Thyroid Hormones in Thermogenesis, Intermediary Metabolism, and Obesity
Thomas Reinehr
Introduction
Thyroid hormones can modulate numerous cellular processes that are relevant for thermogenesis, basal metabolic rate, and consequently for body weight (1,2,3). The association between thermogenesis and thyroid hormones explains the cold intolerance in hypothyroidism as well as the heat intolerance in thyrotoxicosis. At least 30% of the heat produced from basal metabolic rate (basal thermogenesis), is thyroid hormone dependent, and thyroid hormones are essential for the adaptive thermogenic response to cold exposure (4,5). Clinicians are familiar with several metabolic abnormalities in hypothyroid patients, most notably increased total and low-density lipoprotein (LDL) cholesterol and hypertriglyceridemia. Such manifestations are a reflection of diminished or absent thyroid hormone on various intermediary metabolism pathways.
While it is well known that hyperthyroidism leads to weight loss, and hypothyroidism is associated with weight gain, there has been an increasing focus on the relationship between thyroid function and body weight in recent years. Obesity is associated with moderately increased thyroid stimulating hormone (TSH) and triiodothyronine (T3) levels, while anorexia nervosa (AN) is associated with decreased TSH and T3 concentrations in humans. Low serum T3 levels in starvation and in underweight subjects, and high serum T3 levels in obesity and overfeeding suggest a role for T3 in metabolic adaptation to these situations: Increased basal metabolic rate and thermogenesis in obesity and decreased metabolic rate in AN (3,6). The low serum T3 levels in AN adds to energy sparing, and thus to metabolic economy during starvation, while the increased serum T3 levels in obesity increase resting energy expenditure (REE), leading to energy consumption by reducing the availability of energy for conversion into fat (6,7). White adipose tissue, the largest energy store in the body, actively produces various hormones, cytokines, and chemokines, which together exert important roles in homeostasis and also in thyroid hormone regulation (8,9). The adipokine leptin seems to be the most promising link between body weight and TSH levels since leptin stimulates the hypothalamic–pituitary–thyroid axis (6,10).
Thermogenesis
Thermogenesis is composed of basal thermogenesis, which occurs in every living cell and adaptive thermogenesis, which is an adaptive process in reaction to cold exposure.
Basal thermogenesis is mainly the result of adenosine triphosphate (ATP) metabolism (see Fig. 26.1) (5). The energy contained in substrates is largely released in the mitochondria captured in the form of ATP. The energy contained in ATP is then used to sustain vital functions. A fraction of the energy involved both in the synthesis of ATP and in its utilization is lost as heat (5,11). Thyroid hormones increase basal thermogenesis, seemingly by a dual mechanism (5): Increasing ATP
demands due to increased metabolic activity, and by reducing the thermodynamic efficiency of ATP synthesis.
demands due to increased metabolic activity, and by reducing the thermodynamic efficiency of ATP synthesis.
Adaptive thermogenesis is characterised by an uncoupling of oxidative phosphorylation in cold-exposed brown adipose tissue (BAT), stimulated by the sympathetic nervous system and amplified by thyroid hormones. Recently, the existence of BAT has been proven in adult humans, especially in the supraclavicular region (12). Interestingly obese individuals seem to have less BAT mass and activity than do their lean counterparts (13). This may be related to lower energy consumption for thermogenesis in obesity.
In small mammals, sympathetic adrenergic stimulation of BAT induces uncoupling protein-1 (UCP-1). UCP-1 is a protein that uncouples the mitochondrial proton gradient from ATP production, thereby promoting the generation of heat. A critical element in this pathway is the type 2 deiodinase (D2), which increases local intracellular T3 production from thyroxine (T4), increasing thyroid hormone receptor saturation from 70% to nearly 100% upon cold exposure (14). Serum T3 levels do not appear to be affected by cold exposure, however (14). Studies of the D2-knockout mouse have confirmed the centrality of D2-generated T3 in cold-induced BAT thermogenesis: These mice have impairment in the nonshivering thermogenic response to cold exposure that can be rescued by administration of T3 (15).
In adaptive thermogenesis, thyroid hormones interact with the sympathoadrenal system (4,5): The norepinephrine signal is potentiated by thyroid hormones, resulting in an increase in enzymatic activities needed for thermogenic function.
Despite BAT, skeletal muscle is assumed to play an important thermogenic role in humans, given its mass, innervation, and metabolic activity. A significant portion of the variation in basal metabolic rate among normal humans could be attributable to this tissue alone (16). Considerable data exist in support of a role for T3 in skeletal muscle-based obligatory thermogenesis. The breadth of possibilities is highlighted by a microarray-based study that examined the effect of 75 μg of T4 daily on the vastus lateralis transcriptosome in healthy male volunteers. This study reported that 22 genes related to mitochondrial energy metabolism were upregulated, as were uncoupling-related genes including UCP-3 and adenine nucleotide translocases 1 and 2 (17). It has also been demonstrated that short-term T3 administration to healthy human subjects leads to a T3-driven uncoupling mechanism in the skeletal muscle, with an increase in tricarboxylic acid cycle flux without changing the rate of ATP synthesis (18). Several studies have produced consistent evidence that T3 promotes the uncoupling of ATP hydrolysis from sarcoplasmic calcium cycling, increasing the energy turnover associated with calcium cycling during contraction and rest, via transcriptional regulation of the sarcoplasmic reticulum calcium ATPase isoform 1 (19,20). This mechanism has been best studied in the cardiac muscle, but could also potentially explain at least some of thyroid hormone’s effects on skeletal muscle energy expenditure. However, the molecules connecting T3 with the metabolic uncoupling seen in the muscle have not been precisely determined. Furthermore, other approaches towards establishing a link between T3 and uncoupling in non-BAT tissues have met with limited success (12). It was once thought that the third member of the uncoupling protein family, UCP-3, could be the missing link, given its highly T3-responsive expression in skeletal muscle. However, this molecule has not been proven to have a role in uncoupling, and its overall role in metabolism remains controversial (21,22).
Since thermogenesis is partly determined by thyroid hormones, thyrotoxicosis is associated with heat intolerance, and hypothyroidism with cold intolerance. Basal metabolic rate can decrease 30% or more in severe hypothyroidism, indicating that heat production is reduced by the same amount, or probably more (4,5). Severely hypothyroid patients may develop hypothermia after exposure to low environmental temperatures or to medications that compromise heat conservation. Thermogenesis is markedly reduced in these patients: Basal thermogenesis is low because of the slow rate of metabolism, and adaptive thermogenesis is decreased because of the diminished response to catecholamines and limited substrate availability (5). In thyrotoxicosis, both basal thermogenesis and the responsiveness to catecholamines in adaptive thermogenesis are increased. Since D2 is stimulated by norepinephrine and inhibited by T4 (4,5) in thyrotoxicosis, the activity of D2 in BAT and the generation of T3 in this tissue are reduced limiting heat generation.
Intermediary Metabolism
Thyroid hormones have widespread effects on intermediary metabolism. In contrast to other hormones and regulators of metabolism, thyroid hormones stimulate opposite processes in intermediary metabolism, such as lipolysis and lipogenesis, protein synthesis and degradation, and glucose consumption production. These pathways all have potential relevance for REE, and therefore for body weight, though assessing their relative importance remains difficult. T3-driven mechanisms that may determine energy expenditure have been proven for skeletal muscle (e.g., thermogenesis see above). Of course, T3-driven mechanisms in tissues other than skeletal muscle may ultimately prove to be important as well. For example, T3 may influence the work done by the heart, which may be responsible for up to 15% of overall energy expenditure at rest (23), or
perhaps cycling of fatty acids (24). Numerous studies have identified potential mechanisms for T3-mediated energy expenditure in liver, kidney, and other tissues (25). In summary, thyroid hormones are one major determinant of human REE, although not all of the underlying pathways are fully understood.
perhaps cycling of fatty acids (24). Numerous studies have identified potential mechanisms for T3-mediated energy expenditure in liver, kidney, and other tissues (25). In summary, thyroid hormones are one major determinant of human REE, although not all of the underlying pathways are fully understood.
Effects of Thyroid Hormones on Protein Metabolism
Thyroid hormones stimulate protein turnover by stimulating both protein synthesis and degradation (26). This effect is the result of multiple genes whose expression is directly or indirectly stimulated by thyroid hormones (see Chapter 8). Stimulation of protein synthesis is augmented by thyroid hormone in a dose-dependent manner (26). The effect of thyroid hormones on protein degradation is also dose related, and is the result of controlled, coordinated activation of various proteases (27). Even though protein degradation predominates over stimulation of protein synthesis in severe thyrotoxicosis, it is, in general, proportional to synthesis (28). On the other side, protein turnover and amino acid oxidation are reduced in hypothyroidism (29).
Effects of Thyroid Hormones on Carbohydrate Metabolism
Except in severe hypothyroidism, the effects of thyroid hormones on carbohydrate metabolism are of little clinical significance. Blood glucose concentrations are, in general, not altered in thyroid dysfunction, but the turnover of glucose is stimulated by thyroid hormones (30). Thus there is increased glucose consumption that is offset by increased glucose production. Accordingly, it has been found that the cycling between glycogenolysis and gluconeogenesis is stimulated by thyroid hormones (31). Fasting blood glucose is frequently reduced in severe hypothyroidism due to impaired gluconeogenesis. A key enzyme in gluconeogenesis is the cytosolic phosphoenolpyruvate carboxykinase (PEPCK), which catalyzes the generation of phosphoenolpyruvate from oxaloacetate. This enzyme is under complex regulation by glucagon, norepinephrine, glucocorticoids, and thyroid hormones (32). It has been shown that T3 stimulates the PEPCK gene directly (32). Furthermore, thyroid hormones also might regulate glucose transport via effects on glucose transporter 4 (GLUT-4) expression (33).
Effects of Thyroid Hormones on Lipid Metabolism
Thyroid hormones stimulate both the de novo synthesis of fatty acids (lipogenesis) and the degradation of lipids (lipolysis and fatty acid oxidation). Lipogenesis is stimulated by T3 mainly in the liver, with rather limited effects in white adipose tissue, kidney, and heart. This is the result of the stimulation of synthesis of key lipogenic enzymes at the transcriptional level (34). The hyperthyroid state is associated with increased fat utilization, which arises from increased synthesis in the liver and from triglyceride lipolysis in white adipose tissue. Thyroid hormones promote lipolysis indirectly, by enhancing the catecholamine-induced stimulation of the hormone-sensitive lipase in adipose tissue.
De novo lipogenesis is reduced in hypothyroidism, particularly in the liver, and this is corrected by thyroid hormone (34). Furthermore, hypothyroidism is associated with reduced lipoprotein lipase (LPL) and hepatic lipase activities, both of which respond to thyroid hormone replacement (35). These effects continue to increase, in a T3 concentration-dependent manner, in the transition to thyrotoxicosis (36). Such observations may explain the elevation in serum triglyceride levels frequently seen in hypothyroid patients (35,36).
Serum cholesterol levels have long been known to be sensitive to thyroid status. Total serum cholesterol is reduced in thyrotoxicosis and increased in hypothyroidism. Thyroid hormones reduce cholesterol serum concentrations in a dose-dependent manner (12), which is largely the result of thyroid hormonal stimulation of hepatic cholesterol uptake by LDL receptors. Thyroid hormones increase the concentration of the LDL receptor by stimulation of the expression of sterol regulatory element binding protein-2 (SREBP-2), a transcription factor that enhances LDL gene transcription and expression (37).
However, thyroid hormones also increase cholesterol synthesis, stimulating the rate-limiting enzyme 3-OH-methyl glutaryl CoA reductase (HMG CoA reductase) (38). By stimulating the synthesis of cholesterol (and the other basic components of the plasma membrane), thyroid hormones facilitate its effects and those of other hormones in cell function, remodeling, and differentiation. In addition, cholesterol constitutes the foundation for steroid hormone synthesis.
The relationship between thyroid hormone and body weight
Body weight is a consequence of the relationship between energy intake and energy consumption. Energy consumption is largely determined by physical activity and REE. Since thyroid hormones are one major determinant of human REE, they determine body weight, at least in part.
Since thyroid hormones up-regulate many metabolic pathways relevant for REE, it is not surprising that patients with thyroid diseases usually exhibit changes in body weight. Hypothyroidism is usually associated with decreased thermogenesis and metabolic rate, resulting in a modest weight gain, whereas hyperthyroidism is typically associated with an elevated metabolic rate and weight loss despite an increased appetite.
Although thyroid function is usually normal in obese subjects, it is known that TSH and body mass index (BMI) are positively correlated. In fact, many studies in humans have consistently demonstrated that higher serum TSH concentrations are found in obese children and adults compared with normal-weight individuals (7,39,40,41,42,43,44,45). Furthermore, not only has a positive correlation between BMI and serum TSH been demonstrated but also a positive correlation has been observed between weight gain over 5 years of follow-up and a progressive increase in serum TSH (46). Since the majority of studies report elevated concentrations of TSH (usually below 10 IU/L) in up to 25% of the obese subjects, the question
arises as to whether at least some obese subjects suffer from hypothyroidism. Several studies have excluded clinically relevant hypothyroidism in obese humans with moderately elevated TSH levels (6,7,40,41). Indeed, the elevated TSH levels in obesity are typically associated with normal T4 levels and normal up to slightly elevated T3 levels (3,7,39,40,41,42,43,44,45,47,48,49,50,51).
arises as to whether at least some obese subjects suffer from hypothyroidism. Several studies have excluded clinically relevant hypothyroidism in obese humans with moderately elevated TSH levels (6,7,40,41). Indeed, the elevated TSH levels in obesity are typically associated with normal T4 levels and normal up to slightly elevated T3 levels (3,7,39,40,41,42,43,44,45,47,48,49,50,51).
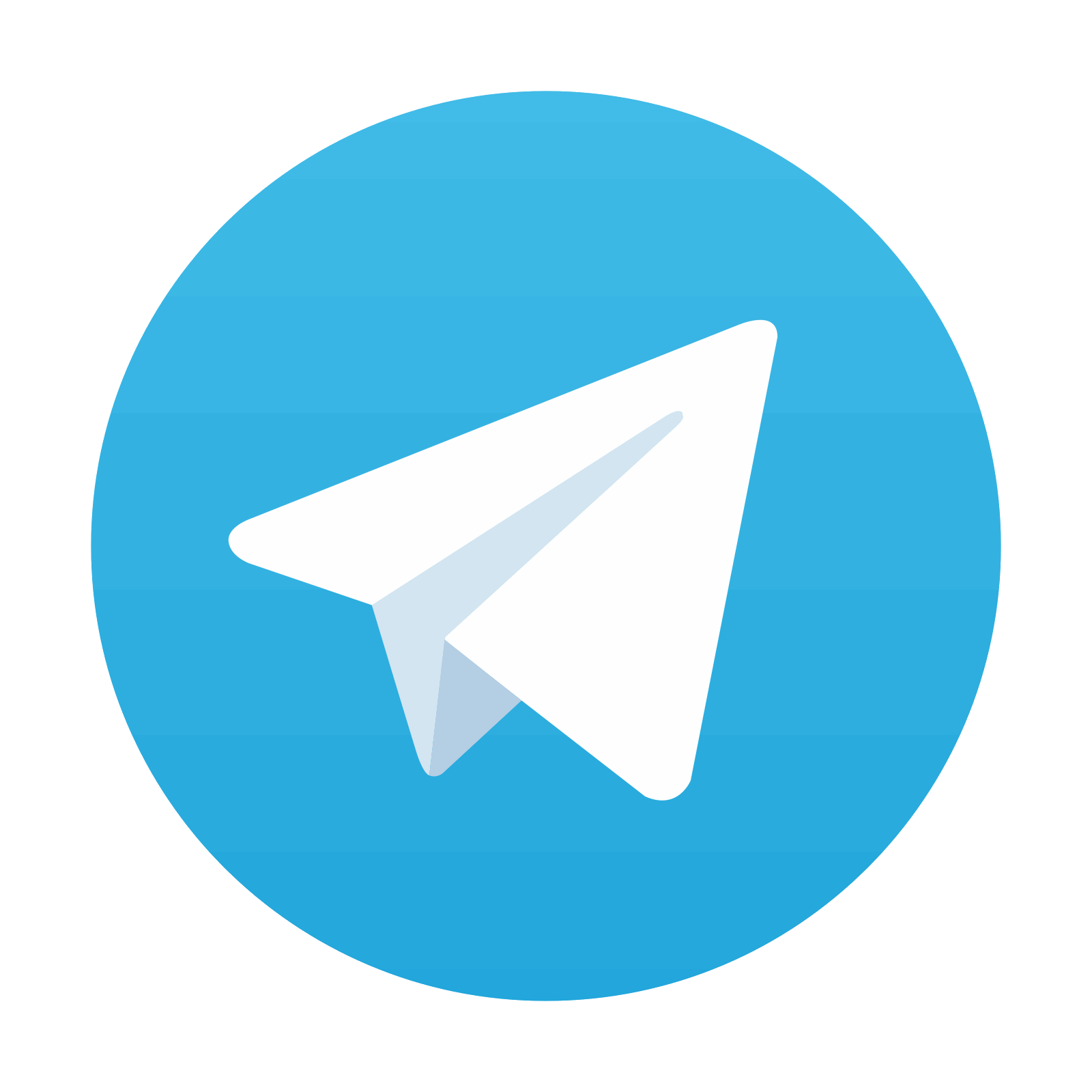
Stay updated, free articles. Join our Telegram channel

Full access? Get Clinical Tree
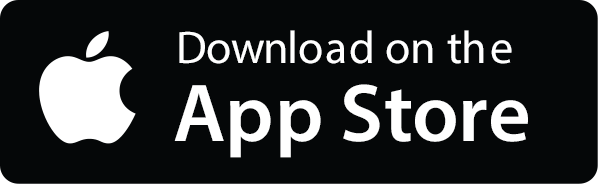
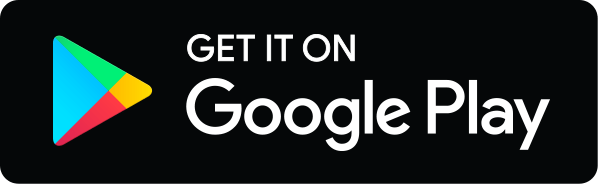