Thyroid hormone is a potent regulator of metabolic rate and is essential to the function of most organ systems. In addition, the maintenance of normal thyroid status through childhood is necessary for normal growth and neurodevelopment. Best outcome in pediatric thyroid disease requires early detection as well as appropriate therapy with careful monitoring. These tasks are generally accomplished through successful collaboration between primary care providers and endocrine specialists. With an appropriate index of suspicion, pediatric thyroid disease can be detected in early stages and outcome is usually excellent. This chapter begins with a review of thyroid physiology and clinical testing. We then present diagnostic approaches to common pediatric thyroid disorders, grouped into the categories of hypothyroidism, thyrotoxicosis, and nodular thyroid disease.
The fetal thyroid begins as a thickening of the pharyngeal floor which forms a diverticulum that descends caudally into the resting position of the mature thyroid gland. During this migration, a track called the thyroglossal duct is formed that connects the pharyngeal floor to the thyroid bed. This thyroglossal duct normally involutes and, by embryonic day 50, the thyroid primordium has fused with the ventral aspect of the fourth pharyngeal pouch to form a bilobed structure. The cells of this primordium differentiate into thyroid follicular cells that synthesize thyroid hormone. Thyroid C cells, which produce calcitonin, have a distinct embryologic origin and are derived from the ultimobranchial glands.
By 11 to 12 weeks of gestation, the fetal thyroid is capable of concentrating iodine and synthesizing thyroxine (T4). Prior to this point, the human fetus is dependent on maternal thyroid hormone. Even in later pregnancy, transplacental passage remains an important source of fetal thyroid hormone, as evidenced by the fact that T4 is detectable in the serum of infants born with complete thyroid agenesis.
Analyses of serum obtained through cordocentesis indicate that the fetal relationship of thyrotropin (also called thyroid-stimulating hormone [TSH]) to T4 matures throughout gestation. Compared to maternal serum, fetal serum has low concentrations of both thyroxine (T4) and triiodothyronine (T3). Birth is associated with a robust but transient peak in both serum TSH and T4 (referred to as the neonatal surge), followed by rapid changes in the metabolism of thyroid hormone in peripheral tissues. This normal neonatal thyroid surge typically lasts 1 to2 days, which is the rationale for delaying newborn screening until 2 days after birth.
From a clinical standpoint, deviation from the normal anatomic development of the thyroid (termed thyroid dysgenesis) is the most common cause of congenital hypothyroidism. As described in the following text, the study of patients with thyroid dysgenesis has provided valuable insight into the molecular biology of normal thyroid development.
The sole physiologic function of the thyroid gland is to synthesize T4 and T3. Dietary iodine is a critical nutritional requirement for the formation of both these thyroid hormones. Endemic cretinism is a term that describes the clinical consequence of dietary iodine deficiency, which is characterized by thyroid enlargement and neurodevelopmental retardation from thyroid hormone insufficiency. The pathophysiology of endemic cretinism is fully prevented by supplementation of dietary iodine.
Iodine is absorbed from the small intestine via a TSH-independent mechanism. Once in the circulation, iodine is transported into thyroid follicular cells via the sodium-iodine symporter, oxidized, and organified onto tyrosyl residues of thyroglobulin, a large glycoprotein that resides in thyroid colloid (Figure 4-1). These iodinated tyrosyl residues are coupled to form T4 and T3, which are eventually released into the circulation after proteolysis of thyroglobulin. Each step in the synthesis of thyroid hormone is catalyzed by enzymes that, when deficient, may lead to goiter and/or hypothyroidism. Autosomal recessive mutations in genes that encode these enzymes or their cofactors have been shown to cause dyshormonogenic congenital hypothyroidism.
The hypothalamus produces thyrotropin-releasing hormone (TRH), which stimulates pituitary thyrotrophs to secrete TSH, which in turn stimulates the thyroid to secrete thyroid hormone. Both thyroid hormones, T4 and T3, exert negative feedback on the hypothalamus and pituitary (Figure 4-2). Because the inverse relationship between free T4 and TSH is log-linear, small changes in serum T4 produce large compensatory changes in serum TSH (Figure 4-3). For this reason, serum TSH is the most sensitive test for the diagnosis of primary thyroid dysfunction. Laboratory results that consistently document deviation from the normal free T4 to TSH relationship warrant an investigation for central thyroid disease due to an abnormality of the hypothalamus and/or pituitary (Table 4-1).
Serum TSH | Serum-Free T4(or Free T4 Index) | Serum-Free T3(or Free T3 Index) | |
---|---|---|---|
Primary hypothyroidism | High | Normal or low | Normal or low |
Central hypothyroidism | Low/normal/high | Low | Normal or low |
Consumptive hypothyroidism | High | Normal or low | Normal or low |
Resistance to thyroid hormone | Normal/high | High | High |
TSH-secreting pituitary adenoma | Normal/high | High | High |
Hyperthyroidism | Low | Normal or high | Normal or high |
Thyrotoxicosis without hyperthyroidism | Low | Normal or high | Normal or high |
Figure 4-2
Hypothalamic-pituitary-thyroid axis. The hypothalamus and pituitary stimulate thyroid hormone secretion, primarily in the form of the prohormone T4, which is then converted to T3 by outer-ring deiodination in peripheral tissues. Both T4 and T3 exert negative feedback on the hypothalamus and pituitary.

Figure 4-3
Log-linear relationship between serum TSH and free T4. Small changes in serum T4 produce large deviations in serum TSH. (Redrawn with permission from: Larsen P, Davies T, Schlumberger M, Hay I. Thyroid physiology and diagnostic evaluation of patients with thyroid disorders. In: Kronenberg HM, Melmed S, Larsen PR, Polonsky KS, eds. Williams Textbook of Endocrinology, 12th ed. Philadelphia, PA: Saunders/Elsevier; 2011.)

The effects of thyroid hormone are mediated by the binding of T4 and T3 to thyroid hormone receptors (TRs) in the nuclei of target cells (Figure 4-4). TRs are encoded by two distinct genes, THRA and THRB, which encode several distinct receptors via alternative splicing. Three of these TR isoforms are functional (TRα1, TRβ1, and TRβ2), and each has a unique tissue distribution. Binding of T4 or T3 to a functional TR leads to the formation of a transcriptional complex that stimulates or represses the expression of target genes.
Figure 4-4
Thyroid hormone signaling. Circulating T4 and T3 enter target cells through specific transporters and are activated or inactivated by type 1 (D1), type 2 (D2), and type 3 (D3) deiodinases. Nuclear T3 then binds the thyroid hormone receptor (TR) and additional cofactors to form a transcriptional complex that stimulates or represses the expression of thyroid hormone responsive genes.

Though both T4 and T3 can bind TRs and signal thyroid hormone action, T3 is more potent because it binds TRs with 15-fold greater affinity than T4. Most thyroid hormone is secreted from the thyroid gland in the form of T4, which is subsequently converted into the more active T3 by enzymatic outer-ring deiodination in peripheral tissues (Figure 4-5). Because of this peripheral activation, T4 is often referred to as a prohormone.
Figure 4-5
Thyroid hormone deiodination. Outer-ring deiodination (removal of a 5′ position iodine), catalyzed by D1 and D2, activates the T4 prohormone into T3. Inner-ring deiodination (removal of a 5′ position iodine), primarily catalyzed by D3, inactivates T4 and T3. (Redrawn and modified from: Larsen P, Davies T, Schlumberger M, Hay I. Thyroid physiology and diagnostic evaluation of patients with thyroid disorders. In: Kronenberg HM, Melmed S, Larsen PR, Polonsky KS, eds. Williams Textbook of Endocrinology, 12th ed. Philadelphia, PA: Saunders/Elsevier; 2011.)

The main pathway of thyroid hormone metabolism is sequential deiodination, which is catalyzed by a family of enzymes called the deiodinases. The three members of this family include designated type 1 (D1), type 2 (D2), and type 3 (D3) deiodinase. Each deiodinase is encoded by a distinct gene and has unique enzyme kinetics and tissue distribution. D1 and D2 remove a single iodine from the outer ring of T4, converting it to the more active T3. Together, D1 and D2 activity produce 80% of circulating T3, which explains why hypothyroid patients treated with T4 alone have normal serum levels of T3. Conversely, inner-ring deiodination of either T4 or T3 produces the inactive metabolites reverse T3 (rT3) and T2, respectively. D3 is the major inactivating enzyme for both T4 and T3 (see Figure 4-5).
The vast majority of thyroid hormone in the circulation is bound to serum proteins, including thyroxine-binding globulin (TBG), transthyretin, and albumin. In euthyroid individuals, only 0.02% of T4 and 0.3% of T3 is unbound or “free” and immediately available to enter cells and mediate thyroid hormone signaling. An understanding of this is helpful in the interpretation of serum thyroid function tests because individuals with normal thyroid status (and normal free thyroid hormone concentrations) but abnormal binding proteins can have deranged serum measurements of total T4 and/or total T3. Recognizing this is important to avoid the false diagnosis of thyroid disease in such individuals (see Table 4-1).
Whereas many tests are available to evaluate thyroid disorders, most patients can be accurately diagnosed by a focused history and physical examination, combined with standard serum thyroid tests (see Table 4-1). In a minority of thyrotoxic patients, quantification of thyroid radioiodine uptake (RAIU) can be useful to distinguish transient thyroiditis from early Graves hyperthyroidism. Anatomic imaging (such as sonography) can generally be reserved for patients with thyroid nodules.
The normal thyroid is located in the midline of the neck, between the thyroid cartilage and the suprasternal notch. Physical examination should begin with visual inspection of the neck for gross thyroid enlargement or asymmetry, followed by palpation of the gland. The examiner may palpate the thyroid with the fingertips while standing behind the patient, or may face the patient and palpate the thyroid with his or her thumbs (Figure 4-6A and B). In the authors’ opinion, the latter approach is more sensitive for detecting small nodules and offers the benefit of visual correlation during palpation. Because it is encased by pretracheal fascia, the thyroid moves with swallowing, a feature that is critical in distinguishing the gland from adjacent structures. Accordingly, the patient should be provided a cup of water and asked to sip intermittently while the examiner palpates the gland, beginning in the midline and moving laterally to define the lateral borders of the gland. Infants too small to stand or sit may be examined in the supine position. One hand should be placed under the infant’s shoulders and slowly raised until the neck falls gently into mild hyperextension. The anterior neck can then be palpated with the opposite hand (Figure 4-6A).
Thyroid size and the presence of any nodules should be noted. If a thyroid nodule is present, the cervical lymph node chains should be carefully palpated to assess the possibility of local metastasis from thyroid cancer. The normal thyroid grows during childhood and a helpful “rule of thumb” is to remember that each thyroid lobe should be about the size of the distal segment of the patient’s thumb. With practice, one should be able to palpate the normal thyroid gland of nearly all patients of school age or older.
Clinical testing to accurately measure serum TSH, T4, and T3 is widely available. Because variations in thyroid hormone–binding proteins can occur, when measuring total thyroid hormone concentrations it is important to include an index of hormone binding, such as thyroid hormone–binding ratio (also called T3 resin uptake). These values can then be used to calculate a free T4 index. Alternatively, free T4 and free T3 concentrations may be assayed directly. In general, the highly sensitive methods required to measure accurately the picomolar concentrations of free thyroid hormone exist only in reference laboratories. The less expensive free T4 assays used in most clinical laboratories indirectly estimate free T4 concentrations without physically separating free from bound hormone. These estimates are useful in many routine settings but may be inaccurate in patients with binding abnormalities or intercurrent nonthyroidal illness. Thus, if an initial free T4 measurement deviates from the clinical impression or indicates a very rare disorder such as central thyroid dysfunction, measuring free T4 by a gold standard method (direct dialysis or ultrafiltration) is justified to avoid extensive evaluations for potentially artifactual abnormalities. These issues are discussed in detail in consensus guidelines.1
As noted previously, serum TSH is the most sensitive single test to screen for primary thyroid disease (see Figure 4-3). If central thyroid disease is being considered, or if the index of suspicion for thyroid dysfunction is high, serum-free T4 (or free T4 index) should be added. Though adjunctive tests may be helpful after thyroid dysfunction is diagnosed, the combination of serum TSH and free T4 is a sufficient initial screen, as the combination of normal TSH and normal free T4 effectively rules out treatable forms of thyroid dysfunction. Of note, though Table 4-1 represents the vast majority of patients with thyroid dysfunction, two rare additions should be noted. Though patients with mutations in THRB show the classic pattern of resistance to thyroid hormone (RTH) (hyperthyroxinemia and hypertriiodothyronemia), rare patients with mutations in THRA display a different pattern of thyroid function tests characterized by high-normal T3, low-normal T4, and low-normal TSH.2, 3, and 4 This same pattern is seen in Allan-Herndon-Dudley syndrome, a rare X-linked disorder caused by loss-of-function mutations in SLC16A2, which encodes the neuronal T3 transporter MCT8. In clinical practice, both THRA and SLC16A2 mutations are associated with severe extrathyroidal dysmorphology (growth retardation, skeletal dysplasia, and constipation with THRA mutation; congenital hypotonia, intellectual disability, and limited motor and language skills with SLC16A2 mutation) so thyroid dysfunction is almost never the presenting feature of these conditions. However, awareness of these thyroid function patterns avoids the risk of misdiagnosingcentral thyroid disease in such individuals and risksof inappropriate treatment with thyroid hormone or antithyroid medications.
When interpreting thyroid tests, it is important to apply age-specific normal ranges (Table 4-2)5 and to be aware that certain medications, including amiodarone and common anticonvulsants (such as phenytoin and carbamazepine), can also confound the interpretation of serum thyroid tests by altering the normal serum T4:T3 ratio. During some stages of childhood the normal limits of free T4 extend outside the standard adult reference range. Failure to consider this can lead to incorrect diagnosis of central thyroid disease. Clinicians must also take into account preanalytic factors that may alter serum thyroid tests in the absence of true thyroid pathology. The most common of these is nonthyroidal illness, a term used to describe the pattern of transient derangement of serum thyroid function tests found in up to 75% of hospitalized patients in the absence of true thyroid disease. Though varies from one patient to another, this pattern is generally characterized by a fall in serum T3, followed by a fall in serum T4 and then serum TSH as the severity of illness progresses. As patients recover from their illness, serum TSH can transiently rebound above the normal reference range. Thus, many critically ill patients display abnormal serum thyroid function. As there is no available clinical test to reliably distinguish nonthyroidal illness from true thyroid disease, it is generally recommended that thyroid function tests be avoided in critically ill patients unless there is a specific suspicion for thyroid dysfunction. In addition, if mild thyroid function abnormalities are observed during illness, it is appropriate to defer treatment and monitor closely for resolution as recovery proceeds.
Age | Free T4 (ng/dL) | T4 (μg/dL) | Free T3 (pg/dL) | T3 (ng/dL) | TSH (mLU/L) |
---|---|---|---|---|---|
Cord blood | 0.9-2.2 | 7.4-13.0 | 15-75 | 1.0-17.4 | |
1-4 d | 2.2-5.3 | 14.0-28.4 | 180-760 | 100-740 | 1.0-39.0 |
2-20 wk | 0.9-2.3 | 7.2-15.7 | 185-770 | 105-245 | 1.7-9.1 |
5-24 mo | 0.8-1.8 | 7.2-15.7 | 215-720 | 105-269 | 0.8-8.2 |
2-7 y | 1.0-2.1 | 6.0-14.2 | 215-700 | 94-241 | 0.7-5.7 |
8-20 y | 0.8-1.9 | 4.7-12.4 | 230-650 | 80-210 | 0.7-5.7 |
21-45 y | 0.9-2.5 | 5.3-10.5 | 210-440 | 70-204 | 0.4-4.2 |
This test permits a direct assessment of thyroid function by quantifying the ability of an individual’s thyroid gland to concentrate iodine. Thyroid radioiodine uptake (RAIU) is calculated by measuring thyroidal radioactivity 4 or 24 hours after oral administration of radioactive iodine. Though both 131I and 123I can be used, 123I is preferred in children because it provides much lower radiation exposure. Because RAIU depends on dietary iodine intake, normal ranges vary among geographic regions. High RAIU values are characteristic of all hyperthyroid conditions, and this feature distinguishes hyperthyroid patients from those with thyrotoxicosis due to exogenous thyroid hormone or transient thyroiditis, in whom RAIU is low. Though RAIU can be low in certain forms of hypothyroidism, its measurement is not useful to diagnose hypothyroidism in iodine-sufficient populations (such as North America) because in this setting even euthyroid individuals can have RAIU values as low as 4%.
Images may be obtained with a pinhole gamma camera after oral 123I administration to define the location of functional thyroid tissue. This can identify sites of ectopic thyroid tissue or determine the functional status of thyroid nodules (Figure 4-7). Thyroid scintigraphy can also be used to detect iodine-avid metastases in patients with differentiated thyroid cancers of follicular cell origin (Figure 4-8). Like RAIU testing, thyroid scintigraphy is affected by dietary iodine intake, and high pharmacologic doses of iodine (from sources such as iodinated computed tomography [CT] contrast) can compromise 123I imaging.
Figure 4-7
Thyroid scintigraphy of cold versus hot nodules. Anterior views of two different patients with thyroid nodules are shown. Panel A shows a photopenic defect (marked by arrowheads) in a euthyroid patient with a right-sided thyroid nodule, demonstrating that the nodule is hypofunctioning or “cold.” Panel B shows focal 123I uptake into the large left-sided nodule of a hyperthyroid patient, demonstrating that the nodule is hyperfunctioning or “hot.” (Reproduced with modifications from: Huang SA. Thyroid. In: Treves ST, ed. Pediatric Nuclear Medicine/PET. 3rd ed. New York, NY: Springer; 2007:57-73, with kind permission of Springer Science and Business Media.)


Sonography of the thyroid is a noninvasive tool that can accurately quantify thyroid size. In the hands of an experienced user, it is also an ideal modality to confirm the presence of thyroid nodules and, when appropriate, to monitor the size of thyroid nodules over time. When fine-needle aspiration of the thyroid is performed, sonographic guidance is critical to optimizing both diagnostic accuracy and patient safety.
Hypothyroidism is defined as inadequate action of thyroid hormone at the tissue level. Hypothyroidism is the most common thyroid disorder, with an estimated population prevalence in childhood of 0.14% and a female to male ratio of 3:1.6 Autoimmune thyroiditis is the most common etiology.
Hypothyroidism can arise from secretory defects at any level of the hypothalamic-pituitary-thyroid axis (central or primary hypothyroidism), from decreased tissue responsiveness (RTH), or from accelerated degradation of circulating thyroid hormone at a rate that exceeds the synthetic capacity of the normal thyroid gland (consumptive hypothyroidism).
Common signs and symptoms of hypothyroidism are listed in Table 4-3. Of note, none is completely sensitive or specific for hypothyroidism, and symptom severity can vary greatly between patients with similar degrees of biochemical derangement. The clinician’s history should include inquiry into energy level, sleep pattern, cold intolerance, and weight gain. In addition to thyroid palpation, the physical examination should include an assessment of fluid status, muscle strength, heart rate, and deep tendon reflexes. Because autoimmune hypothyroidism can be the first presentation of an autoimmune polyglandular syndrome (see Chapter 5), signs and symptoms of possible coexisting autoimmune disorders such as diabetes mellitus and Addison disease should be sought.
Goiter Growth retardation Delayed skeletal maturation Abnormal pubertal development (delay or pseudoprecocity) Slowed mentation Fatigue Bradycardia (decreased cardiac output) Constipation Cold intolerance Hypothermia Fluid retention and weight gain (impaired renal free water clearance) Dry, sallow skin Delayed deep tendon reflexes |
Long-standing hypothyroidism causes growth retardation that disproportionately impairs linear growth more than weight gain. Prolonged elevation of TSH from untreated primary hypothyroidism can also cause pseudopubertal changes such as testicular enlargement, premature breast development, or premature menarche due to cross-reactivity of TSH with the follicle-stimulating hormone (FSH) receptor. These changes can be distinguished from true puberty by the absence of growth acceleration and by the fact that hypothyroidism causes delay rather than advancement in skeletal maturation.
Serum thyroid tests are sensitive for diagnosing hypothyroidism and sufficient for identifying the common subtypes (see Table 4-1). Growth data and the review of systems can provide insight into the duration of thyroid insufficiency.
Serum TSH and free T4 should be measured when hypothyroidism is suspected (see Table 4-1). Serum TSH is elevated in all patients with primary hypothyroidism; in more severe cases, serum-free T4 is low. If serum-free T4 is low with an inappropriately normal or low serum TSH, central hypothyroidism should be considered. Conversely, if serum-free T4 and/or free T3 are persistently high with an inappropriately normal or high TSH, the diagnosis of thyroid hormone resistance should be investigated. Measurement of serum T3 is not useful in the diagnosis of hypothyroidism because compensatory increases in thyroidal T3 secretion and peripheral T4-to-T3 conversion maintain normal serum T3 levels in many hypothyroid patients.
This section reviews the major causes of hypothyroidism in children. Though identifying the specific cause of a child’s hypothyroidism rarely changes initial therapy, this information is useful to predict the patient’s long-term course and to counsel parents appropriately.
Primary hypothyroidism refers to disorders of the thyroid gland itself that reduce its production of T4 and T3. As serum T4 and T3 fall, even within the normal range, loss of inhibitory feedback results in TSH hypersecretion. Elevation of serum TSH is the first abnormality detected in primary hypothyroidism. As the severity of primary hypothyroidism increases, serum T4 and then serum T3 fall below normal. Common causes of primary hypothyroidism are discussed in the following sections.
Autoimmunity is the most common cause of acquired hypothyroidism in both children and adults (Figures 4-9 and 4-10). Persistent hypothyroidism may be caused by two forms of chronic autoimmune thyroiditis: type 2A (goitrous, classic Hashimoto disease) and type 2B (nongoitrous, atrophic thyroiditis). Both are characterized by lymphocytic infiltration of the thyroid parenchyma, destruction of follicular thyroid cells, and high serum concentrations of thyroid autoantibodies. Documenting high serum titers of either thyroperoxidase antibodies or thyroglobulin antibodies is sufficient for diagnosing chronic autoimmune thyroiditis as the cause of a patient’s hypothyroidism. Thyroperoxidase antibodies are more sensitive and should be measured first, but thyroglobulin antibodies may be added if thyroperoxidase antibodies are not elevated. Approximately 95% of patients with autoimmune thyroiditis are positive for at least one of these antibodies.
Figure 4-10
(A) A 14-year-old juvenile-appearing boy with long-standing autoimmune hypothyroidism. He was as tall as the average 8.5-year-old and had a skeletal maturation of 7.5 years. (B) The boy’s general physically immature appearance. The 5th percentile of height for age is shown as the black line above his head. (C) The boy’s appearance after 3.5 years of replacement thyroid hormone treatment. The stadiometer is set at the 5th percentile of height for age. Note that before leuprolide treatment was available, many children with long-standing hypothyroidism would undergo rapid pubertal development and skeletal maturation once started on treatment and would fail to reach “expected” adult height, as was the case in this child.



The prevalence of autoimmune hypothyroidism increases with age, with a 3:1 female to male ratio.6 Presentation under 3 years of age is rare, but cases have been described even in infancy. Though the pathophysiology of thyroid autoimmunity is incompletely understood, there is a strong familial component. Individuals with Down syndrome, Klinefelter syndrome, and Turner syndrome are at increased risk.7
Upon diagnosis, families should be counseled that autoimmune hypothyroidism is usually lifelong, but that replacement therapy is effective. Children on levothyroxine treatment should be followed carefully to ensure consistent euthyroidism. Because autoimmune thyroiditis may be the initial presentation of an autoimmune polyglandular syndrome (see Chapter 5), the review of systems should include direct inquiry into symptoms concerning for other autoimmune endocrinopathies such as type 1 diabetes and Addison disease.
Approximately one in 3000 children is born with permanent hypothyroidism. Eighty-five percent of these cases are due to thyroid dysgenesis, defined as defective anatomic development of the gland with resultant agenesis, hypoplasia, or ectopy. Though mutations in transcription factors such as TITF1/NKX2-1 (formerly called TTF-1), FOXE1 (formerly called TTF-2), and PAX-8 that are required for normal thyroid development can cause thyroid dysgenesis in humans, such patients are extremely rare (representing only 2% of children with thyroid dysgenesis) and their congenital hypothyroidism typically arises in the context of broader phenotypic syndromes. For instance, mutations in TITF1/NKX2-1 cause choreoathetosis, respiratory distress, and variabledegrees of thyroid deficiency, whereas mutations in FOXE1 cause Bamfort-Lazarus syndrome, characterized by cleft palate, choanal atresia, spiky hair, and athyreosis.8 In contrast, the vast majority of children with thyroid dysgenesis have hypothyroidism as their only congenital defect and 98% of these cases are sporadic.9
Ten to 15% of congenital hypothyroidism cases are due to dyshormonogenesis, or inborn defects in thyroid hormone synthesis, that are often inherited in an autosomal recessive fashion. Mutations in several of the genes important in thyroid hormone synthesis (including those encoding thyroperoxidase, thyroglobulin, thyroid dual oxidase 2 and 2A, iodotyrosine deiodinase, the sodium-iodine symporter, and the TSH receptor) have been found to cause congenital hypothyroidism in humans (see Figure 4-1).10 Because such kindreds represent a small minority of patients with congenital hypothyroidism, the relevance of these findings to genetic testing and counseling is limited, but these cases have provided important insight into the mechanisms of thyroid hormone synthesis. Interestingly, mild mutations in several of these genes resulting in less severe enzymatic deficiency may present not as congenital hypothyroidism but rather as acquired hypothyroidism or euthyroid goiter in later childhood or adolescence. Recent research has shown that the presentation of human dyshormonogenesis can vary even within the newborn period, presenting as permanent primary hypothyroidism, transient hypothyroidism (reported in infants with monoallelic mutations in DUOX2, which encodes thyroid dual oxidase 2),11 or as delayed TSH rise (due to mutations in DEHAL1, which encodes iodotyrosine deiodinase).12
Radiographic studies such as neck sonography and radionuclide scintigraphy may be performed to characterize the subtype of a child’s congenital hypothyroidism, but, because these findings rarely alter acute management, they should be considered optional. When testing to diagnose etiology is desired, most clinicians obtain a thyroid ultrasound and measure serum thyroglobulin. Visualization of a eutopic thyroid gland indicates dyshormonogenesis or hypoplasia. The absence of a eutopic thyroid gland indicates agenesis if serum thyroglobulin is undetectable or ectopy if serum thyroglobulin is present/elevated. For the latter, if ultrasonography shows no glandular tissue superior to the thyroid bed, 123I scintigraphy may be considered to identify the location of ectopic thyroid tissue. These tests must be interpreted in the context of the patient’s prenatal history, as exposure to maternal antithyroid drugs or the transplacental passage of maternal TSH receptor-blocking autoantibodies can compromise the sensitivity and specificity of imaging. For instance, infants with transient hypothyroidism from maternal TSH receptor-blocking antibodies can have reductions in RAIU and serum thyroglobulin that are diagnostically indistinguishable from thyroid hypoplasia or agenesis.
A small percentage of congenital hypothyroidism is central in origin due to pituitary or hypothalamic defects. Congenital central hypothyroidism is usually accompanied by deficiencies in other pituitary hormones,13 so a full evaluation of pituitary function must be performed whenever secondary hypothyroidism is diagnosed. Germline mutations in genes that regulate pituitary gland formation, including POU1F1, PROP1, HESX1, LHX3, and LHX4 have been documented as causes of familial hypopituitarism in humans.
Transient hypothyroidism can occur in the newborn period secondary to the transplacental passage of maternal medications or thyroid autoantibodies or in infants born to mothers who ingest excessive amounts of iodine (due to transfer through the placental circulation or the breast milk).14 A careful medical and prenatal history will reveal these risk factors.
Clinical signs and symptoms of congenital hypothyroidism can be difficult to detect in the newborn period and, as the majority of cases are sporadic, it not possible to predict which infants will be affected. Accordingly, newborn screening for congenital hypothyroidism is universally recommended and available in most developed countries. Affected neonates who are diagnosed by screening and begin treatment within the first weeks of life can have normal intelligence. Though this is a great triumph of newborn screening, it is important to remember that up to 75% of the world’s population is born in countries without newborn screening. Thus, from a global health perspective, congenital hypothyroidism remains one of the most common preventable causes of mental retardation.
In the United States, screening is performed on dried heel-stick blood and the testing strategy varies between regions.15 There are three common screening strategies: (1) initial T4 test with “reflex” TSH measurement in the subset of samples with the lowest T4 levels; (2) initial TSH test; and (3) combined initial T4 and TSH test.16 Strategies that measure TSH have the potential advantage of detecting isolated hyperthyrotropinemia or mild/subclinical hypothyroidism. In contrast, strategies that measure T4 have the ability to diagnose central hypothyroidism. Screening samples should be obtained between 2 and 5 days of age, and some programs also routinely obtain a second specimen between 2 and 6 weeks of age to capture infants with delayed TSH rise. This phenomenon of delayed TSH rise occurs in about 1:30,000 term newborns but is much more common in low-birth-weight infants (1:95).17 Delayed TSH rise is commonly attributed to mild forms of primary hypothyroidism or to the presence of confounding factors such as prematurity or critical illness that blunt the normal hyperthyrotropinemic response to hypothyroidism.15,18
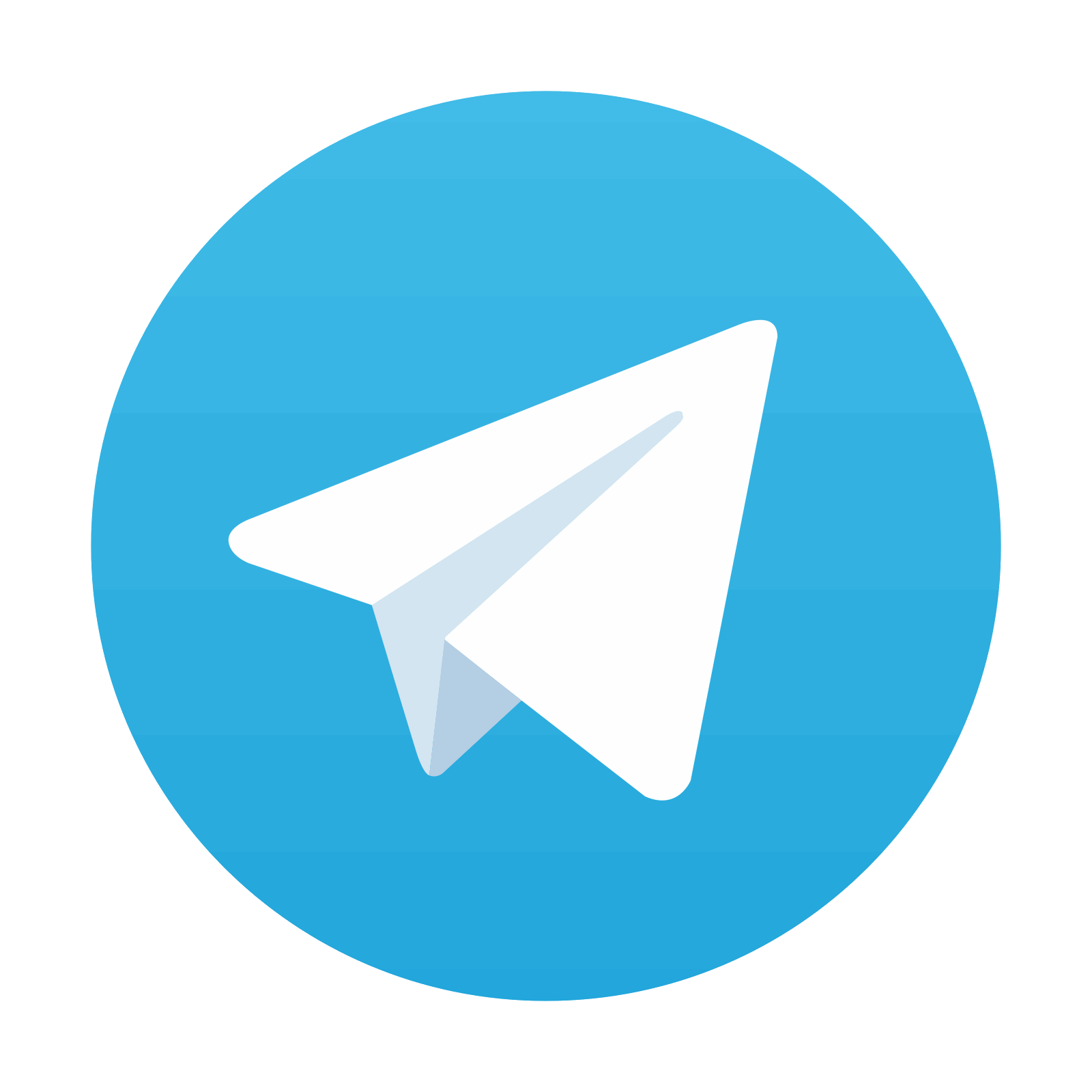
Stay updated, free articles. Join our Telegram channel

Full access? Get Clinical Tree
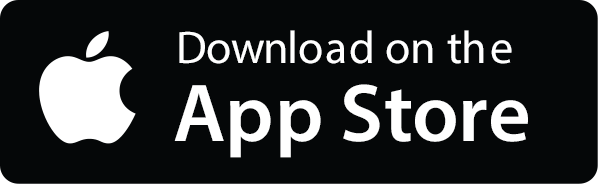
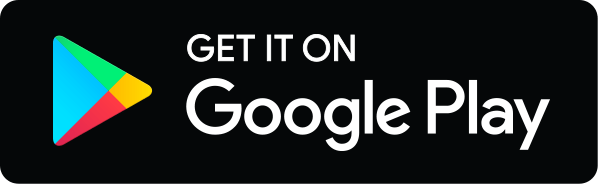