The majority of cases of diabetes mellitus falls into one of two categories: (1) type 1 diabetes, which is most commonly caused by autoimmune destruction of the pancreatic islet cells resulting in β-cell failure and an absolute deficiency of insulin (type 1 A diabetes, T1DM), and (2) type 2 diabetes (T2DM), which results from a combination of insulin resistance in target tissues and a lack of an adequate compensatory insulin response to overcome this resistance. The American Diabetes Association (ADA)1 recognizes more than 50 other specific types of diabetes (Table 11-1). All of these disorders collectively account for only 1% to 5% of cases of diabetes (see Part D of this chapter later on).
Initial presentation in patients < 6 mo old |
Neonatal diabetes |
Transient |
Permanent |
Genetic defects in insulin action |
Rabson-Mendenhall syndrome |
Donahue syndrome (leprechaunism) |
Initial presentation in patients > 6 mo old |
Type 1 diabetes |
Immune-mediated |
Slowly progressive type 1 diabetes |
Nonimmune-mediated |
Type 2 diabetes |
Monogenic diabetes |
Atypical diabetes (Flatbush) |
Maturity-onset diabetes of youth (MODY) |
MODY1 |
MODY2 |
MODY3 |
MODY4 |
MODY5 |
MODY6 |
MODY 7 |
MODY 8 |
Mitochondrial diabetes |
Genetic defects in insulin action |
Type A insulin resistance |
Lipoatrophic diabetes |
Diseases of the exocrine pancreas |
Cystic fibrosis |
Trauma/pancreatectomy |
Neoplasia |
Pancreatitis |
Hemochromatosis |
Endocrinopathies |
Acromegaly |
Cushing syndrome |
Glucagonoma |
Pheochromocytoma |
Hyperthyroidism |
Somatostatinoma |
Aldosteronoma |
Drug- or chemical-induced |
Corticosteroid-induced |
Others |
Infections/critical illness |
Uncommon forms of immune-mediated diabetes |
“Stiff man” syndrome |
Type B insulin resistance |
Autoimmune hypoglycemia |
Genetic syndromes associated with diabetes |
Down syndrome |
Klinefelter syndrome |
Turner syndrome |
Wolfram syndrome |
Friedreich ataxia |
Huntington chorea |
Laurence-Moon-Biedl syndrome |
Myotonic dystrophy |
Porphyria |
Prader-Willi syndrome |
IPEX |
Gestational diabetes mellitus |
Ensuring a correct diagnosis and defining the correct type of diabetes is important for choosing the most appropriate therapy, managing the expected complications, anticipating associated disorders, and predicting disease risk in relatives or reoccurrence in offspring. Although clinical differences at the time of diagnosis usually allow the presumptive classification of patients as T1DM or T2DM, the classification is not always clear-cut. Likewise, it is important to know when to consider one of the more unusual forms of diabetes, as differentiating them from the more common type 1 and type 2 diabetes can present a significant diagnostic challenge.
A complete discussion of the different types of diabetes is presented in the subsequent parts of this chapter. Part B: Type 1 Diabetes and Part C: Type 2 Diabetes present the epidemiology, specific clinical presentations, and treatments of type 1 and type 2 diabetes, respectively. Part D: Other Specific Types of Diabetes Mellitus and Causes of Hyperglycemia, discusses diabetes in infants less than 6 months at diagnosis as well as the differential diagnosis of patients with an autosomal dominant or maternally transmitted patterns of diabetes inheritance as well as diabetes associated with medication or other endocrine disorders.
The criteria for making the diagnosis of diabetes are the same, regardless of the etiology. The diagnostic criteria are presented in Table 11-2. Type 1 and type 2 diabetes mellitus are, by far, the most common forms, but atypical presentations or clinical course should alert the clinician that one of the less common forms may be the actual diagnosis.
Any of the following findings on two separate occasionsa: |
Fasting plasma glucose ≥ 126 mg/dL |
OR |
2-h glucose ≥ 200 mg/dL after a 75-g glucose load (or 1.75 g/kg) |
OR |
HbA1c ≥ 6.5%. The test should be performed in a laboratory using a method that is NGSP-certified and standardized to the DCCT assay. |
In the absence of a preceding history of polydipsia and polyuria, a diagnosis of diabetes mellitus should not be made based on hyperglycemia documented during a serious illness. This stress-induced hyperglycemia is usually moderate in degree, typically no higher than 200 to 250 mg/dL, although levels above 600 mg/dL have been reported. Typically, the glucose rapidly normalizes in these patients as the illness resolves. If blood glucose testing after resolution of the intercurrent illness does not confirm the presence of hyperglycemia or diabetes, the risk that such a child will develop diabetes in the future is very low, unless the child has measurable diabetes-associated autoantibodies, or is an adolescent with high risk factors for type 2 diabetes. Elevations in HbA1c are indications of sustained hyperglycemia (Table 11-2).
The differential diagnosis of hyperglycemia and diabetes mellitus is listed in Table 11-1 and Figures 11-1 and 11-2. While this is an extensive list, the vast majority of children have type 1 diabetes, up to 50% of newly diagnosed teens in minority groups may have type 2 diabetes, and the other forms are uncommon. Nevertheless, it is important to recognize unusual presentations or an atypical clinical course for childhood diabetes and look further for these rarer forms of diabetes. When generating a differential diagnosis, it is important to discriminate between diseases in which diabetes is the primary abnormality and those where diabetes is a secondary abnormality presenting as a symptom of another disorder or syndrome (Figure 11-3). It is also important to recognize the different causes of diabetes in patients who develop diabetes before versus after 6 months of age (see Part D).
As β-cell function declines the serum glucose level rises. The symptoms of hyperglycemia are similar regardless of the underlying cause and include polyuria, polydipsia, polyphagia, blurry vision, and fatigue, and, in cases of severe insulin deficiency, weight loss. Patients may be asymptomatic if mild hyperglycemia is present. Once the blood glucose concentration exceeds the capacity of the proximal renal tubules to reabsorb glucose, which is at approximately 180 mg/dL, some of this glucose is lost in the urine. This glucosuria serves as an osmotic force that increases water and electrolyte excretion. As urinary fluid losses increase, the thirst center is activated to work to maintain normal hydration leading to polydipsia. The glucosuria also leads to a loss of calories; this combined with the loss of the anabolic actions of insulin results in weight loss. This physiology explains the signs and symptoms of diabetes: polydipsia, polyuria, polyphagia, and weight loss.
Typical complaints that suggest polyuria include the reemergence of bed-wetting, new or increased nocturia, and disruption of class work in school for frequent visits to the bathroom or requests to get water to drink. These symptoms are typically only present for less than 1 month. This acute presentation is most common in T1DM, however, T2DM and several of the more unusual forms of diabetes can also present acutely.
In evaluating a child for diabetes it is important to obtain a thorough history and review of symptoms and to perform a full physical examination to ensure that the diagnosis of diabetes type is made as accurately as possible. The history should focus on the acuity of the symptoms, as patients with diabetes resulting from insulin resistance often have an insidious course whereas diabetes owing to insulin insufficiency is usually more acute in onset. Insulin-resistant adolescents may exhibit other signs of insulin resistance such as obesity, acanthosis nigricans of the neck, axillary area, knees, or inguinal creases, hypertension, and, in females, a history of oligomenorrhea or even amenorrhea. (If sexually active, pregnancy should be ruled out if amenorrhea is present.) Calculation of the body mass index (BMI* = kg/m2) may help to quantify the degree of overweight/obesity and percentiles for age, gender, and ethnicity are published (see Chapter 9).1 Stress-induced diabetes and medication-induced hyperglycemia are not usually associated with symptoms of polyuria, polydipsia, or weight loss before the precipitating event resulted in elevated blood glucose concentrations.*
*The body mass index (BMI) is a measure of adiposity, and an abnormal BMI confers increased morbidity in general, including an increased risk for the development of type 2 diabetes. BMI is calculated as (weight in kg/height [in meters squared]). Charts of BMI versus age for boys and for girls are shown in Chapter 9).
It is important to obtain a list of the patient’s prescribed and over-the-counter (OTC) medications to determine if the diabetes is as a result of medication use (see Diabetes Part D for medications causing hyperglycemia). In addition, a detailed family history will allow detection of similarly affected family members, which becomes especially important when trying to ascertainif type 2 diabetes, maturity-onset diabetes of the young (MODY) (autosomal dominant), or mitochondrial diabetes (maternal transmission) are possible diagnoses. In all patients, but especially in infants, close attention should be given to the presence or absence of dysmorphic features or prototypical features which can be seen in the various genetic and endocrine disorders that have diabetes as one of their manifestations (see Figure 11-3).
If severe hyperglycemia is left untreated, the patient can develop nonketotic hyperosmolar syndrome or ketoacidosis, so inquiries regarding the presence of nausea, vomiting, abdominal pain, volume of urine output, fluid intake, difficulty breathing, and alterations in mental status should be made. If type 1 diabetes is suspected, laboratory confirmation of diabetes should be obtained urgently. The initial physical assessment should include vital signs, with specific attention paid to the heart rate and blood pressure, as these may be abnormal if dehydration is present. If tachycardia is present in the setting of suspected fluid depletion, the blood pressure should be taken with the patient in both a supine and upright position to assess hydration status. Orthostatic hypotensionin adults is defined as a decrease in blood pressure in the upright position of more than 20/10 mm Hg. In a child that presents with nausea and vomiting, diabetic ketoacidosis (DKA) should be considered if there is a preceding history of polydipsia and polyuria, particularly in the absence of supportive evidence of a viral illness, such as lack of sick contacts, diarrhea, and fever. Additionally, the history of polyuria in a dehydrated-appearing child should alert the clinician to the possibility of diabetes with DKA as the etiology of the vomiting. The presence of Kussmaul respirations can serve as indicators of the patient’s acid/base balance and suggest the presence of a significant metabolic acidosis (see Part B later in this chapter).
In the presence of a history indicating polydipsia and polyuria, a single random, or casual, plasma glucose concentration of 200 mg/dL or greater confirms the diagnosis of diabetes. However, there are situations when glucosuria or hyperglycemia is identified as an incidental finding in an individual without symptoms of diabetes. In these situations, the diagnosis of diabetes is based on finding an elevated plasma glucose level (see Table 11-2): a fasting level of greater than 125 mg/dL, a random value greater than 199 mg/dL, or a level greater than 199 mg/dL at 2 hours during an oral glucosetolerance test (OGTT). Only in the absence of symptoms an abnormal value should be confirmed on a second day. A hemoglobin A1c level of 6.5% or greater is a diagnostic test for diabetes in the presence of diabetic symptoms; alternatively, in an asymptomatic child any combination of elevated fasting plasma glucose, elevated 2-hour plasma glucose during an OGTT, or elevated hemoglobin A1c on two separate occasions (eg, at least 2 separate days) is diagnostic for diabetes.
Glycosuria and ketonuria are highly indicative of diabetes but a plasma glucose level of greater than 200 mg/dL is required to confirm a diagnosis of diabetes, while documentation of acidosis (venous pH < 7.3 and/or HCO3 < 15 mEq/L) and ketonemia or ketonuria document DKA.
In an individual with evolving type 1 diabetes, the period of time prior to the development of symptoms of diabetes during which there are abnormalities of glucose control is usually relatively brief. Therefore, it is not useful to screen for type 1 diabetes except within a research trial.
In the past, a diagnosis of diabetes mellitus in a child rarely involved consideration of causes other than type 1 diabetes. However, there are now increasing number of children and adolescents identified with type 2 diabetes. Therefore, there are clinical scenarios where etiologies other than type 1 diabetes must be considered, including children with risk factors for type 2 diabetes (see Figure 11-2).
Clinical differences at the time of diagnosis, more completely discussed in parts B and C, usually do allow presumptive classification of most patients as T1DM or T2DM. However, classification is not always clear-cut. Obese and minority pubertal children and adolescents, those with a parent with type 2 diabetes and those with signs of insulin resistance (acanthosis nigricans, hypertension, or hyperlipidemia) are more likely to have T2DM. However, the weight distribution of children with T1DM is proportionate to the weight distribution in the general population. Thus, approximately 25% of children with T1DM would be expected to be overweight, although they are rarely as severely overweight as most patients with T2DM. Additionally, while family history is important in identifying the possibility for T2DM, the family history of diabetes alone is not sufficient to differentiate the two.
Glycated hemoglobin (HbA1c) levels are not helpful in differentiating between the two diabetes types. Ketosis or ketoacidosis is more common with T1DM, however, up to 33% of type 2 patients have ketosis at disease onset and 10% may present with DKA because of hyperglycemia-induced β-cell toxicity, resulting in very low insulin levels. Owing to this suppressed β-cell function, C-peptide levels may not be helpful in classification at onset of diabetes since they may be low at diagnosis of T2DM and normal during the honeymoon phase of T1DM. Individuals who do not follow the typical course expected for T2DM may be tested for C-peptide levels2 years following diagnosis of diabetes. The C-peptide level at this time may be helpful in differentiating diabetes type since T2DM patients generally still have modestly elevated insulin levels while T1DM individuals usually have low insulin levels 2 years after diagnosis.
Further discussion of the differentiation of T1DM versus T2DM is presented in Part C: Type 2 Diabetes.
In young infants or children with a family history suggesting an autosomal dominant or maternally inherited pattern of diabetes, a diagnosis of neonatal diabetes, MODY, or maternally inherited diabetes could be considered (see Table 11-1). These forms of diabetes are not autoimmune-mediated, generally are not associated with increased insulin resistance, and have characteristic clinical presentations and findings. A definite diagnosis requires specific genetic testing. The clinical presentations and diagnostic tests are described in more detail in Part D.
The prevalence of type 1 diabetes (T1DM) in children 0 to 19 years of age in the United States is approximately 2 per 1000.1 The incidence of T1DM varies substantially across the globe, with rates near 1 per 100,000 person-years in China and parts of South America, while rates above 30 per 100,000 person-years are found in Sweden, Finland, and Sardinia. The incidence of T1DM in non-Hispanic white children in the United States is now greater than 20 per 100,000 children per year.2 Numerous studies, including some from the United States, have indicated a worldwide increase in the incidence of T1DM of approximately 3% per year, with the greatest increase seen in children younger than 5 years of age.2*
*This part is reproduced with permission in part from Cooke DW, Plotnick L. Type 1 diabetes in pediatrics. Pediatr Rev. 2008;29:374-384; and Cooke DW, Plotnick L. Management of diabetic ketoacidosis in children and adolescents. Pediatr Rev. 2008;29:431-435.
The incidence of T1DM in the United States is highest in non-Hispanic white children. The incidence in African American and Hispanic children 0 to 14 years of age is only approximately one-half that of non-Hispanic white children, although the difference is less in children 15 to 19 years of age. The incidence is lowest in American Indian and Asian/Pacific Islander children.
Males and females have an equal incidence of T1DM. There are two peaks in its age-specific incidence: the largest is at 10 to 14 years of age, with a smaller peak in earlier childhood. There is some seasonal variation in the onset of T1DM, with fewer cases presenting during the summer months; this seasonal variation has decreased over the past 10 years.
T1DM is caused by an absolute deficiency of insulin. In almost all cases, this is caused by an autoimmune process that results in the destruction of the insulin-producing β-cells of the pancreatic islets. This type 1A diabetes is what is traditionally referred to when discussing “type 1” diabetes. A rare form of insulinopenic diabetes, type 1B, can occur without evidence of autoimmunity; these patients are most often of African or Asian ancestry. It is strongly inherited, but does not share the same genetic linkage to the HLA locus as type 1A diabetes. The causeof type 1B diabetes is not known, although some cases have now been identified as forms of monogenicdiabetes. Type 1 diabetes is felt to occur when an environmental trigger initiates an autoimmune response to the pancreatic β-cells in a genetically susceptible individual.3 Once initiated, immune-mediated destruction of the β-cells leads to a degree of insulinopenia that prevents normal glucose homeostasis, and the patient develops hyperglycemia (Figure 11-4). The inhibition of lipolysis is sensitive to low amounts of insulin, so that initially hyperglycemia exists without significantly disordered lipid metabolism. However, if the diabetes is unrecognized for a period of time, the insulinopenia worsensand the patient is at risk of developing ketoacidosis (DKA) from the combined effects of unrestrained lipolysis and hyperglycemia.
Figure 11-4
Evolution of type 1 diabetes mellitus, an immunologic trigger from the environment initiates an autoimmmune response to pancreatic β-cells. This leads to the destruction of β-cells, ultimately resulting in sufficient insulinopenia to cause diabetes. The rate of decline in β-cell mass varies among individuals, with diabetes devolving once greater than 80% of β-cell mass is lost. During the “honeymoon” period, some β-cell function remains, somewhat mitigating exogenous insulin requirements. (Reproduced with permission from Michels AW, Eisenbarth GS: Immunologic endocrine disorders. J Allergy Clin Immunol 2010 Feb;125(2 Suppl 2):S226-S237.)
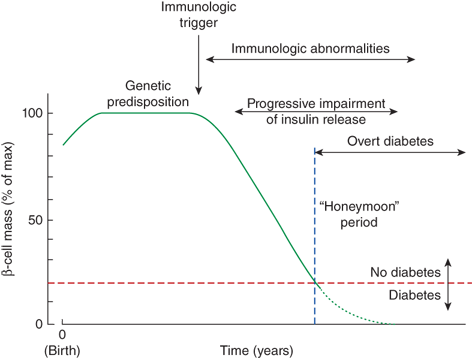
Greater than 80% of the pancreatic β-cells must be lost before a patient becomes sufficiently insulinopenic to develop hyperglycemia. The time from the initiation of the autoimmune response to diabetes can vary from months to years. In general, the process appears to be more rapid in infants and younger children, and to be more gradual in older children and adolescents. Similarly, the time after diagnosis during which significant insulin production persists can vary from weeks to years, with the duration generally being inversely related to the age at presentation. This persistence of insulin secretion results in a “honeymoon” period, where exogenous insulin requirements and glucose fluctuations are both smaller compared to the situation that occurs once minimal or no insulin secretory activity remains.
There is a clear genetic component to the risk of T1DM, as indicated by the increased risk of diabetes in relatives of individuals with diabetes (see Table 11-3). It is important to note, however, that most children diagnosed with T1DM are the first individual in their family with diabetes: only 10% to 20% of individuals with T1DM have a family member with T1DM.
General population | 0.2% |
Individuals with a relative withtype 1 diabetes: | |
Sibling | 6% |
Identical twin | 30%-40% |
HLA | |
Identical | 15% |
Haploidentical | 6% |
Nonidentical | 1% |
Offspring | 5% |
Father with IDDM | 6% |
Mother with IDDM | 2% |
The strongest contributor to the genetic risk of T1DM, contributing 30% to 50%r of that risk, is the major histocompatibility complex (MHC) on chromosome 6. The overwhelming contribution of this risk comes from the genes encoding the class II antigens DRB1 and DQB1, although there is also evidence of risk associated with class I alleles as well. These genes are felt to alter the risk of T1DM by affecting the ability of these class II molecules to bind to and present β-cell protein peptide fragments to T cells and, therefore, to activate an autoimmune reaction.
The risk associated with the MHC is complicated by both the extensive allelic variation of these genes (there are over 1000 HLA DRB1 alleles) as well as the genetic linkage between MHC genes.
There are specific histocompatibility loci antigens (HLAs) that confer an increased risk of T1DM and others that confer a decreased risk.4 For some genes, the relative risk is affected by the linked genes within a haplotype. In general, the linked DR/DQ haplotypes HLA-DR3DQA1*0501-DQB1*0201 (DR3/DQ2) and HLA-DR4-DQA1*0301-DQB1*0302 (DR4/DQ8) are associated with an increased risk of type 1 diabetes. The DQ6.2 allele (DQA1*0102-DQB1*0602), on the otherhand, confers dominant protection from T1DM (ie, there is decreased risk in the presence of DQ6.2, even in individuals with DR3 and/or DR4). Additionally, as an example of risk related to linked genes, the DRB1*0401-DQB1*0302 haplotype is associated with a significantly increased risk, while DRB1*0401-DQB1*0301 is a modestly protective haplotype. Thus, while 40% of US Caucasians have an HLA-DR3 or -DR4 allele, 95% of patients with T1DM have at least one of these alleles. Only 2.4% of the general population carries the combined HLA-DR3/4 genotype, while 30% to 40% of patients with T1DM have the HLA-DR3/4 genotype. In contrast, while 20% of the general US population has DQ6.2, it is only found in 1% of those with T1DM. Importantly, however, the role of other genes—and factors other than genes—in the development of the disease is indicated by the fact that most individuals with a predisposing haplotype do not develop the disease. While 2% of the population carries the high-risk DR3-DR4/DQ8 genotype, which confers a 15-fold increased risk compared to the general population, still only 1 in 20 of these individuals will actually develop T1DM.
A site of a variable number of tandem repeats (VNTR) upstream of the insulin and IGF2 genes onchromosome 11 contributes approximately 10% to the genetic risk of T1DM. Class I alleles, with 20 to 63 repeats, are associated with susceptibility to T1DM, while class III alleles, with 140 to 210 repeats are associated with protection. Studies have demonstrated that class III alleles are associated with an increase in expression of the insulin gene in the fetal thymus, and it has been proposed that this leads to improved recognition of insulin as a self-antigen, inhibiting autoimmune recognition of β-cells. In addition, imprinting of this region or, alternatively, unequal parental transmission of class III versus class I alleles may be responsible for the increased risk of diabetes in offspring of fathers with T1DM compared to offspring of mothers with T1DM.
The loci in the MHC on chromosome 6 and near the insulin gene on chromosome 11 together confer approximately 50% of the genetic risk of T1DM. Numerous other loci have been identified as confirmed or potential contributors to the remainder of the genetic risk for T1DM. These include genes encoding a number of proteins involved in immune regulation: protein tyrosine phosphatase, nonreceptor type 22 (lymphoid) (PTPN22); cytotoxic T-lymphocyte-associated protein 4 (CTLA4); interleukin-2 receptor-α (IL2RA); and interferon induced with helicase C domain 1 (IFIH1).
The concordance for T1DM in monozygotic twins is only 30% to 40% for diabetes onset by 20 to 30 years of age. While the concordance rate rises to 65% in individuals followed to age 60 years, the lack of full concordance implicates additional factors beyond genetic risk in the development of T1DM. This could be owing to differences that exist in monozygotic twins, including differences in immunologic repertoire through the processof T- and B-cell receptor gene rearrangements, somatic mutations, or other such processes. Alternatively, environmental factors may play a role in the pathogenesis of T1DM. Among other data suggesting a role of environmental factors is the finding that children of parents who have migrated from a low-incidence country have an increased risk if born in a high-incidence region.
Viruses, including mumps, rubella, and coxsackievirus, have been implicated in the pathogenesis of T1DM.5 However, except for congenital rubella infection, where up to 20% of such individuals will develop T1DM, the involvement of other viruses as significant contributors to the causation of type 1 diabetes remains unproven. Exposure to the rodenticide, Vacor, can lead to diabetes, and other environmental toxins such as nitrosoureas have been proposed as triggers for β-cell autoimmunity, but none have been conclusively linked to the development of T1DM. Some studies have found that individuals who were exclusively breast-fed as infants are at lower risk of developing T1DM. Early exposure to cow’s milk and an immunological reaction to bovine milk proteins have been proposed to explain this finding. Mechanisms hypothesized to explain the role of these environmental factors in triggering β-cell autoimmunity include exposure of β-cell antigens after cellular injury owing to toxins or infection, induction of a superantigen response, and molecular mimicry whereby the immune system is triggered by a foreign antigen that shares common or similar immunological epitopes with a β-cell antigen.
Vitamin D has been implicated in regulating the immune system and having involvement in autoimmune diseases, including T1DM. Indeed, the geographic variation in type 1 diabetes incidence, with higher prevalence in northern latitudes where there is less sunlight-induced vitamin D synthesis, supports such a role. Other data also implicate lower vitamin D levels as increasing the riskfor T1DM, including association studies showing lower vitamin D levels in patients with newly diagnosed diabetes compared with controls, and observational studies showing a lower risk of T1DM in infants who had been supplemented with vitamin D compared to controls. However, the prospective Diabetes Autoimmunity Study in the Young (DAISY) found no association between vitamin D levels or intake and the development of diabetes autoantibodies or progression to T1DM.
The immune reaction in T1DM includes the development of antibodies and T lymphocytes targeted to β-cells and β-cell antigens as well as cytokine release within the pancreatic islets. While β-cell autoantibodies are found in greater than 80% of patients at the time of diagnosis of T1DM, they do not appear to play a direct role in β-cell destruction, which is a T-cell mediated process. The most compelling evidence for this is that transfer of T cells, but not of antibody, can induce autoimmune diabetes in experimental animal models. The β-cell destruction is thought to be caused by a combination of direct CD8+ T-cell attack of the β-cell, along with cytotoxicity from free radicals and cytokines (principally IL-1, TNF-α, TNF-β, and IFN-γ) released from macrophages and CD4+ and CD8+ T cells.
Diabetes-associated autoantibodies can be identified in the serum of patients months to years before the development of diabetes, indicating a prolonged preclinical course of the autoimmune process. Commerciallyavailable assays exist for the detection of antibodies against whole islets (islet cell antibodies [ICAs]), and antibodies against insulin, the 65-kd isoform of glutamic acid decarboxylase (GAD-65), and insulinoma-associated protein 2 (IA-2, also identified as islet cell antigen 512 [ICA512]). Notably, of the specific protein targets identified as diabetes-associated autoantibodies, insulin is the only protein unique to β-cells. With the exception of insulin autoantibodies, each of these autoantibodies is detected in approximately 70% to 80% of patients at the time of diagnosis of diabetes. Insulin autoantibodies (IAAs) are often the first diabetes autoantibody detected, although the prevalence of IAAs at the time of diagnosis is somewhat lower than that of the other autoantibodies. It is unclear if all individuals with positive diabetes-associated autoantibodies will develop diabetes, but their presence identifies individuals at higher risk of diabetes.
Measuring diabetes-associated antibodies in children in the general population has not been proven to be sufficiently predictive or cost-effective to justify widespread screening. While one study found that positive ICAs indicated a 45% 7-year risk of T1DM, other studies have found ICAs in 3% to 4% of children, and an observed or predicted risk of only 6% to 7% in such children. In contrast, the presence of diabetes-associated antibodies in individuals with a family member with diabetes can be highly predictive for the development of diabetes. The risk of developing diabetes is highest in individuals with multiple β-cell autoantibodies, with a risk of developing diabetes within 5 years greater than 25% in those with two autoantibodies and greater than 50% in those with three or more autoantibodies. The newly identified autoantibody to the zinc transporter 8 (ZnT8) has been identified as a potential marker for increased risk of progression in autoantibody-positive individuals.
As more is learned about the pathophysiology underlying T1DM, as well as information learned from studies of its epidemiology, consideration is being givento whether it is possible to prevent its development. Certainly it can be justified to endorse interventions with little or no risk that provide benefits beyond the potential to prevent T1DM—such as prolonged breastfeeding. For other interventions, it is necessary to understand the risks and to balance them against the likelihood and benefits of success. Ongoing research studies are aimed at either primary or secondary prevention of T1DM.
Primary prevention studies seek to interrupt the natural history of the development of T1DM at some time prior to its clinical presentation. These studies could in theory involve interruption prior to the initiation of the autoimmune attack of β-cells or after the initiation of the autoimmune reaction, but at a time when sufficient β-cells remain to allow normal glucose homeostasis. A critical aspect to primary prevention is to identify individuals at increased risk of developing T1DM compared to the low risk in an unselected population (~0.4%). By evaluating genetic risk (through family history and/orHLA typing), measuring diabetes-associated autoantibodies, and characterizing insulin secretion and glucose control, such individuals can now be identified and accurately stratified into groups with defined risk. Recent prevention trials have included investigations of treatment with insulin (both oral and parenteral) and with a 65-kD isoform of glutamic acid decarboxylase (GAD-65). Thus far, however, no successful primary prevention has been identified. Because of this, except for within research studies (such as those coordinated through the Type 1 Diabetes TrialNet), there is no indication to screen individuals to identify those at risk.
Secondary prevention studies seek to terminate the autoimmune process at or shortly after the individual presents with clinically diagnosed diabetes—a time when as much as 10% to 20% of normal β-cell function remains. Because optimal control of diabetes is more successfully achieved when the individual retains residual, albeit subnormal, endogenous insulin secretion (as is seen during the “honeymoon” period), successful secondary prevention would be of great benefit to patients. Such attempts at interventions in the autoimmune process began in the 1970s and 1980s, utilizing prednisone, antithymocyte globulin and cyclosporine. Successful secondary prevention has not yet been demonstrated, although a number of approaches to modulating the immune system at the time of diagnosis have shown potential for success to this approach. Current studies include those coordinated by the Type 1 Diabetes TrialNet and by the Immune Tolerance Network and include those utilizing anti-CD3, anti-CD20 and costimulation blockade agents.
Major immune intervention studies include the diabetes prevention trial type 1 (DPT-1) and continuing in TrialNet. A number of immunomodulatory agents have been evaluated including various monoclonal antibodies, oral, parenteral and intranasal insulin, and GAD-antigen.
reference:
Tables 11-4A and B outline the presenting findings of T1DM and of ketoacidosis. The hallmark signs and symptoms of diabetes are polydipsia, polyuria, polyphagia, and weight loss. Typical complaints that suggest polyuria include the reemergence of bed-wetting, new or increased nocturia, and disruption of class work in school for frequent visits to the bathroom or requests to get water to drink. These symptoms are typically only present for less than 1 month.
Hyperglycemia | Ketoacidosis | Dehydration | Insulin Resistance |
---|---|---|---|
Asymptomatic | Nausea | Tachycardia | Obesity |
Polyuria | Vomiting | Normotensive or hypotensive (late finding) | Acanthosis nigricans |
Polydipsia | Abdominal Pain | Hirsutisma | |
Polyphagia | Tachypnea | Sunken eyes | Irregular menses or amenorrheaa |
Weight loss | Kussmaul respirations | Poor skin turgor | |
Blurry vision | Sweet-smelling/fruity breath (acetone odor) | Dry mucous membranes | Acnea |
Fatigue | Altered mental status | Cool extremities | Hepatic steatosis |
Nocturia | Lethargy | Delayed capillary refill | |
Secondary enuresis | Coma | ||
Candidiasis—oral and/or cutaneous |
Hyperglycemia | Ketoacidosis | Insulin Resistance |
---|---|---|
Glycosuria | Glucosuria | Elevated insulin |
Ketonuria/ketonemia | Elevated C-peptide | |
Anion gap acidosis | Elevatedtrigylcerides | |
Decreased pH | Elevated liver enzymes | |
Decreased Pco2 |
Up to 40% of children with T1DM present with varying degrees of DKA. The development of DKA requires a greater degree of insulin deficiency than that which causes hyperglycemia, so that these children often will have a preceding history of the classical symptoms of diabetes. Ketoacidosis results in abdominal pain, nausea, and vomiting. The nausea and vomiting impair fluid intake, which combines with the ongoing glucosuria-driven osmotic diuresis to cause dehydration. The stress of the evolving physiological deterioration results in an increase in circulating levels of the counter-regulatory hormones glucagon, cortisol, growth hormone, and epinephrine. The greater the degree of insulin deficiency, along with the elevations in counter-regulatory hormone levels (especially glucagon), cause a greater rise in blood glucose level, as well as the production and accumulation of ketoacids. The acidosis stimulates the respiratory center, resulting in deep Kussmaul respirations; the patient may have a sense of respiratory distress. There is a further rise in the plasma glucose and accumulation of ketoacids in the blood as excretion of these compounds is impaired owing to a decrease in glomerular filtration with the developing dehydration. The resulting hyperosmolarity and acidosis may produce an impairment of consciousness that can progress from lethargy to coma.
The diagnostic criteria for diabetes are given in Table 11-2. In the presence of a history indicating polydipsia and polyuria, a plasma glucose concentration greater than 200 mg/dL confirms a diagnosis of diabetes.6 In a child that presents with nausea and vomiting, DKA should be considered if there is a preceding history of polydipsia and polyuria, particularly in the absence of supportive evidence of a viral illness, such as lack of sick contacts, diarrhea, and fever. Documentation of acidosis (venous pH < 7.3) and ketonemia or ketonuria confirm DKA.
When glucosuria or hyperglycemia are identified in an individual without symptoms of diabetes, the diagnosis of diabetes is based on an elevated plasma glucose level, that is, a fasting level greater than 125 mg/dL or a level more than 199 mg/dL at 2 hours during an OGTT. However, the vast majority of children are diagnosed with T1DM based on either their presentation in DKA or with unequivocal symptoms of hyperglycemia with a plasma glucose level above 199 mg/dL.
In certain clinical scenarios, a cause of diabetes other than T1DM should be considered. In adolescents, T2DM should be considered. In infants less than 12 months of age or in children with specific family histories of diabetes mellitus, genetic forms of diabetes should be considered. Stress hyperglycemia should be considered for children presenting with an acute illness but without a preceding history of polydipsia and polyuria. Later sections of this chapter discuss when to consider and how to evaluate for causes of diabetes other than T1DM.
Optimal treatment of T1DM requires the expertise of a number of health professionals, so that affected patients are best managed by an experienced diabetes team that can work together to coordinate the various aspects of care. This team is usually led by a pediatric endocrinologist or other physician with experience managing pediatric patients with diabetes, and ideally also includes a nurse educator, dietitian, and mental health professional. DKA should be treated in pediatric emergency departments or in hospitals with expertise in managing critically ill children.
The goals of treatment for children and youth with T1DM are to
Achieve as close to metabolic normalcy as possible with currently available technology
Avoid acute complications
Minimize the risk of long-term microvascular and macrovascular complications
Allow the child to achieve normal psychological maturity and independence and a normal lifestyle
To reach these goals the physician and the family will need to set glycemic goals for the child guided by American Diabetes Association (ADA) targets,7 and provide the patient and family with the educational resources and treatment options needed to reach this goal. Patients and their families should be seen by the diabetes team about every 3 months. At these appointments, it is important to review individual goals and glycemic control, discuss acute complications occurring since the last appointmentand strategies to avoid recurrence of these problems, and monitor for and treat complications and comorbidities.
One fundamental and important goal in diabetes care is independent self-management. Families who are well educated about diabetes and its management are equipped with the knowledge to make independent decisions about care. This ability can enhance independence and self-esteem. Day-to-day decisions about hypoglycemia, hyperglycemia, ketonuria, sick-days, erratic meals, and sports schedules are well handled by knowledgeable families.
Decisions about which insulin regimen to use should consider the needs, abilities, and resources of the child and the family. The meal plan should be designed using sound nutritional principles as well as consideration of the family’s food preferences and habits. Children with T1DM should be able to participate in any activities appropriate to their age and interest, and their diabetes regimen can be adapted to allow this flexibility.
Box 11-1 lists the circumstances in which a patient suspected of having T1DM should be referred to an emergency department or to a specialist.
Box 11-1. When to Refer
Diabetes Mellitus Type 1
All children with DKA should be immediately referred to an emergency department with expertise in managing DKA in the pediatric age group. However, if this is not locally available, initial referral can be to a nearby emergency room, with subsequent transfer to a facility with expertise in managing pediatric DKA.
All children with diabetes requiring insulin, including newly diagnosed diabetes, should be referred to and managed by a team experienced in the management of type 1 diabetes as soon as the diagnosis is made.
At the time of the diagnosis of diabetes, patients have some residual β-cells. Their function may improve with insulin treatment when the glucotoxic and lipotoxic effects on β-cells of the untreated diabetes are removed. Thus, there is often a temporary decrease in insulin requirements 1 to 3 months after diagnosis that is called the “honeymoon period” as noted previously. Insulin requirements may decrease to well below 0.5 U/kg/day. The honeymoon period may last several months and sometimes persists as long as 12 or more months. Over time, however, the majority of patients with T1DM will have no residual insulin production. Except during this honeymoon period, most prepubertal children need about 0.5 to 1.0 U/kg/day of insulin, with even lower doses in young children.8 Adolescents usually need insulin doses that are about 25% higher, or about 0.8 to 1.2 U/kg/day. This increased dose requirement is due to physiologic insulin resistance during puberty.
Recombinant DNA technology is used to manufacture all currently available types of insulin and is based on the amino acid sequence of human insulin. Insulin preparations are listed here by timing of action.
Rapid-acting analogs:
Lispro (Humalog, Eli Lilly)
Aspart (NovoLog, Novo-Nordisk)
Glulisine (Apidra, Sanofi-Aventis)
After subcutaneous injection, analogs are absorbed and cleared more rapidly than regular insulin. Thus, they more closely mimic the timing of pancreatic insulin secretion following food ingestion. They can be given after a meal in young children when food intake is unpredictable, although this may be associated with more postprandial hyperglycemia than if given at the start of the meal.
Short-acting insulin: regular (Humulin, R, Eli Lilly; Novolin R, Novo Nordisk)
Regular insulin is also used in intravenous infusions to treat DKA.
Intermediate-acting insulins:
Neutral protamine Hagedorn (NPH) (Humulin, N, Eli Lilly; Novolin N, Novo-Nordisk)
Detemir (Levemir, Novo-Nordisk)
NPH has intermediate timing for peak and duration of action. Detemir can be considered either intermediate or long acting. Its duration of action is dose-related.
Long-acting insulins:
Detemir (Levemir, Novo-Nordisk)
Glargine (Lantus, Sanofi-Aventis)
Glargine is peakless and has a duration of action of about 20 to 24 hours or more and can usually be given once per day. Detemir has a shorter duration of action than glargine and may require two injections per day. There may be less variability in the effect of detemir compared with that of glargine.
Insulin pharmacodynamics are summarized in Table 11-5 and Figure 11-5. With all the insulin formulations currently available, many options exist for insulin treatment regimens.
NPH-based or split/mixed regimens.
These are two or three injection regimens based on NPH, an intermediate-acting insulin. See Figure 11-6A and Table 11-6. Two injections of NPH are given per day: one in the morning shortly before breakfast and a second in the evening, either at dinner or at bedtime. Then either regular- or a rapid-acting analog is given at breakfast and dinner and sometimes at lunch. Absorption may vary from different injection sites and is more rapid in exercised sites and those at higher temperatures. Injection into hypertrophied sites may slow absorption.
These regimens may use NPH and regular insulins together at breakfast and again at dinner. The total daily dose is split into two injections and each is a mix of NPH and regular which may be given in the same syringe. With one variation the evening dose is divided so that regular insulin is given at dinner and NPH at bedtime. This allows the NPH to last until morning. The peak actions of insulin used in these NPH-based, split/mixed regimens do not correlate well with usual mealtimes or with nutrient absorption. Because of this there is significant between-meal insulin action and to counter this, specific mealtimes and meal amounts, including snacks, are needed to avoid hypoglycemia. The risk of nocturnal hypoglycemia appears to be greater with NPH and regular than with glargine or detemir and with rapid-acting analogs.9 Split/mixed regimens may use rapid-acting insulin in place of regular insulin. Like regular, rapid-acting insulins can be mixed with NPH in the same syringe and given as a single injection. The use of rapid-acting insulin decreases the problem of between-meal insulin peaks when regularis used. However, the peak with NPH occurs several hours after injections (see Table 11-5 and Figure 11-5) and can be variable. Thus, achieving glycemic targets without causing hypoglycemia using split/mixed regimens may be unachievable. Because of this, most patients should be treated with a basal/bolus regimen.
Traditional dose recommendations, when split/mixed regimens are used, are to give about two-thirds of the total daily dose in the morning and one-third in the evening. These doses usually are split between one-third regular/rapid-acting insulin and two-thirds NPH to closer to one-half/one-half (see Table 11-6). More regular/rapid-acting insulin may be required in the morning than at dinner. This may be because of the “dawn phenomenon,” a decrease in insulin sensitivity in the morning owing to normal nocturnal increases in some counter-regulatory hormones.
While most children are now using basal/bolus regimens, it is useful to know how to convert a patient from a two- or three-injection regimen with NPH to a basal/bolus regimen. Recommendations are to use 50% to 80% of the NPH dose for the initial basal insulin dose, with the lower percentages for younger children.
Basal/bolus regimens.
The goal of basal/bolus insulin regimens is to provide more physiological insulin levels so that peak levels coincide with nutrient absorption and basal levels occur between meals so that there is less between-meal insulin action. The basal insulin component provides baseline, between-meal, and fasting insulin needs. The bolus component provides insulin to cover food requirements and to correct hyperglycemia. The basal component may be provided by either rapid-acting insulin given by the basal rate using an insulin pump, or with once- or twice-daily injections of detemir or glargine. (Per labeling, unlike NPH insulin, detemir and glargine cannot be mixed with any other insulin, requiring that they be given as separate injections.) The bolus insulin is provided by rapid-acting insulin which may be given by injections or by the bolus function through an insulin pump. Figure 11-6A shows a diagram of a basal/bolus regimen.
Morning (before breakfast): 16 NPH | ||
Before dinner or at bedtime: 6 NPH | ||
Sliding scale regular or rapid-acting dose: | ||
Blood Glucose | Breakfast | Dinner |
< 50 | 6 | 4 |
50-100 | 7 | 5 |
100-150 | 8 | 6 |
150-200 | 9 | 7 |
200-250 | 10 | 8 |
250-300 | 11 | 9 |
> 300 | 12 | 10 |
Figure 11-6
Three insulin regimens. For each panel, the y-axis shows the amount of insulin effect and the x-axis shows the time of day. B, breakfast; CSII, continuous subcutaneous insulin infusion; HS, bedtime; L, lunch; S, supper. An asterisk indicates that either insulin lispro or aspart can be used. The time of insulin injection is shown with a vertical arrow. The type of insulin is noted above each insulin curve. (A) The injection of two shots of intermediate-acting insulin (NPH or Lente) and short-acting insulin (insulin lispro, aspart, or regular insulin) Only one formulation of short-acting insulin is used. (B) A multiple-component insulin regimen consisting of one shot of glargine at bedtime to provide basal insulin coverage and three shots of insulin lispro or aspart to provide glycemic coverage for each meal. (C) Insulin administration by insulin infusion device is shown with the basal insulin and a bolus injection at each meal. The basal insulin rate is decreased during the evening and increased slightly prior to the patient awakening in the morning. (A and C: Reproduced with permission from Farkas-Hirsch R, ed. Intensive Diabetes Management. 2nd ed. Alexandria, VA: American Diabetes Association; 1998.)
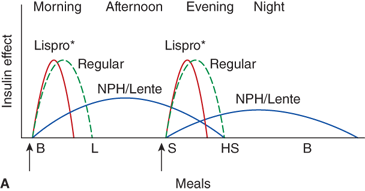
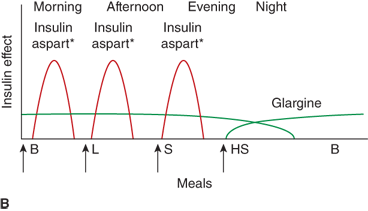
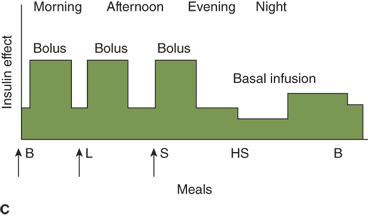
Estimated dose calculations are shown in Table 11-7. These calculations are based on clinically determined empiric formulas. Modifications to initial calculations will often need to be made once individual patient responses to starting doses are assessed. Usual basal requirements are about 30% to 50% of total daily insulin requirements, with younger children often requiring the lower proportion of daily insulin as basal. Bolus doses have two parts: first, the dose of insulin needed to cover the amount of carbohydrate grams in the meal or snack, and second, the dose of insulin needed to correct for blood glucose levels which are outside of the individual’s blood glucose target range. The insulinto carbohydrate ratio is the amount of insulin needed to cover each gram of ingested carbohydrate, specified as 1 unit per each X carbohydrate grams (eg, 1 unit per10 carbohydrate grams). The correction or sensitivity factor refers to the amount that an individual patient’s blood glucose will decrease when given 1 unit of insulin. In some individuals, insulin sensitivity may differ significantly at different times of the day. Such individuals may require different insulin to carbohydrate ratios and correction factors at different times of the day.
Total daily insulin dose = 32 U (0.8 U/kg) |
Basal insulin = 50% of total daily dose = 16 units given as either 16 units glargine per day OR 0.6 units per hour of rapid-acting insulin (lispro, aspart, or glulisine) as pump basal rate. |
Bolus doses for food: insulin to carbohydrate ratio for meals and snacks determined by the 450-500 rule: |
450-500 divided by total daily insulin dose (450-500/32 = 14-15). Use 1 unit per 15 carbohydrate grams to start. |
Bolus doses for high glucose correction: determined by 1800 rule: |
1800 divided by total insulin dose (1800/32 = 56). Use 1 unit per 56 (round to 60) blood glucose points (or 0.5 unit per 30 blood glucose points) above target. |
Target blood glucose: generally 120 in the daytime and 150 at night, usually somewhat higher for young children and those with hypoglycemia unawareness. |
The amount of the premeal bolus dose, therefore, is the sum of: first, the insulin to carbohydrate ratio multiplied by (planned or ingested) grams of carbohydrate and second, the correction or sensitivity factor multiplied by the amount that the blood glucose needs to fall from the blood glucose level determined preprandially to achieve the target range. Age-dependent target ranges are discussed below.
A disadvantage of glargine or detemir for basal insulin compared to insulin pumps is that pumps offer flexibility in basal rate (Figure 11-6C). With pumps, the basal rate can be set differently at different times of day. Pumps can also give insulin doses in smaller fractions than the 0.5-unit increments given by injections. Finally, pumps allow administration of insulin with snacks and for blood glucose levels above target range without additional injections. Children using an injection-based basal/bolus regimen may resist additional needle sticks. These advantages may result in a small improvement in overall glycemic control using a pump compared with injections.
Combining long-acting insulin by subcutaneous injections with insulin pump use can be done. This regimen replaces most of the pump basal rate with long-acting insulin given by a subcutaneous injection. This plan can be used to allow pump users to disconnect from the pump for several hours for swimming or for sports, for example. In addition, this approach can alleviate some of the variability in insulin action that can happen between the first and the last day of an insulin infusion site, and provide protection against DKA in the event of undetected pump failure.
It is important for children and adolescents with TDM to have a nutritionally balanced diet with adequate calories and nutrients for normal growth. Recommendations usually include about 50% to 55% as carbohydrate calories, 20% protein, and approximately 30% fat. The majority of carbohydrate calories should be complex carbohydrates, and the fat component should be “heart healthy” emphasizing low levels of cholesterol and saturated fats. For patients who are using NPH-based split/mixed insulin regimens, constancy in the timing and the carbohydrate amount of meals is important as this will help minimize blood glucose variability. In addition to the usual three meals, midafternoon snacks are necessary. This is because the timing of this snack coincides with the typical peak of the patient’s morning NPH insulin dose and with most after-school sports activities. Bedtime snacks are important for most children receiving evening NPH doses and help avoid nocturnal hypoglycemia. Midmorning snacks are useful in preschool-aged children. However, school-aged children often find that midmorning snacks are disruptive to their school schedule. Midmorning snacks are usually not recommended after a child begins elementary school. The child’s meal plan should allow occasional treats. Patients and their families can learn how to adjust insulin doses to accommodate times of increased caloric intake, such as holidays and birthdays. For patients using basal/bolus regimens near-total flexibility in timing, amount, and content of meals is possible. Overweight and obese patients will require calorie control. Meal plans that fit with the child and family’s food preferences and that provide satiety are necessary for realistic adherence to the nutritional recommendations. Clearly meal plans need to be individualized for each child and family.
An increased frequency of eating disorders, particularly in adolescent girls with T1DM has been reported at some centers. When this occurs, metabolic consequences can be devastating. Serious intervention, such as inpatient admission to an eating disorder unit, is indicated.
Nutritional management is complex, and is best guided by regular evaluations with a nutritionist who has expertise in diabetes management. This becomes especially important when using sophisticated regimens based on carbohydrate counting.
While all patients with T1DM require treatment with insulin, this therapy currently remains imperfect: even with excellent glycemic control, blood glucose levels are not normalized. Much of this is due to the pharmacodynamics of current insulin treatment. However, it is possible that additional improvements can be achieved with adjunct medications.10 Pramlintide, α-glucosidase inhibitors, and metformin have all been investigated as such medications.
Pramlintide.
Pramlintide is a synthetic analogue of amylin produced by recombinant DNA technology. Amylin is a hormone that is cosecreted with insulin in response to meals. It inhibits glucagon release and slows gastric emptying. In T1DM, amylin secretion, as well as insulin secretion, is deficient, suggesting that amylin replacement has a role in treatment. Pramlintide is given as a subcutaneous injection immediately before meals. It results in a decrease in postprandial hyperglycemia, a small decrease in HbA1c, and less weight gain than with standard insulin-only therapy, and is approved by the FDA as an adjunct to mealtime insulin treatment in adults with T1DM or T2DM; it is not currently approved for use in children. The side effects of pramlintide include hypoglycemia, nausea, and vomiting. These side effects are significant because delayed gastric emptying and nausea can complicate treatment of hypoglycemia. No clinical trials of pramlintide in the treatment of diabetes in children have been reported, so that additional clinical experience in children is needed before this insulin can be recommended for routine use in pediatric patients.
α-Glucosidase inhibitors.
α-Glucosidase inhibitors are oral agents that act within the gastrointestinal tract to delay the digestion and subsequent absorption of complexcarbohydrates. They are not absorbed, but can result in gastrointestinal side effects if nonabsorbed carbohydrate reaches the colon. In trials in adults with T1DM, acarbose decreased postprandial glucose excursions, but did not significantly lower HbA1c. The need to take this medication with meals and the gastrointestinal side effects, with marginal effects on glycemic control, makes this an unlikely agent to find significant use in pediatric patients.
3. Metformin.
Metformin is an insulin-sensitizing drug that improves glycemic control in patients with T2DM. Its main effect is to decrease hepatic glucose production, thus reducing fasting glucose levels. Use of metformin in adult patients with T1DM decreases daily insulin doses, as expected for an insulin sensitizer. Little or no decrease in HbA1c occurs, with an increase in hypoglycemia episodes. Additional data are needed to determine whether metformin has a role in treating children with T1DM.
Physical fitness and regular exercise are to be encouraged in all children with T1DM. Metabolic control, self-esteem, and body image may be better in the physically fit child. Exercise in a child with diabetes, however, requires specific attention. During periods of exercise, extra calories or lower insulin doses may be needed to prevent hypoglycemia.11 Some patients have a delayed hypoglycemic response to exercise, sometimes hours later. Blood glucose monitoring to assess the effects of exercise and the response to interventions on blood glucose levels should be done to determine an effective regimen for the individual child. The stress of exercise when metabolic control is poor (eg, during hyperglycemia, especially with ketosis) may worsen metabolic control through the release of stress hormones, so that it may be necessary to delay exercise at these times.
Self-monitoring of blood glucose is fundamental to diabetes management. More frequent monitoring has been shown to correlate with improved glycemic control. Glucose meters are small, portable, and accurate, and many have memory storage of several hundred readings. Some meters allow patients to record carbohydrate amounts and insulin doses. The ability to download the information to computers with specific software programs and to organize the data in different formats is available and can be very helpful in achieving good metabolic control. Some meters can communicate the glucose level to an insulin pump.
Blood glucose traditionally is monitored before meals, at bedtime, and overnight. Some children also monitor before snacks, especially those receiving bolus insulin with all carbohydrate intake. Basal/bolus regimens require a blood glucose level each time one ingests any calories so that accurate dose decisions can be made. Postprandial levels can be helpful in assessing whether the selected dose was satisfactory. Many people monitor at least 8 to 10 times per day, more often with sports, when ill, or during periods of metabolic instability. Reasonable targets are fasting and preprandial blood glucose readings from 100 to 180 mg/dL for preschool-aged children, 90 to 180 mg/dL for school-aged children, and 90 to 130 mg/dL for teenagers, with 75% of blood glucose levels in these ranges an appropriate goal. Bedtime or overnight levels of 110 to 200 mg/dL in preschoolers, 100 to 180 mg/dL in school-aged children, and 90 to 150 mg/dL in teenagers are also reasonable.
Continuous glucose sensors are available that read interstitial glucose levels and send the readings every few minutes to a display. These devices also display rate and direction of change and have alarms which can be set to alert for low or high glucose levels or impending levels in these ranges. While these devices have been shown to modestly improve glycemic control in adults, such improvement has not been demonstrated in children, in part due to declining use of the sensors over time.12,13
HbA1c is produced through the nonenzymatic glycation of hemoglobin. The rate of production of HbA1c is proportional to the plasma glucose concentration. Thus, because of the lifespan of red blood cells, HbA1c is an objective measure of the average blood glucose concentration over approximately the previous 2 months. The HbA1c should be measured regularly, usually every 3 months in patients with T1DM. While the ideal goal is to achieve a HbA1c level as close to normal as possible (4.5%-6.1%), goals that most patients should achieve vary with age: for toddlers and preschoolers (under 6 years) 7.5% to 8.5%; for school-aged children (6-12)less than 8%; and for adolescents and young adults (13-19) less than 7.5%. However, hypoglycemia may limit the ability to achieve these goals. Indeed, with the limitations of current insulins and methods of administration, reaching these target levels is often not achievable, especially in adolescents. The high risk and vulnerabilityof young children to hypoglycemia are why bloodglucose and HbA1c goals are higher for younger children. In rare situations, a measure of average glycemia other than HbA1c is useful. This most often is in cases of abnormal hemoglobins or abnormal red blood cell production or survival. Glycated albumin is proportional to the average plasma glucose over the preceding 1 to 2 weeks; fructosamine is a measure of glycated serum proteins (predominantly albumin) and is proportional to the average plasma glucose over the preceding 1 to 3 weeks.
Urine or blood ketones also should be monitored when the blood glucose levels are elevated (eg, above 250-300 mg/dL), when children have a fever, when they feel nauseous or are vomiting, or when they are not feeling well. This monitoring is important in achieving the goal of aborting DKA episodes by treating early ketosis.
Living with and managing diabetes is complex and demanding and requires initial and ongoing, lifelong education. Patients and families need to understand all aspects of diabetes, including acute and long-term complications. They must understand details of insulin action, including duration and timing and dose adjustments, injection and insertion techniques, electronics and mechanics of insulin pumps, dietary information including carbohydrate counting, blood glucose monitoring and interpretation, and urine or blood ketone testing and appropriate interventions. They must gain skills in integrating the demanding clinical regimen into their schedules so that they can achieve emotional stability and ongoing psychological growth.
Education must be appropriate to the child’s age and the family’s educational background. Shifting responsibility from parent to child for diabetes self-care skills (eg, insulin injections) should be done gradually and when the child shows interest and readiness to do so. Premature shifting of responsibility may be a cause of deterioration in metabolic control. Management of diabetes involves the whole family even as responsibility is shifted primarily to the adolescent. The life of the entire family is affected by having a child with T1DM. Sharing responsibilities and attending support groups and camps for children with T1DM can help with psychological adjustment.
Teaching about diabetes management is best handled by a diabetes management team, including a physician, nurse educator, dietitian, and mental health professional. Excellent comprehensive educational manuals for children and families are available and several comprehensive websites are exceptional (see selected resources at the end of this part).
A major goal of treatment in T1DM is to avoid both acute and chronic complications. DKA and hypoglycemia are the most significant acute complications of diabetes and its treatment—both with a significant risk of morbidity and mortality. Diabetes mellitus leads to damage to the microvascular circulation that results in tissue and organ damage, most notably in the retina, kidneys, and nerves. Because of these microvascular complications, diabetes mellitus is a leading cause of blindness, end-stage renal disease, and neuropathy. There is also a significant increase in the risk of atherosclerotic vascular disease in individuals with diabetes. This macrovascular disease results in an increased risk for strokes and heart attacks which are the most common cause of death in patients with diabetes.
Recombinant insulin analogs, insulin pumps, and newer devices for home monitoring have markedly improved our ability to control glucose levels in patients with diabetes. However, the feedback control in the healthy state that allows minute-to-minute regulation of insulin secretion cannot be recapitulated with current diabetes therapies, so that full metabolic normalization is not yet possible. Thus, some degree of hyperglycemia persists in virtually all patients with diabetes. Long-term complications, including renal failure, retinopathy, neuropathy, and cardiovascular disease are related to and likely caused by the hyperglycemia.
It is now firmly established that both the microvascular and macrovascular complications of diabetes are related to the hyperglycemia that persists even with treatment of the disease.14, 15, 16, and 17 The development of chronic complications is also dependent on the duration of diabetes, generally taking decades for clinically significant complications to appear. Therefore, while some late adolescents with early onset of diabetes may show early evidence of complications (eg, nonproliferative retinopathy, microalbuminuria [urinary albumin excretion of 30-300 mg/day], or changes in nerve conduction), it is extremely uncommon for a child to have significant diabetic microvascular or macrovascular complications. Nonetheless, it is necessary to maximize glycemic control in children with diabetes to minimize their risk of future long-term complications.
Clinical trials, including the Diabetes Control and Complications Trial (DCCT) demonstrated that the lower the hemoglobin A1c (HbA1c) that a patient maintains, reflecting a lower average blood glucose level, the lower the risk of microvascular complications. Recent data from the DCCT indicate that the HbA1c level explains nearly all the difference in complication risk in this study. An improvement in HbA1c of 1% (reflecting a decrease in mean glucose levels of 30-35 mg/dL) decreases the risk of long-term complications by approximately 20% to 50%. There was no threshold for this effect—that is, a lower HbA1c is always better in terms of lowering the risk of long-term complications. However, the absolute risk reduction is less at lower HbA1c levels, and lower average glucose levels may increase the risk of the acute complication of hypoglycemia. Therefore, diabetes management involves balancing the long-term benefit of lowering the average glucose level with avoiding the acute complication of hypoglycemia.
In order to prevent hypoglycemia, the normal response to successively lower plasma glucose concentrations in a nondiabetic individual is: first, a decrease and then cessation of insulin secretion; next, the secretion of counter-regulatory hormones—initially glucagon and subsequently epinephrine, growth hormone, and cortisol. In T1DM, where insulin levels come from absorption of exogenously administered insulin, there is noability to decrease insulin levels in response to hypoglycemia. In addition, there is impaired glucagon secretion in patients with T1DM. The defect in glucagon deficiency is relatively modest in the early years after diagnosis, but becomes more significant 10 years after diagnosis. Although not generally present in children, patients who develop autonomic neuropathy may also have impaired secretion of epinephrine, cortisol, and growth hormone.
Hypoglycemia, a blood glucose level less than 60 mg/dL, is a frequent occurrence in children treated for T1DM. It is caused by loss of the normal responses that protect against hypoglycemia as noted above. Most notable is the inability to perfectly match the minute-to-minute changes in insulin requirements with current therapy, resulting in periods when insulin action exceeds insulin requirements. Patients with lower average blood glucose levels may have more frequent episodes of hypoglycemia. The severity of symptoms of hypoglycemia depends on both the degree of hypoglycemia, as well as the rapidity of its development. The adrenergic symptoms of hypoglycemia include sweating, trembling, hunger, and palpitations; the neuroglycopenic symptoms include headache, lightheadedness, dizziness, diplopia, and confusion. With severe hypoglycemia seizures and even coma can occur.
Mild to moderate hypoglycemia is treated by ingesting 10 to 15 g of glucose (eg, 4 oz of juice or nondiet soft drink). Hypoglycemia in infants and young children, and moderate reactions resulting in confusion in older children, require that caregivers, including teachers and coaches, and the like, be prepared to assist in the recognition and treatment of hypoglycemia. Severe reactions require treatment with intramuscular or subcutaneous glucagon (1 mg, except for children less than 20 kg, where 0.5 mg [or 20-30 μg/kg] is given). Because hypoglycemia can occur away from home, a source of glucose to treat it (eg, a tube of cake frosting) and a glucagon emergency kit should always be available.
Hypoglycemia unawareness is defined as having blood glucose values less than 65 mg/dL without the adrenergic symptoms of hypoglycemia caused by decreased epinephrine and/or glucagon output. This may result in altered cognition without self-identified symptoms of hypoglycemia. Hypoglycemia unawareness is an important cause of severe hypoglycemia (36% of the hypoglycemia during the DCCT occurred while subjects were awake). Since the blood glucose threshold for adrenergic release will vary with the recent level of blood glucose control (higher blood glucose values are associated with a higher blood glucose threshold), one hypoglycemic event can lead to a significant decrease in counter-regulatory responses and cause an unawareness of hypoglycemia for the subsequent 24 hours leading to a “snow-ball” effect with increasing frequency of hypoglycemia. Additionally, moderate exercise may result in a decrease in symptoms of hypoglycemia caused by decreased adrenergic release the next day. The blood glucose threshold is also reduced during sleep.
Since hypoglycemia unawareness is a strong predictor for a severe hypoglycemia event, a detailed history of the glucose threshold for hypoglycemia symptoms should be obtained at each visit. Patients and families should be counseled to contact a diabetes provider if hypoglycemia unawareness develops. There is evidence that loss of awareness of hypoglycemia can be reversed by avoiding hypoglycemia for 2 to 3 weeks.18 This is accomplished by setting higher blood glucose targets during this time, decreasing the insulin dose, and increasing the frequency of blood glucose testing, including some middle of the night tests.
Catabolism of fatty acids results in production of the ketone bodies acetoacetate, β-hydroxybutyrate, andacetone. The presence of urine or blood ketones is an indication of significant insulin deficiency. Urine ketones should never be measurable and blood ketones should not be elevated in a patient with T1DM. Whenever persistent, significant hyperglycemia occurs (eg, bloodglucose > 250 mg/dL in spite of corrective doses of insulin being given), urine or blood ketones should be tested. Urine or blood ketones should also be tested whenever the child feels ill, particularly with nausea and vomiting. Aggressive treatment with additional insulin is necessary once ketosis develops in order to prevent deterioration to ketoacidosis. In a patient whose diabetes is managed with an insulin pump, insulin doses to correct persistent hyperglycemia or ketosis should be given by injection with needle and syringe, as pump malfunction is a possible cause of the insulin deficiency. Rapid-actinginsulin at doses of 10% to 20% of the total daily requirement should be given every 3 to 4 hours until the ketones are cleared. In a child who is not able to take sufficient caloric intake because of illness, care must be given to avoid causing hypoglycemia.
Management of diabetes during even simpleillnesses can be challenging, more so in illnesses that interrupt oral intake. While uncertain oral intake puts the patient at risk of insulin-induced hypoglycemia, insulin treatment must be continued to prevent deterioration to DKA; indeed, total insulin requirements may increase during illness because of the insulin-antagonizing effects of inflammation and stress hormones. Management of diabetes during an illness will usually require guidance from the patient’s diabetes team. Basal insulin should be continued, generally at the usual dose, but sometimes at a slightly decreased dose based on blood glucose levels. Blood glucose and ketone measurements should be performed frequently (at least every 3-4 hours). Extra fluids are given to maintain hydration, which also helps excrete excess glucose and ketoacids. If solid foods cannot be eaten, sugar-containing liquids such as soda, juice, jelly, and popsicles can be given to maintain some caloric intake to prevent hypoglycemia. During some illnesses, the usual daily insulin doses, adjusted for intake and glucose levels, can be continued. For illnesses where oral intake is more disrupted, when ketones are present, or for more significant illnesses, it may be best to treat with more frequent, small doses of insulin; typical doses may be 5% to 10% of the total daily dose every 3 to 4 hours, increasing to 10% to 20% of the total daily dose every 3 to 4 hours if ketones are present. Persistent vomiting, or a refusal or inability to take fluids or food orally, requires an emergency department or office visit.
Glucagon must be available to treat hypoglycemia during an illness. The dose of glucagon to maintain glucose values within the normal range or treat mild hypoglycemia owing to poor intake is lower than that required to treat severe hypoglycemia19: 10 μg/year of age (minimum 20 μg, maximum 150 μg); if there is no response at 30 minutes, a repeat dose at twice the initial dose can be attempted. The usual dose (1 mg for weight >20 kg; 0.5 mg for weight ≤ 20 kg) is given for severe hypoglycemia. The higher doses of glucagon frequently cause significant nausea and vomiting, further compromising the ability to ingest food, so should be avoided if possible when treating milder hypoglycemia associated with poor oral intake.
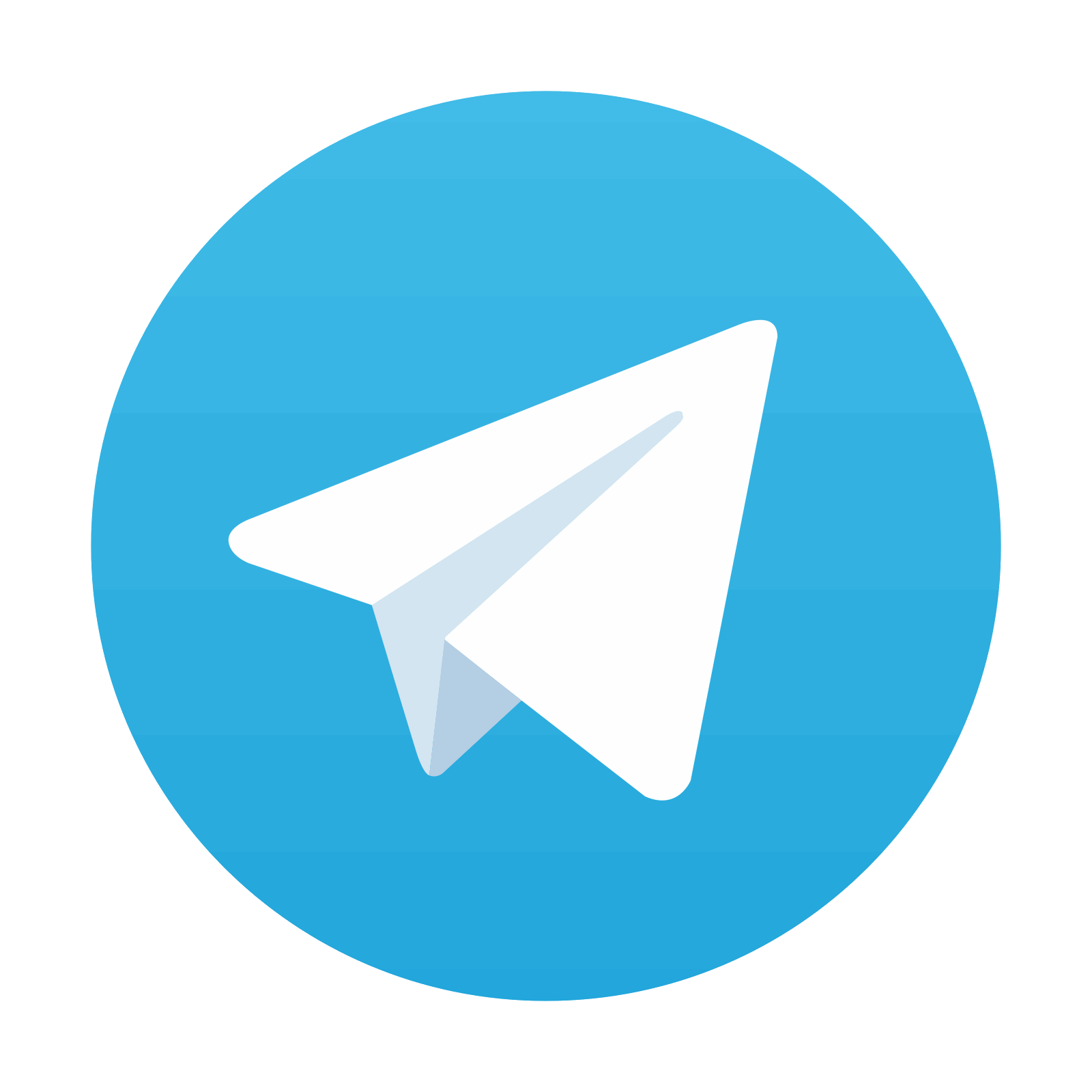
Stay updated, free articles. Join our Telegram channel

Full access? Get Clinical Tree
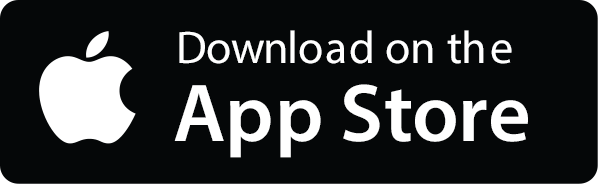
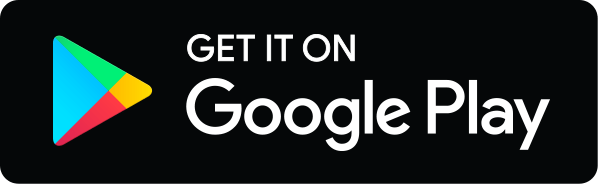