occurs mainly after the long-term use of this drug (52,53). The mechanisms are either an autoimmune destructive thyroiditis, or Graves’ disease including the development of ophthalmopathy (50).
Drugs that Influence Metabolism of Thyroid Hormones
Drugs that Induce the Hepatic p450 Complex (Mixed-function Oxygenases)
Many lipophilic drugs are rendered water soluble by oxidation and conjugation in the liver before their elimination from the body by enzymes being part of the type II metabolic processes (58). The type I metabolic processes include enzymes responsible for the oxidative and reducing reactions and belong to the cytochrome p450 complex that consists of more than 100 isoenzymes. Some of these enzymes (CYP3A) can be induced by antiepileptic agents such as phenytoin, phenobarbital, and carbamazepine, as well as the antituberculous drug, rifampicin.
Antiepileptic Agents
It has been known for over 40 years that the administration of phenytoin causes alterations in serum thyroid hormone levels (59,60). Serum thyroid hormone levels can decrease markedly under phenytoin and rifampicin; the effects on serum TSH are, however, minor. Similar but less important effects have been reported for carbamazepine and phenobarbital. These agents may potentiate the effects of the other antiepileptic agents when used in combination with them (61).
In normal subjects, the hypothalamic-pituitary system is capable to compensate by augmenting thyroid hormone production and secretion, but in subjects with subclinical or overt hypothyroidism treated with these antiepileptic drugs, hypothyroidism may worsen.
Phenytoin is of particular interest because its effects are not limited to the induction of hepatic drug metabolism, as will be discussed separately later.
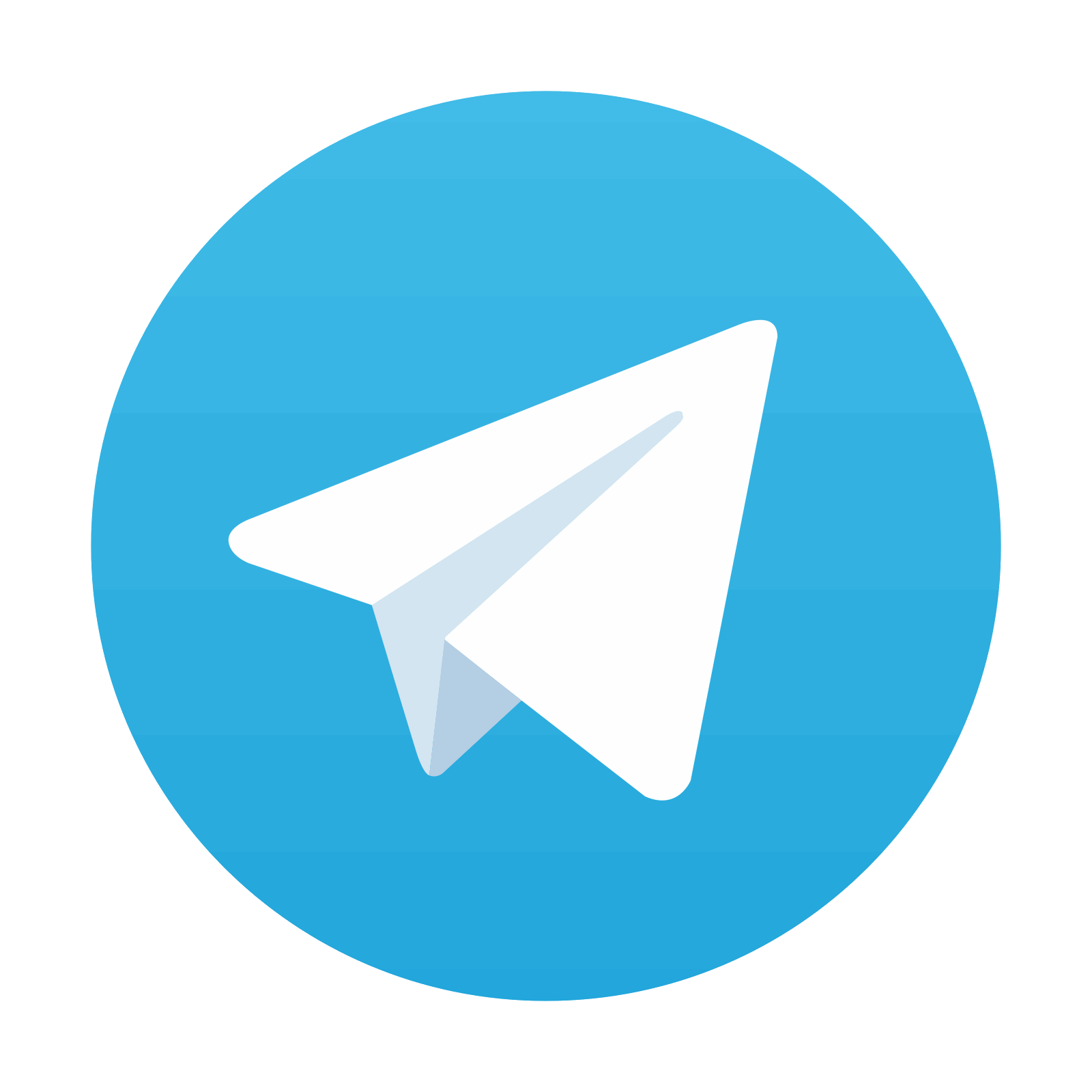
Stay updated, free articles. Join our Telegram channel

Full access? Get Clinical Tree
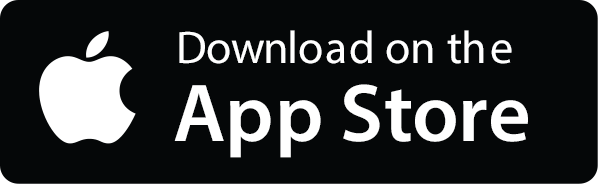
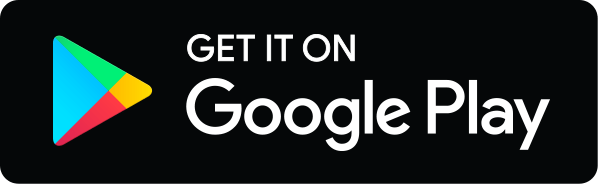