Drug Allergy
Part A
Introduction, Epidemiology, Classification of Adverse Reactions, Immunochemical Basis, Risk Factors, Evaluation of Patients with Suspected Drug Allergy, Patient Management Considerations
Part A
Introduction, Epidemiology, Classification of Adverse Reactions, Immunochemical Basis, Risk Factors, Evaluation of Patients with Suspected Drug Allergy, Patient Management Considerations
Anne Marie Ditto
In the fifth edition of this text, the subject of drug allergy was extensively reviewed (1). Although a reasonably comprehensive overview of this important topic is addressed in the sixth edition (2) and in the current edition, an effort has been made to focus more sharply on clinically applicable information. An even more concise, practical review is published elsewhere (3). Other reviews of drug allergy are also recommended (4). Further, although specific recommendations are suggested regarding drug challenges and desensitization protocols, it is advisable, if possible, for those inexperienced in such matters to consult with physicians who regularly evaluate and manage hypersensitivity phenomena.
Epidemiology
A consequence of the rapid development of new drugs to diagnose and treat human illness has been the increased incidence of adverse reactions to these agents, which may produce additional morbidity and, on occasion, mortality. Their occurrence violates a basic principle of medical practice, primum non nocere (above all, do no harm). It is a sobering fact that adverse drug reactions are responsible for most iatrogenic illnesses. This should serve to remind physicians not to select potent and often unnecessary drugs to treat inconsequential illnesses. Many patients have come to expect drug treatments for the most trivial of symptoms. On the other hand, a physician should not deprive a patient of necessary medication for fear of a reaction. Fortunately, most adverse reactions are not severe, but the predictability of seriousness is usually not possible in the individual case or with the individual drug.
An adverse drug reaction (ADR) may be defined as any undesired and unintended response that occurs at doses of an appropriate drug given for the therapeutic, diagnostic, or prophylactic benefit of the patient. The reaction should appear within a reasonable time after administration of the drug. This definition excludes therapeutic failure, which the patient may perceive as an adverse drug reaction. A drug may be defined as any substance used in diagnosis, therapy, and prophylaxis of disease.
Although the exact incidence of adverse drug reactions is unknown, some estimates of their magnitude are available. Reported estimates of the incidence of adverse drug reactions leading to hospitalization vary and this is complicated by inconsistency with definitions used, with methods used to collect and analyze data, and with some studies measuring prevalence while others measure incidence. One study based on a computerized surveillance system determined that 2%
of hospital admissions were a result of adverse drug reactions (5). A meta-analysis of 33 prospective studies from 1966 to 1996 in the United States showed an incidence of 3.1% to 6.2% of hospital admissions were due to ADRs (6). Other studies from various countries including Switzerland, Australia, and Germany showed that ADRs were the reason for 2% to 6.1% of hospital admissions (7–11). As many as 15% to 30% of medical inpatients experience an adverse drug reaction (12). Drug-attributed deaths occur in 0.01% of surgical inpatients and in 0.14% to 0.17% of medical inpatients (7,10,13–15). Most of these fatalities occurred among patients who were terminally ill (16); in a study of ADR and hospital admissions in Australia, admissions for ADRs increased with patient age (10). Most deaths were due to a small number of drugs that, by their nature, are known to be quite toxic.
of hospital admissions were a result of adverse drug reactions (5). A meta-analysis of 33 prospective studies from 1966 to 1996 in the United States showed an incidence of 3.1% to 6.2% of hospital admissions were due to ADRs (6). Other studies from various countries including Switzerland, Australia, and Germany showed that ADRs were the reason for 2% to 6.1% of hospital admissions (7–11). As many as 15% to 30% of medical inpatients experience an adverse drug reaction (12). Drug-attributed deaths occur in 0.01% of surgical inpatients and in 0.14% to 0.17% of medical inpatients (7,10,13–15). Most of these fatalities occurred among patients who were terminally ill (16); in a study of ADR and hospital admissions in Australia, admissions for ADRs increased with patient age (10). Most deaths were due to a small number of drugs that, by their nature, are known to be quite toxic.
Information regarding outpatient adverse drug events is scant by comparison because most are not reported to pharmaceutical companies and appropriate national registries. Such surveys are complicated by the problem of differentiating between signs and symptoms attributable to the natural disease and those related to its treatment. Adverse drug reactions may mimic virtually every disease, including the disease being treated. The challenge of monitoring adverse drug reactions is further complicated by multiple drug prescribing and the frequent use of nonprescription medications. Despite these limitations, such monitoring did identify the drug-induced skin rash that often follows ampicillin therapy.
Although most drug safety information is obtained from clinical trials before drug approval, premarketing studies are narrow in scope and thus cannot uncover adverse drug reactions in all patient populations. Adverse effects that occur over time or that are less frequent than 1 in 1,000, such as drug hypersensitivity, will not be detected until used by large numbers of patients after drug approval (17).
Thus, postmarketing surveillance is essential to the discovery of unexpected adverse drug effects. However, one estimate is that only 1% of adverse drug reactions are voluntarily reported to pharmaceutical companies and the U.S. Food and Drug Administration (FDA) (18). In an attempt to ensure the timely collection of adverse drug reactions, the FDA introduced a simplified medical products reporting program in 1993, MedWatch (19). Although the FDA had an ADR reporting system in place before MedWatch, it was awkward to use and understandably discouraged health professionals’ participation. Using MedWatch, the reporting individual does not have to prove absolutely an association between the drug and the adverse reaction. When reported, the information becomes part of a large database and can be investigated further. A simple, self-addressed, one-page form is available and can be sent by mail, fax, or electronically (http://www.fda.gov/medwatch). The Web site also has an e-list where one can sign up to receive safety information reports directly by e-mail. Table 17A.1 summarizes how to report ADRs to MedWatch. Voluntary reporting led to the observation that ventricular arrhythmias, such as torsades de pointes, may occur when terfenadine is administered with erythromycin or ketoconazole (20).
Table 17A.1 Reporting Adverse Reactions to MedWatch | ||||||
---|---|---|---|---|---|---|
|
Most ADRs do not have an allergic basis. What follows is a discussion that primarily focuses on those reactions that are, or possibly could be, mediated by immunologic mechanisms.
Allergic drug reactions account for 6% to 10% of all observed adverse drug reactions. It has been suggested that the risk for an allergic reaction is about 1% to 3% for most drugs. It is estimated that about 5% of adults may be allergic to one or more drugs. However, as many as 15% believe themselves to be or have been incorrectly labeled as being allergic to one or more drugs and, therefore, may be denied treatment with an essential medication. At times, it may be imperative to establish the presence or absence of allergy to a drug when its use is necessary and there are no safe alternatives. Although many patients with a history of reacting to a drug could safely receive that drug again, the outcome could be serious if that patient is truly allergic. Hence, a suspicion of drug hypersensitivity must be evaluated carefully.
Classification of Adverse Drug Reactions
Before proceeding with a detailed analysis of drug hypersensitivity, it is appropriate to attempt to place it in perspective with other adverse drug reactions. Physicians should carefully analyze ADRs to determine their nature because this will influence future use. For example, a drug-induced side effect may be corrected by simply reducing the dose. On the other hand, an allergic reaction to a drug may mean that drug cannot be used or may require special considerations before future administration.
Adverse drug reactions may be divided into two major groups: (a) predictable adverse reactions, which are (i) often dose-dependent, (ii) related to the known pharmacologic actions of the drug, (iii) occur in otherwise normal patients, and (iv) account for 80% or more of adverse drug effects; and (b) unpredictable adverse reactions, which are (i) usually dose-independent, (ii) usually unrelated to the drug’s pharmacologic actions, and (iii) often related to the individual’s immunologic responsiveness or, on occasion, to genetic differences in susceptible patients.
Not included in this classification are those reactions that are unrelated to the drug itself but are attributable to events associated with and during its administration. Such events are often mistakenly ascribed to the drug, and the patient is inappropriately denied that agent in the future. Particularly after parenteral administration of a drug, psychophysiologic reactions in the form of hysteria, hyperventilation, or vasovagal response may ensue. Some of these reactions may be manifestations of underlying psychiatric disorders (21). Even anaphylactoid symptoms have been observed in placebo-treated patients (22). Another group of signs and symptoms is considered a coincidental reaction. They are a result of the disease under treatment and may be incorrectly attributed to the drug, for example, the appearance of viral exanthems and even urticaria during the course of a treatment with an antibiotic. Although it may be difficult to characterize a particular drug reaction, a helpful classification is shown in Table 17A.2, followed by a brief description of each.
Table 17A.2 Classification of Adverse Drug Reactions | ||||||||||||
---|---|---|---|---|---|---|---|---|---|---|---|---|
|
Overdosage: Toxicity
The toxic effects of a drug are directly related to the systemic or local concentration of the drug in the body. Such effects are usually predictable on the basis of animal experimentation and may be expected in any patient provided a threshold level has been exceeded. Each drug tends to have its own characteristic toxic effects. Overdosage may result from an excess dose taken accidentally or deliberately. It may be due to accumulation as a result of some abnormality in the patient that interferes with normal metabolism and excretion of the drug. The toxicity of morphine is enhanced in the presence of liver disease (inability to detoxify the drug) or myxedema (depression of metabolic rate). The toxicity of chloramphenicol in infants is due to immaturity of the glucuronide conjugating system, allowing a toxic concentration to accumulate. In the presence of renal failure, drugs such as the aminoglycosides, normally excreted by this route, may accumulate and produce toxic reactions.
Side Effects
Side effects are the most frequent adverse drug reactions. They are therapeutically undesirable, but often unavoidable, pharmacologic actions occurring at usual prescribed drug dosages. A drug frequently has several pharmacologic actions, and only one of those may be the desired therapeutic effect. The others may be considered side effects. The first-generation antihistamines commonly cause adverse central nervous system effects, such as sedation. Their anticholinergic side effects include dry mouth, blurred vision, and urinary retention.
Other side effects may be delayed in expression and include teratogenicity and carcinogenicity. Methotrexate, which has been used in some steroid-dependent asthmatic patients, is teratogenic and should not be used during pregnancy. Immunosuppressive agents can alter host immunity and may predispose the patient to malignancy (23).
Secondary or Indirect Effects
Secondary effects are indirect, but not inevitable, consequences of the drug’s primary pharmacologic action. They may be interpreted as the appearance of another naturally occurring disease rather than being associated with administration of the drug. Some appear to be due to the drug itself, creating an ecologic disturbance and permitting the overgrowth of microorganisms. In the presence of antimicrobial (notably ampicillin, clindamycin, or cephalosporins) exposure, Clostridium difficile can flourish in the gastrointestinal tract in an environment in which there is reduced bacterial competition. Toxins produced by this organism may result in the development of pseudomembranous colitis (24).
Antimicrobial agents may be associated with another group of reactions that may mimic hypersensitivity, but appear to be disease associated. The Jarisch-Herxheimer phenomenon involves the development of fever, chills, headaches, skin rash, edema, lymphadenopathy, and often an exacerbation of preexisting skin lesions. The reaction is believed to result from the release of microbial antigens, endotoxins, or both (25). This has usually followed penicillin treatment of syphilis and leptospirosis, but also has been observed during treatment of parasitic and fungal infections. With continued treatment, the reaction subsides, thus confirming it is not an allergic response. Unfortunately, treatment is often discontinued and the drug blamed for the reaction. Another example would include the high incidence of skin rash in patients with the Epstein-Barr virus treated with ampicillin.
Drug–Drug Interactions
A drug–drug interaction is generally regarded as the modification of the effect of one drug by prior or concomitant administration of another. Fortunately, drug–drug interactions of major clinical consequence are relatively infrequent (26). It is also important to recall that not all drug interactions are harmful, and some may be used to clinical advantage.
As the number of drugs taken concurrently increases, the greater the likelihood of an adverse drug interaction. When an interaction is reported, an average of between four and eight drugs are being taken by the patient. Therefore, the largest risk group are elderly patients, who often receive polypharmacy. The danger of an interaction also escalates when several physicians are treating a patient, each for a separate condition. It is the physician’s responsibility to determine what other medications the patient is taking, even nonprescription drugs.
Several widely prescribed agents used to treat allergic rhinitis and asthma interacted significantly with other drugs. The second-generation antihistamines, terfenadine and astemizole, were metabolized by cytochrome P-450 mixed-function oxidase enzymes. These antihistamines, in combination with drugs that inhibited the P-450 enzyme system, such as the imidazole antifungals ketoconazole and itraconazole or the macrolide antibiotics erythromycin and clarithromycin, resulted in increased concentrations of the antihistamines. This caused potential for prolongation of the QT interval, sometimes producing torsades de pointes or other serious cardiac arrhythmias (19). These antihistamines are no longer available in the United States. Although plasma concentrations of loratadine increased with concomitant administration of ketoconazole, this did not cause prolongation of the QT interval and the risk for torsades de pointes (27).
An excellent review of other adverse drug interactions may be found in a looseleaf publication authored by Hansten and Horn (28).
Intolerance
Intolerance is a characteristic pharmacologic effect of a drug which is quantitatively increased, and often is produced, by an unusually small dose of medication. Most patients develop tinnitus after large doses of salicylates and quinine, but few experience it after a single average dose or a smaller dose than usual. This untoward effect may be genetically determined and appears to be a function of the recipient, or it may occur in individuals lying at the extremes of dose–response curves for pharmacologic effects.
In contrast to intolerance, which implies a quantitatively increased pharmacologic effect occurring among susceptible individuals, idiosyncratic and allergic reactions are qualitatively aberrant and inexplicable in terms of the normal pharmacology of the drug given in usual therapeutic doses.
Idiosyncratic Reactions
Idiosyncrasy is a term used to describe a qualitatively abnormal, unexpected response to a drug, differing from its pharmacologic actions and thus resembling hypersensitivity. However, this reaction does not involve a proven, or even suspected, allergic mechanism.
A familiar example of an idiosyncratic reaction is the hemolytic anemia occurring commonly in African and Mediterranean populations and in 10% to 13% of African American males (sex-linked) exposed to oxidant drugs or their metabolites. About 25% of African American females are carriers, and of these, only one-fifth have a sufficiently severe expression of the deficiency to be clinically important. A more severe form of the deficiency occurs in Caucasian Americans, primarily among people of Mediterranean origin. The erythrocytes of such individuals lack the enzyme glucose-6-phosphate dehydrogenase (G6PD) that is essential for aerobic metabolism of glucose and, consequently, cellular integrity (29). Although the original observations of this phenomenon were among susceptible individuals receiving primaquine, more than 50 drugs are known that induce hemolysis in G6PD-deficient patients. Clinically, the three classes of drugs most important in terms of their hemolytic potential are sulfonamides, nitrofurans, and water-soluble vitamin K analogues. If G6PD deficiency is suspected, simple screening tests dependent on hemoglobin oxidation, dye reduction, or fluorescence generation provide supporting evidence. The study of genetic G6PD deficiency and other genetic defects leading to adverse drug reactions has been termed pharmacogenetics (30).
Allergic Reactions
Allergic drug reactions occur in only a small number of individuals, are unpredictable and quantitatively abnormal, and are unrelated to the pharmacologic action of the drug. Unlike idiosyncrasy, allergic drug reactions are the result of an immune response to a drug following previous exposure to the same drug or to an immunochemically related substance that had resulted in the formation of specific antibodies, of sensitized T lymphocytes, or of both. Ideally, the term drug allergy or hypersensitivity should be restricted to those reactions proved, or more often presumed, to be the result of an immunologic mechanism.
The establishment of an allergic mechanism should be based on the demonstration of specific antibodies, sensitized lymphocytes, or both. This is not often possible for many reactions ascribed to drug allergy. The diagnosis is usually based on clinical observations and, in selected instances, reexposure to the suspected agent under controlled circumstances. Even in the absence of direct immunologic evidence, an allergic drug reaction often is suspected when certain clinical and laboratory criteria are present, as suggested in Table 17A.3. Obviously, none of these is absolutely reliable (31).
Table 17A.3 Clinical Criteria of Allergic Drug Reactions | |
---|---|
|
Immediate reactions occurring within minutes often include manifestations of anaphylaxis. Accelerated reactions taking place after 1 hour to 3 days frequently are manifested as urticaria and angioedema and occasionally as other rashes, especially exanthems with fever. Delayed or late reactions do not appear until 3 days or longer after drug therapy is initiated and commonly include a diverse group of skin rashes, drug fever, and serum sickness–like reactions and, less commonly, hematologic, pulmonary, hepatic, and renal reactions, vasculitis, and a condition resembling lupus erythematosus.
Because clinical criteria are often inadequate, specific immunologic testing is desirable. Until this is accomplished, at best the relationship can be considered only presumptive. With few exceptions, safe, reliable in vivo tests and simple, rapid, predictable in vitro tests for the absolute diagnosis of drug allergy are unavailable. The most conclusive test is cautious readministration of the suspected drug, but usually the risk is not justified.
Pseudoallergic Reactions
Pseudoallergy refers to an immediate generalized reaction involving mast cell mediator release by an immunoglobulin E (IgE)-independent mechanism (32). Although the clinical manifestations often mimic or resemble IgE-mediated events (anaphylaxis), the initiating event does not involve an interaction between the drug or drug metabolites and drug-specific IgE antibodies. A differential point is that these reactions may occur in patients without a previous exposure to these substances.
Such reactions appear to result from nonimmunologic activation of effector pathways. Certain drugs, such as opiates, vancomycin, polymyxin B, and D-tubocurarine, may directly release mediators from mast cells, resulting in urticaria, angioedema, or even a clinical picture resembling anaphylaxis. In general, these reactions can be prevented by pretreatment with
corticosteroids and antihistamines, as outlined for radiographic contrast media (RCM) (33). IgE-mediated allergic reactions, however, cannot.
corticosteroids and antihistamines, as outlined for radiographic contrast media (RCM) (33). IgE-mediated allergic reactions, however, cannot.
Summary
The classification of ADRs presented here must be considered tentative. At times, it may be impossible to place a particular drug reaction under one of these headings. However, the common practice of labeling any ADR as “allergic” should be discouraged.
Immunochemical Basis of Drug Allergy
Drugs as Immunogens
The allergenic potential of drugs is largely dependent on their chemical properties. Increases in molecular size and complexity are associated with an increased ability to elicit an immune response. Hence, high-molecular-weight drugs, such as heterologous antisera, and recombinant proteins (e.g., infliximab and etanercept), streptokinase, L-asparaginase, and insulin, are complete antigens that can induce immune responses and elicit hypersensitivity reactions. Immunogenicity is weak or absent when substances have a molecular weight of less than 4,000 daltons (34).
Most drugs are simple organic chemicals of low molecular weight, usually less than 1,000 daltons. For such low-molecular-weight drugs to become immunogenic, the drug or a drug metabolite must be bound to a macromolecular carrier, often by covalent bonds, for effective antigen processing. The simple chemical (hapten), nonimmunogenic by itself, becomes immunogenic in the presence of the carrier macromolecule and now directs the specificity of the response.
β-Lactam antibiotics are highly reactive with proteins and can directly haptenate carrier macromolecules. However, most drugs are not sufficiently reactive to form a stable immunogenic complex. It is likely that haptens derived from most drugs are reactive metabolites of the parent compound, which then bind to carrier macromolecules to become immunogenic. This requirement for metabolic processing may help to explain the low incidence of drug allergy, the predisposition of certain drugs to cause sensitization as they are prone to form highly reactive metabolites, and the inability of skin testing and other immunologic tests with the unaltered drug to predict or identify the reaction as being allergic in nature.
Another model describing immunogenicity of low-molecular-weight compounds is the pharmacologic interactive (p-i) model in which nonreactive drugs form noncovalent bonds with MHC receptors and directly stimulate T cells (35,36). A third model proposed by Matzinger is the danger model which states that an antigen presenting cell becomes activated when it receives “danger signals” from damaged or stressed cells, thus forming necessary co-stimulatory molecules and cytokines that propagate as well as determine the immunogenic response (37,38).
Penicillin allergy has received the most attention as a model of drug haptenization (39). Unfortunately, relevant drug haptens have not been identified for most allergic drug reactions. Recent studies of human IgE and IgG to sulfonamides have established the N4-sulfonamidoyl determinant to be the major sulfonamide haptenic determinant (40).
It should be noted that an antigen must have multiple combining sites (multivalent) to elicit hypersensitivity reactions. This requirement permits bridging of IgE- and IgG-antibody molecules or antigen receptors on lymphocytes. Conjugation of the free drug or metabolite (hapten) with a macromolecular carrier to form a multivalent hapten-carrier conjugate is necessary to initiate an immune response and elicit a hypersensitivity reaction. The univalent ligand (free drug or metabolite), in large excess, may inhibit the response by competing with the multivalent conjugates for the same receptors. Therefore, the relative concentration of each will determine the frequency, severity, and rate of allergic drug reactions. Also, removal of haptens from carrier molecules by plasma enzymes (dehaptenation) will influence the likelihood of such reactions (41).
Finally, some low-molecular-weight drugs, such as quaternary ammonium muscle relaxants and aminoglycosides, have enough distance between determinants to act as bivalent antigens without requiring conjugation to a carrier (42).
Immunologic Response to Drugs
Drugs often induce an immune response, but only a small number of patients actually experience clinical hypersensitivity reactions. For example, most patients exposed to penicillin and insulin develop demonstrable antibodies; however, in most instances, these do not result in allergic reactions or reduced effectiveness of the drug.
Mechanisms of Drug-induced Immunopathology
An immunologic response to any antigen may be quite diverse and the attendant reactions quite complex. Drugs are no exception and have been associated with all of the immunologic reactions proposed by Coombs and Gell (43) subsequently modified by Janeway (44) and Kay (45). It is likely that more than one mechanism may contribute to a particular reaction, but often one will predominate. Table 17A.4 is an attempt to provide an overview of the immunopathology of allergic drug reactions based on the original Coombs and Gell classification.
Table 17A.4 Immunopathology of Allergic Reactions to Drugs | |||||||||||||||||||||||||||
---|---|---|---|---|---|---|---|---|---|---|---|---|---|---|---|---|---|---|---|---|---|---|---|---|---|---|---|
|
Penicillin alone has been associated with many of these reactions. Anaphylaxis and urticaria following penicillin administration are examples of type I reactions. The hemolytic anemia associated with high-dose penicillin therapy is a type II reaction. A serum sickness–like reaction, now most commonly associated with penicillin treatment, is a type III reaction. Finally, the contact dermatitis that occurred when penicillin was used topically in the past is an example of a type IV reaction.
Risk Factors for Drug Allergy
Several factors have been identified that may influence the induction of drug-specific immune responses and the elicitation of clinical reactions to these agents (46,47) (Table 17A.5).
Table 17A.5 Risk Factors for Drug Allergy | |||||||||||||||||||||||||
---|---|---|---|---|---|---|---|---|---|---|---|---|---|---|---|---|---|---|---|---|---|---|---|---|---|
|
Drug- and Treatment-related Factors
Nature of the Drug
Macromolecular drugs, such as heterologous antisera and insulin, are complex antigens and have the potential to sensitize any patient. As noted earlier, most drugs have molecular weights of less than 1,000 daltons and are not immunogenic by themselves. Immunogenicity is determined by the potential of the drug or, more often, a drug metabolite to form conjugates with carrier proteins.
β-Lactam antibiotics, aspirin and nonsteroidal anti-inflammatory drugs (NSAIDs), and sulfonamides account for 80% of allergic or pseudoallergic reactions.
Drug Exposure
Cutaneous application of a drug is generally considered to be associated with the greatest risk for sensitizing patients (47). In fact, penicillin, sulfonamides, and antihistamines are no longer used topically because of this potential. The adjuvant effect of some intramuscular preparations may increase the risk for sensitization; for example, the incidence of reactions to benzathine penicillin is higher than other penicillin preparations. The intravenous route may be the least likely to sensitize patients.
Once a patient is sensitized, the difference in reaction rates between oral and parenteral drug administration is likely related to the rate of drug administration. Anaphylaxis is less common after oral administration of a drug, although severe reactions have occurred. For other allergic drug reactions, the evidence supporting oral administration is less clear.
The dose and duration of treatment appear to affect the development of a drug-specific immunologic response. In drug-induced lupus erythematosus (DIL), the dose and duration of hydralazine therapy are important factors. Penicillin-induced hemolytic anemia follows high, sustained levels of drug therapy.
There is currently evidence that the frequency of drug administration affects the likelihood of sensitization (48). Thus, frequent courses of treatment are more likely to elicit an allergic reaction as is interrupted therapy. The longer the intervals between therapy, the less likely there will be an allergic reaction.
Patient-related Factors
Age and Gender
There is a general impression that children are less likely to become sensitized to drugs than adults. However, serious allergic drug reactions do occur in children. Some confusion may arise in that the rash associated with a viral illness in children may incorrectly be ascribed to an antibiotic being administered as
treatment. Women are reported to have a higher incidence of ADRs than men (49,50).
treatment. Women are reported to have a higher incidence of ADRs than men (49,50).
Genetic Factors
Allergic drug reactions occur in only a small percentage of individuals treated with a given drug. It is likely that many factors, both genetic and environmental, are involved in determining which individuals in a large random population will develop an allergic reaction to a given drug.
Patients with a history of allergic rhinitis, asthma, or atopic dermatitis (the atopic constitution) are not at increased risk for being sensitized to drugs compared with the general population (47). However, it does appear that atopic patients are more likely to develop pseudoallergic reactions, especially to RCM (51).
The rate of metabolism of a drug may influence the prevalence of sensitization. Individuals who are genetically slow acetylators are more likely to develop DIL associated with the administration of hydralazine and procainamide (52,53). Adverse reactions to sulfonamides may be more severe among slow acetylators (54).
Specific human leukocyte antigen (HLA) genes have been associated with the risk for drug allergy. The susceptibility for drug-induced nephropathy in patients with rheumatoid arthritis treated with gold salts or penicillamine is associated with the HLA-DRw3 and HLA-B8 phenotypes respectively (55). In addition, specific HLA genes have been associated with hydralazine-induced lupus erythematosus, levamisole-induced agranulocytosis, and sulfonamide-induced toxic epidermal necrolysis (TEN) (56). In a Han Chinese population, studies have shown a strong association between carbamazapime-induced SJS and HLA-B*1502 (57) as well as a strong association between HLA-B*5801 and severe cutaneous drug reactions (SJS and TEN) due to allopurinol (58). An association between HLA-B*5201 and hypersensitivity to abacavir, a potent reverse transriptase inhibitor, was shown in an HIV population in Western Australia (59). This has been confirmed in several other cohort studies (60–62); however, this association has not been found in black populations (61).
The possibility of familial drug allergy has been reported recently (56). Among adolescents whose parents had sustained an allergic reaction to antibiotics, 25.6% experienced an allergic reaction to an antimicrobial agent, whereas only 1.7% reacted when their parents tolerated antibiotics without an allergic reaction.
Prior Drug Reactions
Undoubtedly, the most important risk factor is a history of a prior hypersensitivity reaction to a drug being considered for treatment or one that may be immunochemically similar. However, drug hypersensitivity may not persist indefinitely. It is well established that, after an allergic reaction to penicillin, the half-life of antipenicilloyl IgE antibodies in serum ranges from 55 days to an indeterminate, long interval in excess of 2,000 days (47). Ten years after an immediate-type reaction to penicillin, only about 20% of individuals are still skin test positive.
There may be cross-sensitization between drugs. The likelihood of cross-reactivity among the various sulfonamide groups (antibacterials, sulfonylureas, diuretics) is an issue that has not been resolved. There is little supporting evidence in the medical literature that cross-sensitization is a significant problem. Patients who have demonstrated drug hypersensitivity in the past appear to have an increased tendency to develop sensitivity to new drugs. Penicillin-allergic patients have about a 10-fold increased risk for an allergic reaction to non–β-lactam antimicrobial drugs (63,64). The reactions were not restricted to immediate-type hypersensitivity. Fifty-seven percent reacted to a sulfonamide. With the exception of the aminoglycosides, reaction rates were much higher than expected in all other antibiotic classes, including erythromycin. Among children with multiple antibiotic sensitivities by history, 26% had
positive penicillin skin tests (65). These observations suggest that such patients are prone to react to haptenating drugs during an infection (66), possibly due to the “danger” signals induced by infection. Obviously, such patients present difficult clinical management problems.
positive penicillin skin tests (65). These observations suggest that such patients are prone to react to haptenating drugs during an infection (66), possibly due to the “danger” signals induced by infection. Obviously, such patients present difficult clinical management problems.
Concurrent Medical Illness
Although atopy does not predispose to the development of IgE-mediated drug hypersensitivity, it appears to be a risk factor for more severe reactions once sensitivity has occurred, especially in asthmatic patients (46,47). Children with cystic fibrosis are more likely to experience allergic drug reactions, especially during drug desensitization (67). Maculopapular rashes following the administration of ampicillin occur more frequently during Epstein-Barr viral infections and among patients with lymphatic leukemia (68).
Immune deficiency is associated with an increased frequency of adverse drug reactions, many of which appear to be allergic in nature. Patients who are immunosuppressed may become deficient in regulatory T lymphocytes that control IgE-antibody synthesis.
In recent years, much attention has been given to adverse drug reactions, in particular hypersensitivity, which occur with a much higher frequency among human immunodeficiency virus (HIV)-infected patients than among patients who are HIV seronegative (69,70). A retrospective study comparing Pneumocystis carinii pneumonia (PCP) in patients with acquired immunodeficiency syndrome (AIDS) to a similar pneumonia in patients with other underlying immunosuppressive conditions reported adverse reactions to trimethoprim-sulfamethoxazole (TMP-SMX) in 65% of AIDS patients compared with 12% of patients with other immunosuppressive diseases, suggesting the abnormality may be due to the HIV infection (71). Slow acetylator phenotype is a risk factor for TMP-SMX in HIV-negative patients but not HIV-positive patients (72–74). TMP-SMX has been associated with rash, fever, and hematologic disturbances and, less frequently, with more severe reactions such as Stevens-Johnson syndrome (SJS), toxic epidermal necrolysis, and anaphylactic reactions. Also, pentamidine, antituberculosis regimens containing isoniazid and rifampin, amoxicillin-clavulanate, and clindamycin have been associated with an increased incidence of adverse drug reactions, some of which may involve an allergic mechanism. It also appears that progression of HIV disease to a more advanced stage confers an increased risk for hypersensitivity reactions (69).
Concurrent Medical Therapy
Some medications may alter the risk and severity of reactions to drugs. Patients treated with β-adrenergic blocking agents, even timolol maleate ophthalmic solution, may be more susceptible to, and prove to be more refractory to, treatment of drug-induced anaphylaxis, requiring greater fluid resucitation and possibly more epinephrine to overcome the β blockade (75).
Clinical Classification of Allergic Reactions to Drugs
A useful classification is based primarily on the clinical presentation or manifestations of such reactions. The presumption of allergy is based on clinical criteria cited earlier (Table 17A.3). Table 17A.6 provides an overview of a clinical classification based on organ systems involved; namely, generalized multisystem involvement and predominantly organ-specific responses.
Table 17A.6 Clinical Classification of Allergic Reactions to Drugs | ||||||||||||||||||||||||||||||||||||||
---|---|---|---|---|---|---|---|---|---|---|---|---|---|---|---|---|---|---|---|---|---|---|---|---|---|---|---|---|---|---|---|---|---|---|---|---|---|---|
|
What follows is a brief discussion of each of these clinical entities, including a list of most commonly implicated drugs. Detailed lists of implicated drugs appear in periodic literature reviews (76,77).
Generalized or Multisystem Involvement
Immediate Generalized Reactions
The acute systemic reactions are among the most urgent of drug-related events. Greenberger has used the term immediate generalized reactions to underscore the fact that many are not IgE-mediated (78). Drug-induced anaphylaxis should be reserved for a systemic reaction proved to be IgE-mediated. Drug-induced anaphylactoid reactions are clinically indistinguishable from anaphylaxis but occur through IgE-independent mechanisms. Both ultimately result in the release of potent vasoactive and inflammatory mediators from mast cells and basophils.
In a series of 32,812 continuously monitored patients, such reactions occurred in 12 patients (0.04%), and there were 2 deaths (79). Because anaphylaxis is more likely to be reported when a fatality occurs, its prevalence may be underestimated. Drug-induced anaphylaxis does not appear to confer increased risk for such generalized reactions to allergens from other sources (80).
Most reactions occur within 30 minutes, and death may ensue within minutes. In a retrospective study by Pumphrey in the United Kingdom investigating fatalities associated with anaphylaxis, more than one-half of the fatal reactions were iatrogenic. The majority of these reactions were due to intravenous (IV) medications and took 5 minutes or less from the time of administration to the time of arrest (81). Anaphylaxis occurs most commonly after parenteral administration, but it has also followed oral, percutaneous, and respiratory exposure. Symptoms usually subside rapidly with
appropriate treatment, but may last 24 hours or longer, and recurrent symptoms may appear several hours after apparent resolution of the reaction. As a rule, the severity of the reaction decreases with increasing time between exposure to the drug and onset of symptoms. Death is usually due to cardiovascular collapse or respiratory obstruction, especially laryngeal or upper airway edema. Although most reactions do not terminate fatally, the potential for such must be borne in mind, and the attending physician must respond immediately with appropriate treatment.
appropriate treatment, but may last 24 hours or longer, and recurrent symptoms may appear several hours after apparent resolution of the reaction. As a rule, the severity of the reaction decreases with increasing time between exposure to the drug and onset of symptoms. Death is usually due to cardiovascular collapse or respiratory obstruction, especially laryngeal or upper airway edema. Although most reactions do not terminate fatally, the potential for such must be borne in mind, and the attending physician must respond immediately with appropriate treatment.
Table 17A.7 summarizes agents most frequently associated with immediate generalized reactions. In some situations, drugs, such as general anesthetic agents and vancomycin, which are primarily direct mast cell mediator releasers, can produce an IgE-mediated reaction (42,82). This distinction has clinical relevance in that IgE-independent reactions may be prevented or modified by pretreatment with corticosteroids and antihistamines, whereas such protection from drug-induced IgE-mediated reactions is less likely. In the latter situation, when the drug is medically necessary, desensitization is an option.
Table 17A.7 Drugs Implicated in Immediate Generalized Reactions | |||||||||||||||||||||||||||
---|---|---|---|---|---|---|---|---|---|---|---|---|---|---|---|---|---|---|---|---|---|---|---|---|---|---|---|
|
The β-lactam antibiotics, notably penicillin, are by far the most common causes of drug-induced anaphylaxis. Essentially all β-lactam anaphylactic reactions are IgE-mediated. Immediate generalized reactions to other antibiotics occur but are relatively uncommon. Recently, anaphylactoid reactions have been reported after the administration of ciprofloxacin and norfloxacin (83).
Cancer chemotherapeutic agents have been associated with hypersensitivity reactions, most commonly type I immediate generalized reactions (84). L-Asparaginase has the highest risk for such reactions. Serious anaphylactic reactions with respiratory distress and hypotension occur in about 10% of patients treated. It is likely that most of these reactions are IgE-mediated. However, skin testing appears to be of no value in predicting a reaction because there are both false-positive and false-negative results. Therefore, one must be prepared to treat anaphylaxis with each dose. For those reacting to L-asparaginase derived from Escherichia coli, one derived from Erwinia chyoanthermia (a plant pathogen) or a modified asparaginase (pegaspargase) may be a clinically effective substitute. Cisplatin and carboplatin are second only to L-asparaginase in producing such reactions. Skin testing with these agents appears to have predictive value, and desensitization has been successful when these drugs are medically necessary (85). The initial use of paclitaxel (Taxol) to treat ovarian and breast cancer was associated with a 10% risk for anaphylactoid reactions. However, with premedication and lengthening of the infusion time, the risk is significantly reduced (86). All other antitumor drugs, except altretamine, the nitrosoureas, and dactinomycin, have occasionally been associated with hypersensitivity reactions (84). Some appear to be IgE mediated, but most are probably IgE independent.
Anaphylactic and anaphylactoid reactions occurring during the perioperative period have received increased attention. The evaluation and detection of these reactions is complicated by the use of multiple medications and the fact that patients are often unconscious and draped, which may mask the early signs and symptoms of an immediate generalized reaction (87). During anesthesia, the only feature observed may be cardiovascular collapse (88) or airway obstruction. Cyanosis due to oxygen desaturation may be noted. One large multicenter study indicated that 70% of cases were due to muscle relaxants and 12% were due to latex (89). Other agents, such as intravenous induction drugs, plasma volume expanders (dextran), opioid analgesics and antibiotics, also require consideration. With the increased use of cardiopulmonary bypass surgery, the incidence of protamine-induced immediate life-threatening reactions has risen (90). Anaphylaxis to ethylene oxide-sterilized devices has been described; hence, such devices used during anesthesia could potentially cause anaphylaxis (91).
Psyllium seed is an active ingredient of several bulk laxatives, and has been responsible for asthma following inhalation and anaphylaxis after ingestion, particularly in atopic subjects (92). Anaphylactoid reactions following IV fluorescein may be modified by pretreatment with corticosteroids and antihistamines (93). Of patients reacting to iron-dextran, 0.6% had a life-threatening anaphylactoid reaction (94). Anaphylactoid reactions may also be caused by blood and blood products through the activation of complement and the production of anaphylatoxins. Adverse reactions to monoclonal antibodies include immediate generalized manifestations, but the mechanism for such remains unclear (95). Most appear not to be IgE mediated (96) and protocols including rapid desensitization have been established for managing these reactions (97,98).
If one surveys the medical literature, one will find that virtually all drugs, including corticosteroids, tetracycline, cromolyn, erythromycin, and cimetidine, have been implicated in such immediate generalized reactions. However, these infrequent reports should not be a reason to withhold essential medication.
Serum Sickness and Serum Sickness–like Reactions
Serum sickness results from the administration of heterologous (often equine) antisera and is the human equivalent of immune complex-mediated serum sickness observed in experimental animals (99). A serum sickness–like illness has been attributed to a number of nonprotein drugs, notably the β-lactam antibiotics. These reactions are usually self-limited and the outcome favorable, but H1 blockers and prednisone may be needed.
With effective immunization procedures, antimicrobial therapy, and the availability of human antitoxins, the incidence of serum sickness has declined. Currently, heterologous antisera are still used to counteract potent toxins such as snake venoms, black widow and brown recluse spider venom, botulism, and gas gangrene toxins as well as to treat diphtheria and rabies. Equine and rabbit antisera, used as antilymphocyte or antithymocyte globulins and as monoclonal antibodies for immunomodulation and cancer treatment, may cause serum sickness (100). Serum sickness has also been reported in patients receiving streptokinase (101).
β-Lactam antibiotics are considered to be the most common nonserum causes of serum sickness–like reactions (102). One literature review did not support this assertion (103). In fact, such reactions appear to be quite infrequent, with an incidence of 1.8 per 100,000 prescriptions of cefaclor and 1 per 10 million for amoxicillin and cephalexin (104). Other drugs occasionally incriminated include ciprofloxacin, metronidazole, streptomycin, sulfonamides, allopurinol, carbamazepine, hydantoins, methimazole, phenylbutazone, propanolol, and thiouracil. It should be noted that the criteria for diagnosis might not be uniform for each drug.
The onset of serum sickness typically begins 6 to 21 days after administration of the causative agent. The
latent period reflects the time required for the production of antibodies. The onset of symptoms coincides with the development of immune complexes. Among previously immunized individuals, the reaction may begin within 2 to 4 days following administration of the inciting agent. The manifestations include fever and malaise, skin eruptions, joint symptoms, and lymphadenopathy.
latent period reflects the time required for the production of antibodies. The onset of symptoms coincides with the development of immune complexes. Among previously immunized individuals, the reaction may begin within 2 to 4 days following administration of the inciting agent. The manifestations include fever and malaise, skin eruptions, joint symptoms, and lymphadenopathy.
There is no laboratory finding specific for the diagnosis of serum sickness or serum sickness–like reactions. Laboratory abnormalities may be helpful, if present. The erythrocyte sedimentation rate may be elevated, although it has been noted to be normal or low (102). There may be a transient leukopenia or leukocytosis during the acute phase (79,105.) Plasmacytosis may occasionally be present; in fact, serum sickness is one of the few illnesses in which plasma cells may be seen in the peripheral blood (106). The urinalysis may reveal slight proteinuria, hyaline casts, hemoglobinuria, and microscopic hematuria. However, nitrogen retention is rare. Transaminases and serum creatinine may be transiently elevated (100).
Serum concentrations of C3, C4, and total hemolytic complement are depressed, providing some evidence that an immune complex mechanism is operative. These may rapidly return to normal. Immune complex and elevated plasma concentrations of C3a and C5a anaphylatoxins have been documented (107).
The prognosis for complete recovery is excellent. The symptoms may be mild, lasting only a few days, or quite severe, persisting for several weeks or longer.
Antihistamines control urticaria. If symptoms are severe, corticosteroids (e.g., prednisone, 40 mg/day for 1 week and then taper) are indicated. However, corticosteroids do not prevent serum sickness, as noted in patients receiving antithymocyte globulin (100). Skin testing with foreign antisera is routinely performed to avoid anaphylaxis with future use of foreign serum.
Drug Fever
Fever is a well-known drug hypersensitivity reaction. An immunologic mechanism is often suspected. Fever may be the sole manifestation of drug hypersensitivity and is particularly perplexing in a clinical situation in which a patient is being treated for an infection.
The height of the temperature does not distinguish drug fever, and there does not appear to be any fever pattern typical of this entity. Although a distinct disparity between the recorded febrile response and the relative well-being of the patient has been emphasized, clearly such individuals may be quite ill with high fever and shaking chills. Drug fever may be the sole manifestation of a drug allergy but is commonly seen with other signs of drug hypersensitivity such as rash, elevated liver enzymes, and eosinophils.
Laboratory studies usually reveal leukocytosis with a shift to the left, thus mimicking an infectious process. Mild eosinophilia may be present. An elevated erythrocyte sedimentation rate and abnormal liver function tests are present in most cases.
The most consistent feature of drug fever is prompt defervescence, usually within 48 to 72 hours after withdrawal of the offending agent. Subsequent readministration of the drug produces fever, and occasionally chills, within a matter of hours.
In general, the diagnosis of drug fever is one of exclusion after eliminating other potential causes of the febrile reaction. Prompt recognition of drug fever is essential. If not appreciated, patients may be subjected to multiple diagnostic procedures and inappropriate treatment. Of greater concern is the possibility that the reaction may become more generalized with resultant tissue damage. Autopsies on patients who died during drug fever show arteritis and focal necrosis in many organs, such as myocardium, lung, and liver.
Drug-induced Autoimmunity
Drug-induced Lupus Erythematosus
DIL is the most familiar drug-induced autoimmune disease, in part because systemic lupus erythematosus (SLE) remains the prototype of autoimmunity. DIL is termed autoimmune because of its association with the development of antinuclear antibodies (ANAs). However, these same autoantibodies are found frequently in the absence of frank disease. An excellent review of drug-induced autoimmunity appears elsewhere (108) as well as a comprehensive review of the medications implicated (109).
Convincing evidence for DIL first appeared in 1953 after the introduction of hydralazine for treatment of hypertension (110) although it was first described in 1945 associated with sulfadiazine (111). Procainamide-induced lupus was first reported in 1962 and is now the most common cause of DIL in the United States (112). These drugs have also been the best studied. Other agents for which there has been definite proof of an association include isoniazid, chlorpromazine, methyldopa, quinidine, and minocycline. Another group of drugs probably associated with the syndrome includes many anticonvulsants, β blockers, antithyroid drugs, penicillamine, sulfasalazine, and lithium. There have been case reports of DIL associated with monoclonal antibodies such as inflixamab and etanercept (113,114) and an ANA-negative, anti-histone-positive DIL has been described with lisinopril (115). There are case reports linking statins such as lovastatin, fluvastatin, and atorvastatin with DIL but with varying clinical manifestations including pneumonitis, and cutaneous manifestations (116).
The incidence of DIL is not precisely known. In a recent survey of patients with lupus erythematosus seen in a private practice, 3% had DIL (117). The
estimated incidence is 15,000 to 20,000 cases per year (118). In contrast to SLE, patients with DIL tend to be older and males and females are equally affected (119). Patients with idiopathic SLE do not appear to be at increased risk from drugs implicated in DIL (120). Identified risk factors for developing DIL include HLA-DR4 (121), HLA-DR*0301 (122), slow acetlylator status (123), and complement C4 null allele (124).
estimated incidence is 15,000 to 20,000 cases per year (118). In contrast to SLE, patients with DIL tend to be older and males and females are equally affected (119). Patients with idiopathic SLE do not appear to be at increased risk from drugs implicated in DIL (120). Identified risk factors for developing DIL include HLA-DR4 (121), HLA-DR*0301 (122), slow acetlylator status (123), and complement C4 null allele (124).
Fever, malaise, arthralgias, myalgias, pleurisy, and slight weight loss may appear acutely in a patient receiving an implicated drug. Pleuropericardial manifestations, such as pleurisy, pleural effusions, pulmonary infiltrates, pericarditis, and pericardial effusions, are more often seen in patients taking procainamide. Unlike idiopathic SLE, the classic butterfly malar rash, discoid lesions, oral mucosal ulcers, Raynaud phenomenon, alopecia, and renal and central nervous system disease are unusual in DIL. Glomerulonephritis has occasionally been reported in hydralazine-induced lupus. As a rule, DIL is a milder disease than idiopathic SLE. Because many clinical features are nonspecific, the presence of ANAs (homogenous pattern) or anti-histone antibodies is essential in the diagnosis of drug-induced disease.
Clinical symptoms usually do not appear for many months after institution of drug treatment. Clinical features of DIL usually subside within days to weeks after the offending drug is discontinued. In an occasional patient, the symptoms may persist or recur over several months before disappearing. ANAs often disappear in a few weeks to months but may persist for 1 year or longer. Mild symptoms may be managed with NSAIDs; more severe disease may require corticosteroid treatment.
If no satisfactory alternative drug is available and treatment is essential, the minimum effective dose of the drug and corticosteroids may be given simultaneously with caution and careful observation. With respect to procainamide, DIL can be prevented by giving N-acetylprocainamide, the major acetylated metabolite of procainamide. In fact, remission of procainamide-induced lupus has occurred when patients were switched to N-acetylprocainamide therapy (125,126). Finally, there are no data to suggest that the presence of ANAs necessitates discontinuance of the drug in asymptomatic patients. The low probability of clinical symptoms in seroreactors and the fact that major organs are usually spared in DIL support this recommendation (127).
Other Drug-induced Autoimmune Disorders
In addition to DIL, D-penicillamine has been associated with several other autoimmune syndromes, such as myasthenia gravis, polymyositis and dermatomyositis, pemphigus and pemphigoid, membranous glomerulonephritis, Goodpasture syndrome, and immune cytopenias (128). It has been suggested that by binding to cell membranes as a hapten, penicillamine could induce an autologous T-cell reaction, B-cell proliferation, autoantibodies, and autoimmune disorders (129).
Hypersensitivity Vasculitis
Vasculitis is a condition that is characterized by inflammation and necrosis of blood vessels. Organs or systems with a rich supply of blood vessels are most often involved. Thus, the skin is often involved in vasculitic syndromes. In the systemic necrotizing vasculitis group (polyarteritis nodosa, allergic granulomatosis of Churg-Strauss) and granulomatous vasculitides (Wegener’s granulomatosis, lymphomatoid granulomatosis, giant cell arteritides), cutaneous involvement is not as common a presenting feature as seen in the hypersensitivity vasculitides (HSV). Also, drugs do not appear to be implicated in the systemic necrotizing and granulomatous vasculitic syndromes.
Drugs appear to be responsible for or associated with a significant number of cases of HSV (130). These may occur at any age, but the average age of onset is in the fifth decade (131). The older patient is more likely to be taking medications that have been associated with this syndrome, for example, diuretics and cardiac drugs. Other frequently implicated agents include penicillin, sulfonamides, thiouracils, hydantoins, iodides, and allopurinol. Allopurinol administration, particularly in association with renal compromise and concomitant thiazide therapy, has produced a vasculitic syndrome manifested by fever, malaise, rash, hepatocellular injury, renal failure, leukocytosis, and eosinophilia. The mortality rate approaches 25% (132). However, in many cases of HSV, no cause is ever identified. Fortunately, idiopathic cases tend to be self-limited.
The most common clinical feature of HSV is palpable purpura, and the skin may be the only site where vasculitis is recognized. The lesions occur in recurrent crops of varying size and number and are usually distributed in a symmetric pattern on the lower extremities and sacral area. Fever, malaise, myalgia, and anorexia may accompany the appearance of skin lesions. Usually, only cutaneous involvement occurs in drug-induced HSV, but glomerulonephritis, arthralgias or arthritis, abdominal pain and gastrointestinal bleeding, pulmonary infiltrates, and peripheral neuropathy are occasionally present.
The diagnosis of HSV is established by skin biopsy of a lesion demonstrating characteristic neutrophilic infiltrate of the blood vessel wall terminating in necrosis, leukocytoclasis (nuclear dust or fragmentation of nuclei), fibrinoid changes, and extravasation of erythrocytes. This inflammation involves small blood vessels, predominantly postcapillary venules. Recent studies indicate that in drug-induced vasculitis, multispecific antinuclear cytoplasmic antibody (ANCA) (ANCA positive to several neutrophil antigens) is commonly
found. This is distinguished from ANCA to only one neutrophil antigen as is seen with idiopathic vasculitis and may serve to distinguish between the two (133).
found. This is distinguished from ANCA to only one neutrophil antigen as is seen with idiopathic vasculitis and may serve to distinguish between the two (133).
When a patient presents with palpable purpura and has started a drug within the previous few months, consideration should be given to stopping that agent. Generally, the prognosis for HSV is excellent, and elimination of the offending agent, if one exists, usually suffices for therapy. For a minority of patients who have persistent lesions or significant involvement of other organ systems, corticosteroids are indicated.
Predominantly Organ-specific Reactions
Dermatologic Manifestations
Cutaneous eruptions are the most frequent manifestations of adverse drug reactions and occur in 2% to 3% of hospitalized inpatients (134). The offending drug could be identified in most cases and in one study was confirmed by drug challenges in 62% of patients (135). Frequently implicated agents include β-lactam antibiotics (especially ampicillin and amoxicillin), sulfonamides (especially TMP-SMX), NSAIDs, anticonvulsants, and central nervous system depressants (136).
Drug eruptions are most often exanthematous or morbilliform in nature. Most are of mild or moderate severity, often fade within a few days, and pose no threat to life or subsequent health. On rare occasions, such drug eruptions may be severe or even life-threatening, for example, SJS and toxic epidermal necrolysis. Some more typical features of a drug-induced eruption include an acute onset within 1 to 2 weeks after drug exposure, symmetric distribution, predominant truncal involvement, brilliant coloration, and pruritus. Features that suggest that a reaction is serious include the presence of urticaria, blisters, mucosal involvement, facial edema, ulcerations, palpable purpura, fever, lymphadenopathy, and eosinophilia (137). The presence of these usually necessitates prompt withdrawal of the offending drug.
Table 17A.8 provides a list of recognizable cutaneous eruptions frequently induced by drugs, presumably on an immunologic basis.
Table 17A.8 Drug-induced Cutaneous Manifestations | ||||||||||||||||||
---|---|---|---|---|---|---|---|---|---|---|---|---|---|---|---|---|---|---|
|
Exanthematous or Morbilliform Eruptions
Exanthematous or morbilliform eruptions are the most common drug-induced eruptions and may be difficult to distinguish from viral exanthems. The rash may be predominantly erythematous, maculopapular, or morbilliform (measles-like), and often begins on the trunk or in areas of pressure, for example, the backs of bedridden patients. Pruritus is variable or minimal. Occasionally, pruritus may be an early symptom, preceding the development of cutaneous manifestations. Gold salts and sulfonamides have been associated with pruritus as an isolated feature. This rarely progresses to overt exfoliation, although this is possible (138
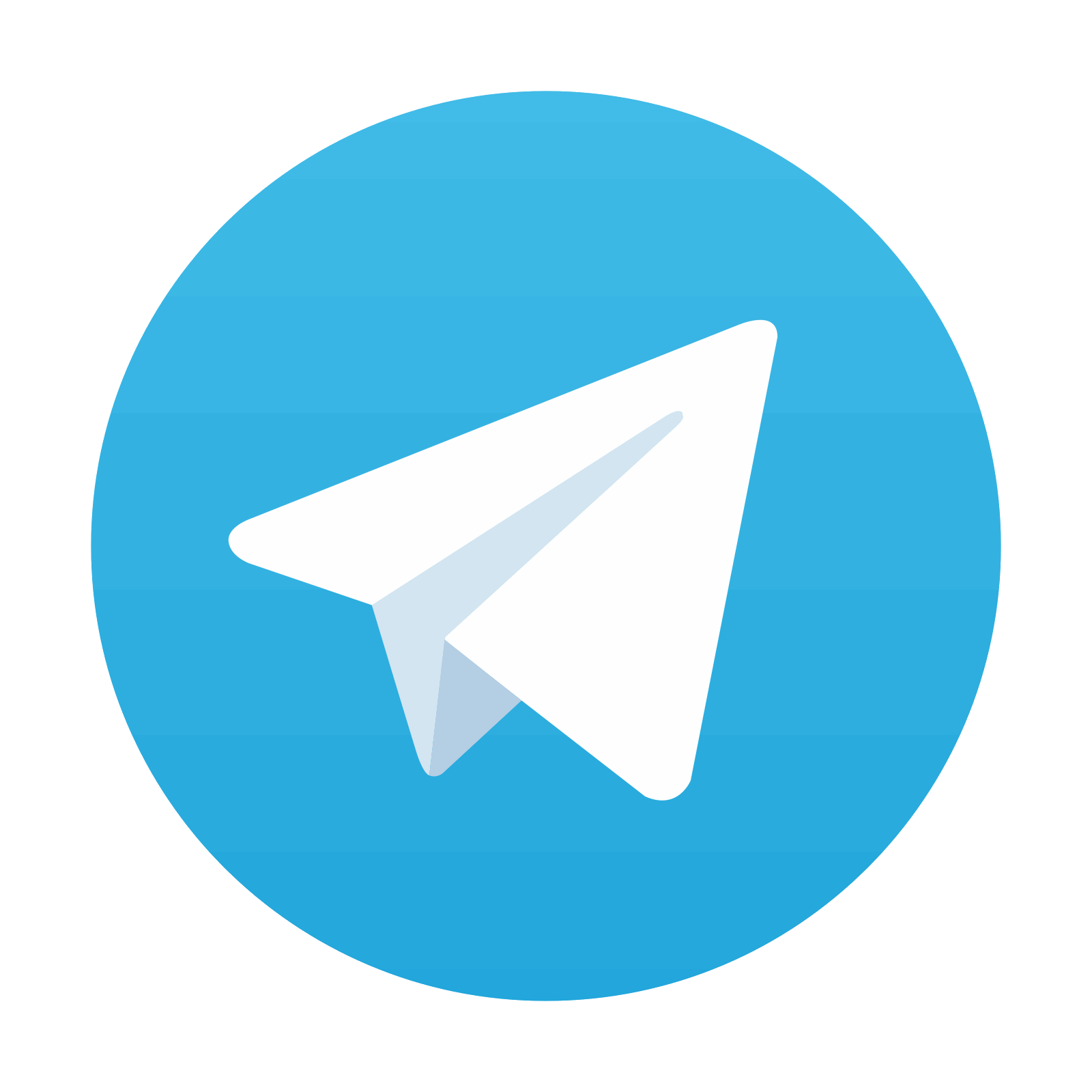
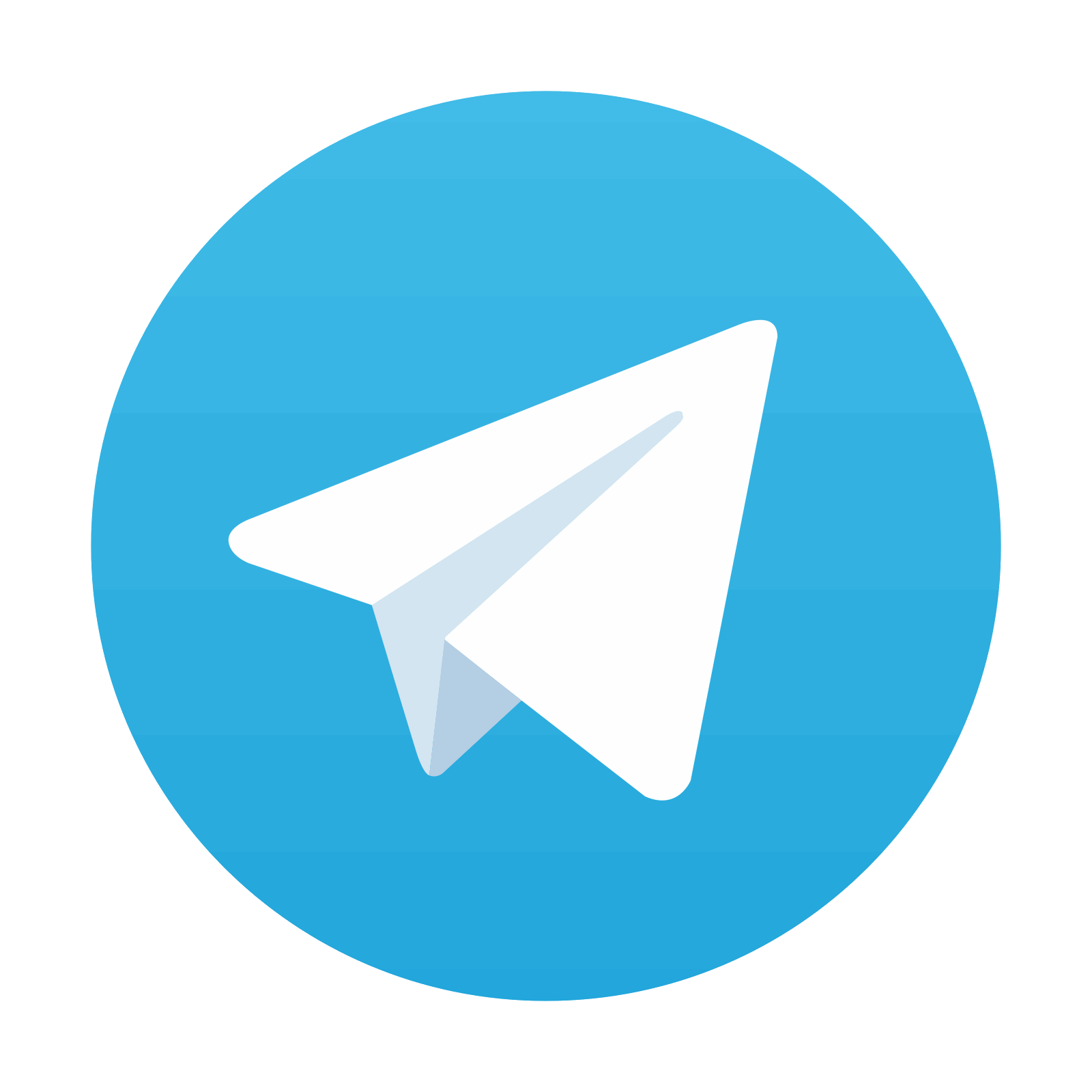
Stay updated, free articles. Join our Telegram channel

Full access? Get Clinical Tree
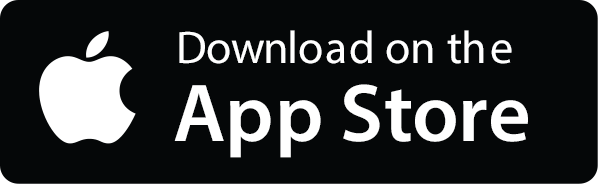
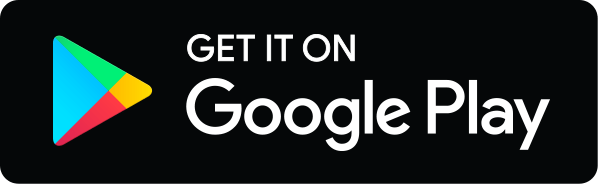
