Toxic Adenoma and Toxic Multinodular Goiter
Ralf Paschke
Toxic adenoma (TA) and toxic multinodular goiter (TMNG) constitute the clinical presentations of thyroid autonomy, a condition where thyrocytes function and produce thyroid hormones independently of thyrotropin (TSH) and in the absence of TSH receptor (TSHR)–stimulating antibodies. The autonomous secretion of thyroid hormones leads to TSH suppression and quiescence in the nonautonomous thyroid tissue and can ultimately result in thyrotoxicosis.
Constitutive activation of the guanine (G) nucleotide–binding protein-coupled TSHR, or, less frequently, the α subunit of the G protein by somatic mutations in their respective genes, have been identified as the predominant molecular causes of thyroid autonomy (1,2).
TA is a clinical term referring to a solitary autonomously functioning thyroid nodule (AFTN) that produces thyroid hormones in excess. The autonomous properties of TA are readily seen on radioiodine or technetium (99mTc) imaging. The classical appearance is that of circumscribed increased uptake with suppression of uptake in the surrounding extranodular tissue (“hot” nodule, Fig. 20.1).
TMNG is a heterogeneous clinical disorder characterized by the presence of AFTNs in a goiter with or without additional nodules with normal or decreased uptake (cold nodules) on scintiscan. Thus, in TMNG the variable scintigraphic pattern includes circumscript areas of increased uptake with suppression of the surrounding thyroid tissue or an uneven (patchy) increase in uptake combined with areas of decreased and/or normal uptake. TMNG comprises the most frequent form of thyroid autonomy.
Epidemiology
Thyroid autonomy is a common finding in iodine-deficient areas, where it accounts for up to 60% of cases of thyrotoxicosis (TA, ∼10%; TMNG, ∼50%), (3,4). Several studies have suggested that TMNG primarily originates from euthyroid goiters. Scintigraphically nonsuppressible foci corresponding to autonomous thyroid tissue have been demonstrated in up to 40% of euthyroid patients with goiters in iodine-deficient areas (5,6). This is consistent with the finding that the prevalence of thyroid autonomy correlates with increased thyroid nodularity and increasing age (3,7,8). In contrast, thyroid autonomy is rare (3% to 10% of cases of thyrotoxicosis) in regions with sufficient iodine supply (3,9). Correction of iodine deficiency in a population results in a decrease of thyroid autonomy. An impressive 73% reduction in the prevalence of TMNG only 15 years after the doubling of the iodine content of salt has been observed in Switzerland (4,10). The likely molecular mechanisms linking iodine deficiency and thyroid autonomy are described below. Smoking has been identified as another environmental risk factor for TMNG in women (11,12). While Graves’ disease prevails in young people with thyrotoxicosis, TMNG tends to occur in older age groups (7,13). As with all thyroid diseases, TA and TMNG are more frequent in women (∼4 to 10:1) (13,14), and a thyroid growth promoting effect of estrogen described in vitro in rat FRTL-5 cells and thyroid cancer cell lines has been proposed as a possible contributing, constitutional effect of gender (15,16).
Clinical Aspects of Thyroid Autonomy
Clinical features in a patient with thyroid autonomy can be attributed to (a) symptoms of thyrotoxicosis and (b) the nodular goiter.
The clinical presentation of thyrotoxicosis varies with age, and in the elderly it is often not symptomatic (14,17). In a series of 84 French patients with overt thyrotoxicosis, atrial fibrillation and anorexia dominated in the older age group (≥70 years), while classical signs of thyrotoxicosis, e.g., nervousness, weight loss despite increased appetite, palpitations, tremor, and heat intolerance were more frequently observed in younger patients (≤50 years) (18). Alternatively, a patient may present with a lump or disfigurement of the neck, intolerance of a tight collar, or increase in collar size. Dysphagia or breathing difficulties due to esophageal or tracheal compression may be apparent, especially with large TMNG (14).
Subclinical thyrotoxicosis, defined by low or suppressed serum TSH with normal free thyroxine (T4) and free triiodothyronine (T3) levels is more commonly observed in older patients with TMNG (19,20). Moreover, the incidental finding of low or suppressed TSH levels on routine investigation for other indications is frequently the first evidence for the presence of thyroid autonomy, particularly in regions with iodine deficiency. Subclinical thyrotoxicosis may be associated with atrial fibrillation and a reduced bone density (19). In addition, an increased cardiovascular mortality rate in patients with low serum TSH levels has been described in a 10-year cohort study in the United Kingdom (21).
Very rarely thyroid autonomy occurs in conjunction with autosomal dominantly inherited non-autoimmune hyperthyroidism caused by activating germline mutations in the TSHR gene (1,23,24,25,26,27) (see Chapter 55). Clinical
characteristics comprise the presence of thyrotoxicosis and goiter in at least two generations with absence of typical diagnostic features of Graves’ disease, e.g., thyroid eye disease and TSHR antibodies. Furthermore, once antithyroid treatment has been stopped or after partial thyroidectomy, thyrotoxicosis will recur in all patients with activating TSHR germline mutations. Lastly, persistent neonatal thyrotoxicosis and unusual relapsing non-autoimmune thyrotoxicosis in childhood are highly suggestive of the condition irrespective of a positive family history. So far, 27 families and 15 children with sporadic occurrence of TSHR germline mutations have been reported in the literature (22). Thyroid ablation is advocated as first-line treatment (surgery and/or radioiodine) for preventing relapses. Molecular analysis for germline TSHR mutations offers the possibility for family screening, preclinical diagnosis, and genetic counseling (1,23,24,25,26).
characteristics comprise the presence of thyrotoxicosis and goiter in at least two generations with absence of typical diagnostic features of Graves’ disease, e.g., thyroid eye disease and TSHR antibodies. Furthermore, once antithyroid treatment has been stopped or after partial thyroidectomy, thyrotoxicosis will recur in all patients with activating TSHR germline mutations. Lastly, persistent neonatal thyrotoxicosis and unusual relapsing non-autoimmune thyrotoxicosis in childhood are highly suggestive of the condition irrespective of a positive family history. So far, 27 families and 15 children with sporadic occurrence of TSHR germline mutations have been reported in the literature (22). Thyroid ablation is advocated as first-line treatment (surgery and/or radioiodine) for preventing relapses. Molecular analysis for germline TSHR mutations offers the possibility for family screening, preclinical diagnosis, and genetic counseling (1,23,24,25,26).
Diagnosis of Thyroid Autonomy
Diagnosis of thyroid autonomy is based on three characteristics:
Confirmation of clinically suspected thyrotoxicosis by an abnormal thyroid function test. Typically, there is overt thyrotoxicosis (low TSH, high free thyroid hormones). However, dependent on the autonomous cell mass, subclinical thyrotoxicosis (TSH low, free T3 and free T4 normal), or even euthyroidism may be found.
Presence of palpable or sonographic nodule(s).
Increased radionuclide uptake in the nodule(s) concomitant with a decreased uptake in the surrounding extranodular thyroid tissue (Fig. 20.1). The most commonly used isotopes are 99mTc and 123I, both of which are transported into the thyrocytes by the sodium-iodide symporter (NIS), but only 123I is organified. Several studies have revealed a comparable diagnostic value of the two isotopes. However, discrepancies due to trapping only nodules can be found in about 5 % of hot nodules, mostly with normal serum TSH (28). The advantages of 99mTc, which is more frequently used in Europe, are its short half-life (6 hours) and low cost (29,30).
If thyroid autonomy is suspected in a euthyroid patient, a “suppression” scan can be performed after administration of thyroid hormone (i.e., 75 μg/day T4 for 2 weeks followed by 150 μg/day for 2 weeks), after which uptake in all nonautonomous tissue will be suppressed and thyroid autonomy is unmasked (5).
Measurement of thyroid autoantibodies is not routinely performed in TA and TMNG. However, in iodine-deficient areas, distinction between Graves’ disease and TMNG can be difficult if extrathyroidal manifestations of the former are absent and diagnostic findings are “atypical,” e.g., presence of thyroid nodules (∼27% to 34% by ultrasonography) in suspected Graves’ disease patients and patchy rather than diffuse uptake on scintiscan (31,32). In these conditions, determination of TSHR antibodies is helpful to establish the correct diagnosis (32,33). Urinary iodine excretion can be measured in case of suspected iodine contamination (13). Computed tomography (CT) and magnetic resonance imaging techniques are not routinely indicated for the diagnosis of thyroid autonomy, but may be used for presurgical planning in patients with large and partly intrathoracic goiters (14).
![]() Figure 20.2 Evolution of toxic nodules from a scintigraphically not detectable clone of mutated thyroid epithelial cells with increasing suppression of TSH to hyperthyroidism over years or decades. See color plate. |
Natural History
Long-term studies on patients with TA have shown that the natural course tends to be slow requiring years or decades (see Fig. 20.2). Sandrock et al. (34) reported an overall 4.1% annual incidence of thyrotoxicosis in a group of 375 untreated euthyroid TA patients in Germany who were followed for a mean of
53 months. Hamburger (9) highlighted the correlation between nodule size and development of thyrotoxicosis in a group of 349 patients with TA: 93.5% of patients with overt thyrotoxicosis had TA >3 cm in size and patients with a euthyroid TA of >3 cm in size had a 20% risk of developing thyrotoxicosis during a 6-year follow-up period, as opposed to a 2% to 5% risk in patients with nodules <2.5 cm in size.
53 months. Hamburger (9) highlighted the correlation between nodule size and development of thyrotoxicosis in a group of 349 patients with TA: 93.5% of patients with overt thyrotoxicosis had TA >3 cm in size and patients with a euthyroid TA of >3 cm in size had a 20% risk of developing thyrotoxicosis during a 6-year follow-up period, as opposed to a 2% to 5% risk in patients with nodules <2.5 cm in size.
Data on progression from euthyroid to subclinical and overt thyrotoxicosis in patients with multinodular goiter are scarce. An incidence of 9% to 10% of overt thyrotoxicosis has been proposed in two studies during a follow-up period of 7 to 12 years (14,34).
In addition, a sudden increase in TA and TMNG function can be induced by the administration of excessive amounts of iodine, e.g., in the form of contrast media used for angiography and CT scans or by iodine-containing drugs, e.g., amiodarone (35). In the European Study Group of Thyrotoxicosis, iodine contamination was found in 36.8% of patients from iodine-deficient areas with thyrotoxicosis (13). Severity of iodine deficiency, autonomous thyroid cell mass, and older age have been proposed as risk factors for the development of iodine-induced thyrotoxicosis (34) (see Chapter 11E).
Morphology
According to World Health Organization criteria, toxic thyroid nodules can be histologically classified as encapsulated follicular neoplasms (true adenomas) or adenomatous nodules, which lack a capsule (36). In addition, secondary changes such as hemorrhage, calcification, or cystic degeneration are frequently observed.
Benign thyroid nodules are distinguished by histology as encapsulated lesions (true adenomas) or adenomatous nodules, which lack a capsule, (2,36). By functional criteria, nodules are classified as either “cold,” “normal,” or “hot” depending on whether they show decreased, normal, or increased uptake on scintiscan. The classification of thyroid nodules on the basis of clonality studies implies that the majority of thyroid nodules are “true” thyroid tumors compared to polyclonal hyperplastic nodules. Traditionally, only thyroid adenomas are considered true tumors on the basis of an exclusive histologic definition—the presence of a capsule and a growth pattern that is different from the surrounding normal parenchyma in an otherwise normal thyroid gland. Strict histologic criteria for an adenoma and its differentiation from hyperplastic thyroid nodules or adenomatous nodules (without a capsule) are, however, difficult to obtain in the frequent presence of goiter or thyroiditis. Therefore, the biologic basis for separating hyperplastic thyroid nodules from true tumors should also depend on their clonality (36). Since many thyroid nodules without a capsule (adenomatous nodules) are monoclonal and since the different characteristics described above are partly overlapping, a mixed functional and molecular definition of true thyroid tumors, as outlined in Figure 20.3, appears more objective, realistic, and consistent. In contrast to solitary nodular thyroid disease, which has a more uniform clinical, pathologic, and molecular picture, TMNG constitutes a mixed group of nodular entities. Thus a combination of hyper-, hypo-, or normally functioning thyroid lesions are usually found within the same thyroid gland. The overall balance of functional properties of individual thyroid nodules within a multinodular goiter ultimately determines the functional status in the individual patient, which may be euthyroidism (normal TSH and free thyroid hormone levels), subclinical hyperthyroidism (low or suppressed TSH and normal free thyroid hormone levels), or overt hyperthyroidism (suppressed TSH and elevated free thyroid hormone levels). The term MNG is applied in the first scenario, while TMNG refers to the latter situations. It is important to emphasize that this functional characterization is not stationary but patients with TMNG usually have a history of long-standing MNG. In general, development of MNG proceeds in two phases: Global activation of thyroid epithelial cell
proliferation (e.g., as the result of iodine deficiency or other goitrogenic stimuli) leading to goiter and a focal increase of thyroid epithelial cell proliferation causing thyroid nodules. The most common stimulus for local (focal) proliferation is a somatic mutation.
proliferation (e.g., as the result of iodine deficiency or other goitrogenic stimuli) leading to goiter and a focal increase of thyroid epithelial cell proliferation causing thyroid nodules. The most common stimulus for local (focal) proliferation is a somatic mutation.
In vivo characteristics of TA include increased iodide uptake, thyroid peroxidase messenger (mRNA), and protein content, as well as increased thyroid hormone secretion (37). Up-regulation of NIS and pendrin contributing to the augmented iodine-trapping characteristics of TA have been reported by other investigators (39). Basal inositol phosphate generation was not found to be increased in toxic thyroid nodules, compatible with a predominant constitutive activation of the cyclic adenosine monophosphate (cyclic AMP)–protein kinase A cascade through TSHR or Gsα protein mutations in TA and TMNG (37). Stimulation of growth has been investigated in TA using the proliferation markers Ki67 and PCNA (37,39). However, the increase in the proliferation index in TA was found to be relatively low (two- to threefold) compared to the surrounding normal thyroid. These data are in agreement with the slow course in TA and TMNG; however, they are difficult to reconcile with the evolution of a macroscopic TA from a single mutated cell (estimated number of 30 cell divisions to yield a nodule volume of ∼1 mL) (2,40) and thus may more likely reflect the end-stage rather than the initiation of disease (see later).
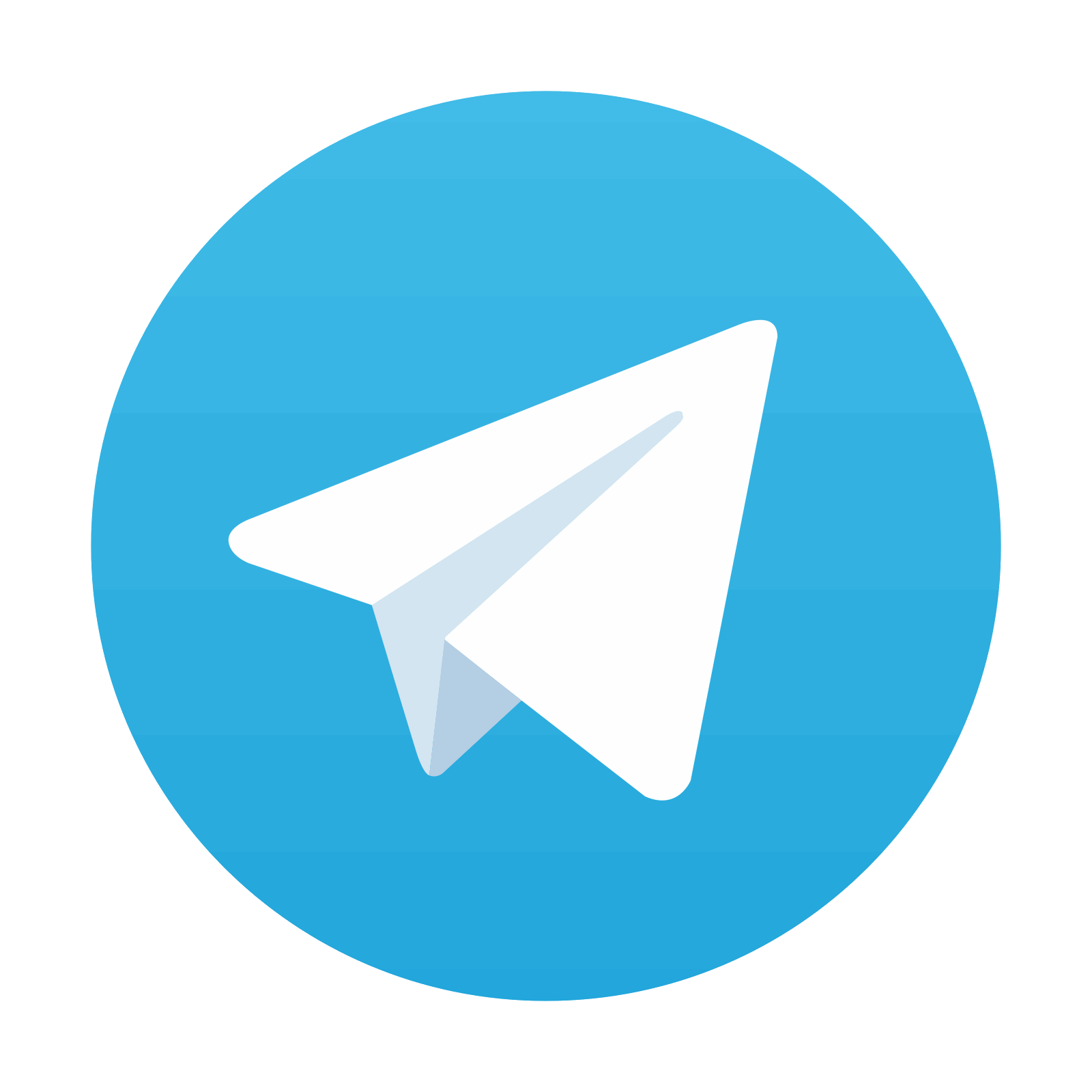
Stay updated, free articles. Join our Telegram channel

Full access? Get Clinical Tree
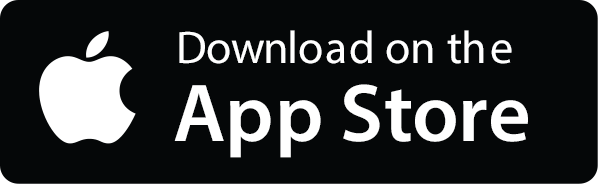
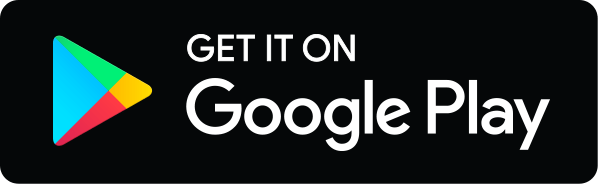