Chapter Outline
Sources of radioisotopes 352
Radiation protection 352
Apparatus for measuring radioactivity in vitro 353
Apparatus for measuring radioactivity in vivo 353
Measurement of radioactivity with a scintillation counter 353
Blood volume 354
Measurement of blood volume 355
Red cell volume 355
Plasma volume 356
Simultaneous measurement of red cell volume and plasma volume 357
Expression of results of blood volume estimations 357
Splenic red cell volume 358
Ferrokinetics 358
Estimation of the lifespan of red cells in vivo 358
Radioactive chromium ( 51 Cr) method 358
Compatibility testing 362
Visualisation of the spleen by scintillation scanning 362
Spleen function 362
Leucocyte imaging 363
Miscellaneous imaging 363
Measurement of blood loss from the gastrointestinal tract 363
Measurement of platelet lifespan 363
Radioactive isotopes must be distinguished from nonradioactive isotopes of the same element. The radioactive forms are usually referred to as radionuclides or radioisotopes. These terms are interchangeable and in this chapter, the latter term is used.
Methods using radioisotopes have an important place in haematological diagnosis. Tests that may be undertaken in haematology departments include total blood volume (TBV), red cell survival studies and, occasionally, ferrokinetic studies.
Other investigations that may have haematological interest are more likely to be referred to a department of medical physics or nuclear medicine. Even when the tests are not carried out directly in the haematology department, it is essential for the haematologist to understand their principles and limitations and to be able to interpret the results in clinical terms. Various textbooks , provide more complete accounts of the theory and practice of nuclear medicine techniques, as does a monograph on radioisotopes in haematology by Lewis and Bayly.
The main properties of the radioisotopes useful in diagnostic haematology are shown in Table 17-1 . The units used to express radioactivity and the effects of radiation on the body are given in the previous edition of this book. Anyone handling radioisotopes must be aware of the potential radiation hazard. It is also important to be aware of the potential biohazard of handling blood products and administering them to patients (see Chapter 24 ).
Element Isotope | Half-Life (T ½ ) | Energies (MeV) | Pharmaceutical | Application | Activities (MBq) | Radiation Dose (mSv) | Chest X-ray Equivalence * |
---|---|---|---|---|---|---|---|
51 Cr | 27.8 days | 0.320 | Sodium chromate | Red cell volume Red cell lifespan Gastrointestinal bleeding Spleen scan Spleen pool | 0.8 2 4 4 4 | 0.3 0.6 1 1 1 | 15 30 50 50 50 |
125 I | 60 days | 0.035 | Iodinated human serum albumin | Plasma volume | 0.2 | 0.06 † | 3 |
111 In | 2.81 days | 0.247 0.173 | Indium chloride → oxine/tropolone | Red cell volume Spleen scan Platelet lifespan | 2 5 4 | 1 2 2 | 50 100 100 |
99m Tc | 6 h | 0.141 | Pertechnetate | Red cell volume Spleen scan Spleen pool | 2 100 100 | 0.02 1 1 | 1 50 |
* The number of chest X-rays that would expose the subject to the same amount of radiation as the radioisotopes that are listed. A simple chest X-ray has a radiation dose of 0.02 mSv, but for some radiological investigations it is far greater. For example, for intravenous urography, computed tomography (CT) scan of the chest and a large bowel barium study the effective radiation doses are 4.0, 8.0 and 9.0 mSv, respectively.
† Provided that the thyroid is blocked and the label is excreted in the urine; if not blocked, about 20% will accumulate in the thyroid, resulting in a radiation dose of 1 mSv to the thyroid.
Sources of radioisotopes
Radioisotopes that emit γ-rays are particularly useful because they have the advantage of emissions that penetrate tissues well, so they can be detected at the surface of the body when they have originated within organs. The radioisotope should have as short a half-life (T ½ ) as is compatible with the duration of the test. A radioisotope with a very short half-life can be administered in much larger amounts than those that are likely to remain active in the body for a considerably longer time.
The longer-lived radioisotopes that are used for haematological investigations are generally available from commercial suppliers. The usual way of obtaining certain short-lived radioisotopes is by means of a radioisotope generator, in which a moderately long-lived parent radioisotope decays to produce the required short-lived isotope. In this way 99m Tc (T ½ = 6 h) can be derived from 99 Mo (T ½ = 66 h).
Radiation protection
The quantity of radioactivity used in diagnostic work is usually small and good laboratory practice is all that is necessary for safe working. However, before using radioisotopes, workers should be familiar with the regulations concerning radiation protection for themselves, their fellow workers and patients.
The effect of radiation on the body depends on the amount of energy deposited and is expressed in grays (Gy). The unit that describes the overall effect of radiation on the body, or the ‘effective dose,’ is measured in sieverts (Sv) or millisieverts (mSv). The annual whole-body dose limit for somebody working with radioisotopes is in the order of 20 mSv, whereas 1 mSv is the annual limit for the general public. To put this into perspective, 1 mSv is produced by normal background radiation in about 6 months and the radiation dose from a single chest X-ray is 0.02 mSv. No statutory limit of total annual radiation dose has been set for patients, but it is an important requirement that radioisotopes should be handled only in approved laboratories under the direction of a trained person who holds a certificate from the appropriate authority specifying the radioisotopes that the individual is authorised to use and the dose limits that must not be exceeded. In the UK, this authority is the Administration of Radioactive Substances Advisory Committee (ARSAC, www.gov.uk/government/organisations/administration-of-radioactive-substances- advisory-committee ). Radioisotopes should not be given to pregnant women unless the investigation is considered imperative; if an investigation is necessary during lactation, breast-feeding should be discontinued until radioactivity is no longer detectable in the milk. When radioisotope investigations are necessary in children, the dose relative to that for an adult should be based on body weight ( Table 17-2 ).
Weight (kg) | Fraction of Adult Dose |
---|---|
10 | 0.3 |
15 | 0.4 |
20 | 0.5 |
30 | 0.6 |
40 | 0.75 |
50 | 0.9 |
60 | 0.95 |
70 | 1.0 |
The laboratory (premises) using radioisotopes should be registered to store, handle and dispose of radioactive materials, and appropriate permits are obtained under the Environmental Permitting Regulations 2010.
In general, the radioactive waste from radioisotopes used in haematological diagnostic procedures may be poured down a single designated laboratory sink. It should be washed down with a large quantity of running water. If the waste material exceeds the amount allowed for disposal in this way, it should be stored in a suitable place until its radioactivity has decayed sufficiently for it to be disposed of via the refuse system. All working and storage areas and disposal sinks should be clearly labelled with the internationally recognised trefoil symbol. Records should be kept of the amount of radioactive waste disposed down the drains and this should not exceed the permitted amount on the premises’ registration.
Decontamination of working surfaces, walls and floors can usually be achieved by washing with a detergent such as Decon 90 (Decon Laboratories, Ltd; www.decon.co.uk ). Glassware can be decontaminated by soaking in Decon 90 and plastic laboratory ware can be decontaminated by washing in dilute (e.g. 1%) nitric acid.
Protective gloves must always be worn when handling radioisotopes; any activity that does get on the hands can usually be removed by washing with soap and water or, if that fails, with a detergent solution. For each laboratory in which isotopes are used, a radiation protection supervisor (RPS) should be nominated to supervise protection procedures and to ensure that a careful record is kept of all administered radioisotopes. This RPS should work in association with the departmental safety officer (see p. 527) and must ensure that all personnel working with radioactive materials wear dosimetry badges (available from an approved dosimetry service provider, e.g. Landauer, www.landauer.co.uk ), which must be checked at regular intervals.
Apparatus for measuring radioactivity in vitro
The radioisotopes used for most haematological tests are measured in a scintillation counter with thallium-activated sodium iodide crystals. These are available in various shapes and sizes. A ‘well-type’ crystal contains a cavity into which is inserted a small container or test tube holding up to 5 ml of fluid. Because the sample is almost surrounded by the crystal, counting is achieved with high efficiency. Because the geometric efficiency of a well-type counter depends on the position of the sample in relation to the crystal, it is important to use the same volume for each sample in a series. Another form of crystal detector is a solid circular cylinder, 2.5–10 cm in diameter. In this form, it is used for in vivo measurements and occasionally for the measurement of bulky samples (e.g. samples of faeces or 24-h urine specimens), thus avoiding the need to concentrate them to a smaller volume.
An alternative method for measuring bulky material is by using two opposed detectors in a single counting system. The sample is placed in a 450 ml waxed cardboard carton with a screw-top lid and is positioned between two counters placed above and below it with a plastic ring over the lower counter to ensure that the specimen in the carton is approximately equidistant from both crystals. The counting system is surrounded by lead and the responses of both crystals are counted together. If a single detector system is used, it is essential to homogenise the samples.
Apparatus for measuring radioactivity in vivo
Surface counting
Surface counting depends on shielding the crystals by means of a lead collimator to exclude as far as possible the radiation from outside a well-defined area of the body. It is thus possible to measure the radioactivity in individual organs such as the spleen and liver.
Imaging
The most widely used method for imaging is by the scintillation camera (gamma camera). It consists of a lead shielding, a large thin sodium iodide detector, an array of photomultiplier tubes, a collimator with multiple parallel holes and a system for pulse height analysis and for storage and display of the data. By scanning down the body, an image of the distribution of the label is built up and recorded. It can also be used to measure the quantity of the isotope in various organs. By rotating the scintillation camera around the body, single-photon emission computed tomography (SPECT) can be performed to produce sectional images. Positron emission tomography (PET) has augmented scintillation scanning and uses radioisotopes that are positron emitters. ,
Measurement of radioactivity with a scintillation counter
Standardisation of working conditions
For each radioisotope, it is necessary to plot a spectrum of pulse height distribution and to identify a window corresponding to the energy at which the maximum number of pulses is emitted. Examples of spectra and selected settings are illustrated in Figure 17-1 . The setting of the apparatus, once determined, should remain constant for many months.

Counting technique
Measurement of radioactivity.
Measurements are usually carried out for a fixed time period and the results are recorded as counts per second (cps) or counts per minute (cpm). Radioactivity is subject to random but statistically predictable variation similar to that in blood cell counts (see p. 555). The accuracy of the count depends on the total number of the counts recorded as the variance (σ) of a radioactive count = √ total count.
Thus, on a count of 100 the inherent error is 10%, whereas it is 1% on a count of 10 000. Any measured activity represents the difference between the sample count and the background count, in which the errors of the two counts are cumulative. In practice, a net count of 2500 over background is adequate for the precision required for clinical studies.
Background counts should be measured alongside that of the radioactive material. If the count rate of the sample is not much above background, then the background should be counted for as long a time as the sample.
Correction for physical decay
Because physical decay is a continuous process that proceeds at an exponential rate, it is possible to correct mathematically for the loss of radioactivity and to convert any measurement back to the initial reference time. This is necessary when comparing successive observations made at different times after the administration of a radioisotope to a patient.
Double radioisotope measurements
If more than one radioisotope is present in a sample, it is possible to measure the radioactivity of each radioisotope separately by one of the following techniques.
Differential decay.
Differential decay is of value especially when one of the labels has a very short half-life (e.g. 99m Tc, half-life 6 h). The method is to count the activity in the mixture twice – the second count being performed after the short-lived label has effectively disappeared.
Physical separation.
When the two radioisotopes produce γ rays of different energies, they can be identified by their characteristic features and separated using an energy analyser. Correction for any ‘cross talk’ is carried out by counting a standard (a source of known radioactivity) of each radioisotope (A and B) at both channel settings. The proportion of A (P A ) spilling over into channel B = channel B counts ÷ channel A counts from the radioisotope A standard (both corrected for background at setting for B) and the proportion of B (P B ) spilling over into channel A = channel A counts ÷ channel B counts from the radioisotope B standard at setting for A. The total counts obtained for labels A and B in their correct channels can then be corrected for the proportion of ‘foreign’ counts.
Blood volume
The haemoglobin concentration (Hb), red cell count and packed cell volume or haematocrit (PCV/Hct) do not invariably reflect the total red cell volume (RCV). Whereas in most cases for practical purposes, there is adequate correlation between peripheral blood values and (total) RCV, there will be a discrepancy if the plasma volume is reduced or increased disproportionately. Fluctuation in plasma volume may result in haemodilution, giving rise to pseudoanaemia, or conversely, haemoconcentration, giving rise to pseudopolycythaemia.
An increase in plasma volume occurs in pregnancy, returning to normal soon after delivery. Increased plasma volume may also be found in patients with cirrhosis, nephritis and congestive cardiac failure and when there is marked splenomegaly. Reduced plasma volume occurs with oedema, with dehydration, following the administration of diuretic drugs, in smokers and sometimes as a persistent unexplained phenomenon. It also occurs during prolonged bed rest.
In contrast to the fluctuations in plasma volume, RCV does not fluctuate to any extent if erythropoiesis is in a steady state.
Measurement of blood volume should thus be considered whenever the Hct is persistently higher than normal; demonstration of an absolute increase in RCV is necessary to diagnose polycythaemia and to assess its severity. However, it should be noted that the discovery of a recurring JAK2 mutation ( JAK2 V617F) in the great majority of patients with polycythaemia vera means that blood volume studies are now rarely needed for the confirmation of this diagnosis. The component parts of the TBV (i.e. red cell and plasma volume) can also be measured separately in the elucidation of obscure anaemias when the possibility of an increase in plasma volume cannot be excluded.
Measurement of blood volume
Principle
The principle is that of dilution analysis. A small volume of a readily identifiable radioisotope is injected intravenously, either bound to the red cells or to a plasma component, and its dilution is measured after time has been allowed for the injected material to become thoroughly mixed in the circulation but before significant quantities have left the circulation or become unbound. The most practical method now available is to use a small volume of the patient’s red cells labelled with radioactive chromium ( 51 Cr), technetium (pertechnetate) ( 99m Tc) or indium ( 111 In). The labelled red cells are diluted in the whole blood of the patient and from their dilution the TBV can be calculated; the RCV, too, can be deduced from knowledge of the PCV/Hct. The plasma volume can be measured directly by injecting human albumin labelled with radioactive iodine ( 125 I) that is diluted in the plasma compartment.
In contrast to measurement of RCV, plasma volume measurements are only approximations because the labelled albumin undergoes continuous slow interchange between the plasma and extravascular fluids, even during the mixing period. For this reason, it is undesirable to attempt to calculate RCV from plasma volume on the basis of the observed PCV/Hct. However, because the RCV is generally more stable, calculation of TBV from RCV is usually more reliable, provided that the difference between whole-body and venous PCV is appreciated and allowed for (see p. 356). Measurement of red cell and plasma volumes separately by direct methods is to be preferred.
Red cell volume
Radioactive chromium method
For the radioactive chromium method, add approximately 10 ml of blood to 1.5 ml of sterile National Institutes of Health (NIH)-A acid–citrate–dextrose (ACD) solution (see p. 561) in a sterile bottle with a screw cap. Centrifuge at 1200–1500 g for 5 min. Discard the supernatant plasma and buffy coat and slowly, with continuous mixing, add to the cells 8 × 10 3 Bq of Na 2 51 CrO 4 ( www.polatom.pl or www.perkinelmer.com ) per kg of body weight. The sodium chromate should be in a volume of at least 0.2 ml, being diluted in 9 g/l NaCl (saline). Allow the blood to stand for 15 min at 37 °C for labelling to take place. Wash the red cells twice in 4–5 volumes of sterile saline: for all procedures requiring sterile saline, this should be 9 g/l (0.9%) sodium chloride BP (nonpyrogenic); 12 g/l NaCl should be used when red cell osmotic fragility is greatly increased (e.g. in cases of hereditary spherocytosis).
Finally, resuspend the cells in a volume of sterile saline sufficient for an injection of about 5 ml and the preparation of a standard. Take up the appropriate volume into a syringe that is weighed before and after the injection. The volume injected is calculated from the following formula:
Volume injected ml = Weight of suspension injected g Density of suspension g/ml
The density of the suspension = 1.0 + Hb of suspension (g/l) × 0.097/340, assuming that packed red cells have a mean cell haemoglobin concentration (MCHC) of 340 g/l and a density of 1.097 g/ml.
Inject the suspension intravenously without delay and note the time; at 10, 20 and 30 min later, collect 5–10 ml of the patient’s blood and add it to the appropriate amount of K 2 ethylenediaminetetra-acetic acid (EDTA) anticoagulant. This blood should preferably be drawn from a vein other than that used for the injection. However, it is often convenient to insert a self-retaining needle; in this case, care must be taken to ensure that the isotope is well-dispersed into the bloodstream when injected by flushing through with 10 ml of sterile saline. When the mixing time is likely to be prolonged, as in splenomegaly, cardiac failure or shock, another sample should be taken 60 min after the injection.
Measure the PCV of each sample. PCV should be obtained by microhaematocrit centrifugation for 5 min or for 10 min if the PCV is more than 0.50 and correcting for trapped plasma by deducting 2% from the measurement. A more accurate measurement of the PCV can be obtained by the International Council for Standardisation in Haematology (ICSH) surrogate reference method (see p. 24).
Deliver 1 ml volumes into counting tubes and lyse with saponin; a convenient method is to add 2 drops of 2% saponin. Measure their radioactivity in a scintillation counter. Then dilute an aliquot of the original suspension that was not injected 1 in 500 in water (for use as a standard) and determine the radioactivity of a 1 ml volume. Then:
Red cell volume RCV ml = Radioactivity of standard cpm /ml × Dilution of standard × Volume injected ml Radioactivity of post-injection sample cpm /ml × PCV (on blood sample)
Technetium method
99m Tc is available as sodium pertechnetate. This passes freely through the red cell membrane and will become attached to the cells only if it is present in a reduced form as it enters the cells when it binds firmly to β chains of haemoglobin. For this to occur, the red cells must be treated with a stannous (tin) compound by the following in vivo procedure.
Dissolve a vial of stannous reagent (stannous fluoride and sodium medronate available from Diagnostic Imaging Ltd, www.diagimaging.com or Medi-RadioPharma Co., Ltd, www.mediradiopharma.com ) in 6 ml of sterile saline and inject intravenously 0.03 ml/kg body weight.
After 15 min, collect 5 ml of blood into a sterile container to which has been added 200 iu of heparin. Add 2 MBq of freshly generated 99m Tc in approximately 0.2 ml of saline or 100 MBq if measurement of splenic red cell pool and scanning are also required. Allow to stand at room temperature for 5 min. Centrifuge; wash twice in cold sterile saline and resuspend in a sufficient volume of cold sterile saline for an injection of 5–10 ml. Draw 5 ml into a syringe that is weighed before and after injection and carry out subsequent procedures as for the chromium method. Because of the short half-life of 99m Tc, radioactivity must be measured on the day of the test. Because 5–10% of the radioactivity is eluted from the red cells within an hour, the method is less suitable than the chromium and indium methods when delayed mixing is suspected (e.g. in splenomegaly).
Indium is available as 111 In chloride. The labelling procedure is simpler than with 99m Tc and, because there is less elution than with technetium during the first hour, it is particularly suitable for delayed sampling. For labelling blood cells, the indium is complexed with oxine or tropolone.
Calculating total blood volume
The TBV can be calculated by multiplying the value for RCV by 1/(whole-body PCV) (see below). Plasma volume can be calculated by subtracting RCV from TBV.
If a sample has been taken at 60 min in cases in which delayed mixing is suspected and there is a significant difference between the measurements at 10–30 min and 60 min, then the 60 min measurement should be used for calculating the RCV.
Plasma volume
125 l-human serum albumin method
Human serum albumin (HSA) labelled with 125 I or 131 I is available commercially (from Mallinckrodt Radiopharmacy Services, www.ucl.ac.uk/nuclear-medicine ). The albumin concentration should not be less than 20 g/l. The user must ensure that the albumin is derived from plasma that has been thoroughly tested for viral infections. 125 I is readily distinguishable from 51 Cr, 99m Tc and 111 In and this makes possible the simultaneous direct determination of RCV and plasma volume (see below). If further doses of the radioisotope are to be administered for repeat tests, it is advisable to block the thyroid by administering 30 mg of potassium iodide by mouth on the day before the test and daily for 2–3 weeks thereafter.
Withdraw approximately 20 ml of blood into a syringe containing a few drops of sterile heparin solution and transfer to a 30 ml sterile bottle with a screw cap. After centrifuging at 1200 to 1500 g for 5–10 min, transfer approximately 7 ml of plasma to a second sterile bottle and add 2.5 × 10 3 Bq of the radioisotope-labelled HSA per kg body weight (approximately 0.2 MBq in total). Inject a measured amount (e.g. 5 ml) and retain the residue for preparation of a standard.
After 10, 20 and 30 min, withdraw blood samples from a vein other than that used for the original injection (or after prior flushing through with 10 ml of sterile 9 g/l NaCl [saline] if a butterfly needle has been used) and deliver into bottles containing EDTA or heparin.
Measure the PCV (see above), centrifuge the sample and separate the plasma. Prepare a standard by diluting part of the residue of the uninjected HSA 1 in 100 in saline.
Measure the radioactivity of the plasma samples in a scintillation counter and, by extrapolation on semi-logarithmic graph paper, calculate the radioactivity of the plasma at zero time. If only a single sample is collected 10 min after the injection, the radioactivity at zero time may be approximated by multiplying by 1.015 to allow for early loss of the radioisotope from the circulation. Reliance on a single 10 min sample will lead to error if the mixing of the albumin in the plasma is delayed. After measuring the radioactivity of the standard, the plasma volume (ml) is calculated as follows:
Radioactivity of standard cpm /ml × Dilution of standard × Volume injected ml Radioactivity of postinjection sample cpm /ml , adjusted to zero time
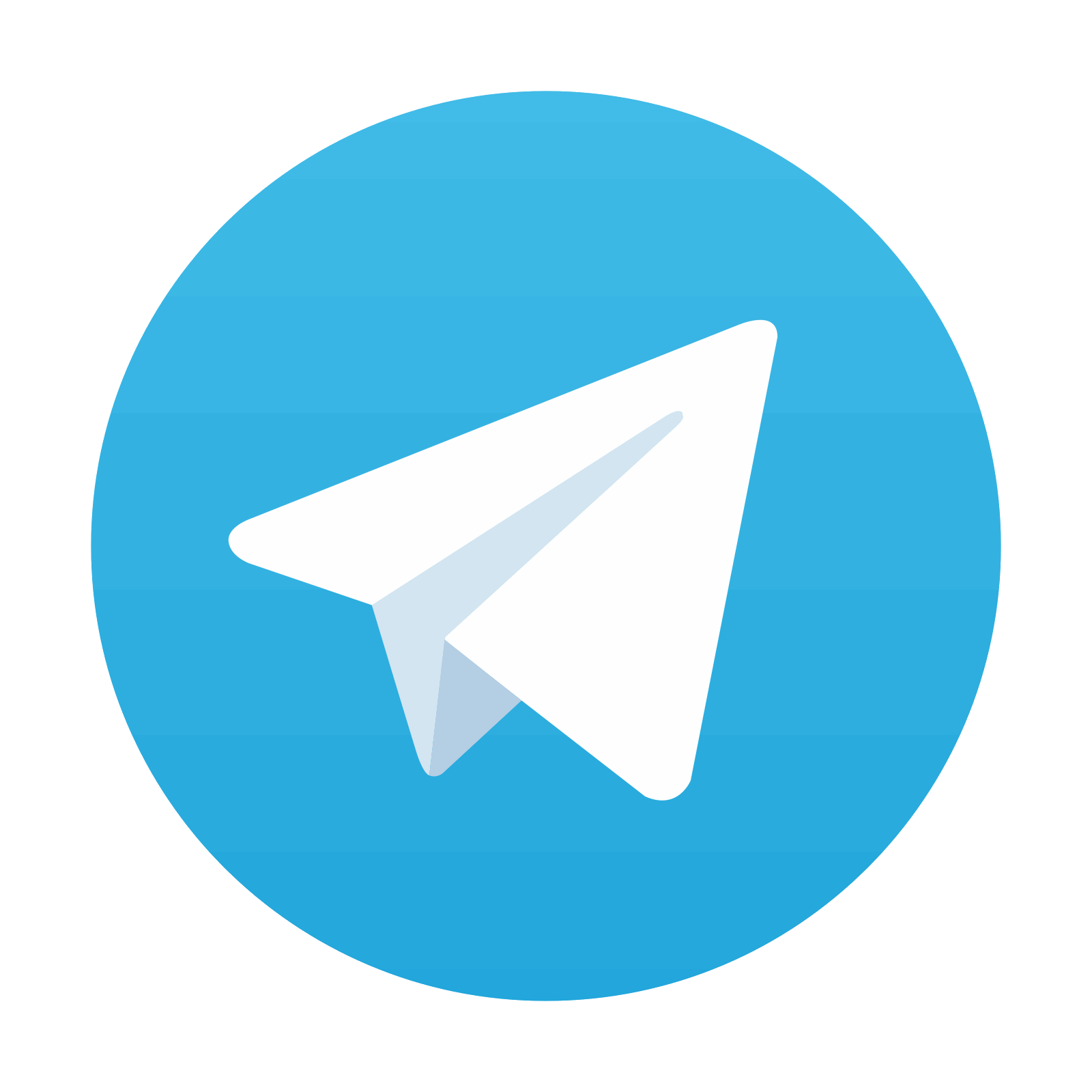
Stay updated, free articles. Join our Telegram channel

Full access? Get Clinical Tree
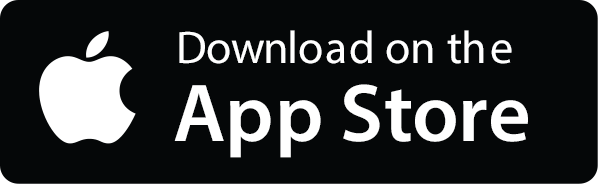
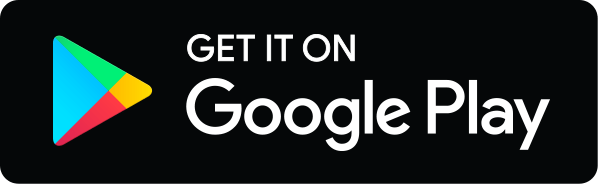