There are broad principles and concepts in endocrinology that the reader must understand. Familiarity with concepts such as negative feedback loops, hormone-receptor function, and hormone replacement therapy allows the physician to generalize what is learned in one area and apply it to others. This gives the ability to predict the effects of an endocrine abnormality or perturbation on downstream hormones and its subsequent clinical effects. Conversely, it also allows the clinician to consider a set of symptoms, work backward to develop a differential diagnosis, and test this by looking for laboratory abnormalities that are diagnostic for an endocrine disease. This chapter reviews many of these basic principles that are applicable across the field in order to provide groundwork for later chapters. Following a discussion of general hormone function and integration of endocrine systems, we will discuss the classification of hormones. Reviews of hormone synthesis, processing, and transport follow, and then we will outline the regulation of hormone secretion. Following this, we will discuss genetics for the endocrinologist, examine the evolving field of hormone receptors, and consider nontraditional endocrine systems. The clinical relevance of the preceding material is apparent in the section covering principles of endocrine disease. Finally, we will summarize important principles in endocrine testing.
The endocrine system consists of a dizzying number of hormones, and newly discovered ones are added to the list on a regular basis. The word “hormone” comes from the Greek word “ormaein” meaning to set in motion or to spur on. This is an apt derivation, because, as classically defined, hormones are chemicals secreted by one tissue that produce effects in distant tissues, leading to an array of physiologic responses. In addition to the classic sense, hormones may have local effects as discussed later in this chapter. In children, we can categorize hormones by the systems that they affect, including growth, reproduction, homeostasis, and energy regulation. Many hormones play roles in multiple categories, emphasizing the complex network of interactions and the redundancy built into these processes. Table 1–1 shows how selected endocrine systems fit into this categorization, illustrating this redundancy.
Hormone Function | Regulatory Hormones | Effector Hormones |
---|---|---|
Growth | GHRH | GH |
Somatostatin | IGF-1 | |
TRH | T4 | |
TSH | T3 | |
LH | Estradiol | |
FSH | Testosterone | |
Insulin | ||
Reproduction | GnRH | Estradiol |
LH | Testosterone | |
FSH | MIS | |
Prolactin | ||
Homeostasis | TRH | T4 |
TSH | T3 | |
CRH | ||
ACTH | Cortisol | |
GnRH | ||
LH | Estradiol | |
FSH | Testosterone | |
ADH | ||
Renin | ||
Angiotensin | ||
Aldosterone | ||
PTH | ||
Vitamin D | ||
Insulin | ||
Epinephrine | ||
Leptin | ||
Energy balance | TRH | T4 |
TSH | T3 | |
GHRH | GH | |
Somatostatin | IGF-1 | |
CRH | Cortisol | |
ACTH | ||
Leptin | ||
Glucagon | ||
Insulin |
Growth is an exceedingly complex process that is unique to pediatric medicine. When considering the endocrine control of growth, many think first of growth hormone (GH), but growth involves many other hormones as well. For example, thyroid hormone and sex steroids are permissive for GH secretion and, in the presence of hypothyroidism or hypogonadism, GH is not secreted normally. GH itself is critical for the secretion of insulin-like growth factor-1 (IGF-1), a hormone that is responsible for many of the effects of GH on linear growth and other metabolic processes, both in fetal life and in childhood and beyond. Before birth, the effects of GH are less noticeable, and individuals with deletions of the GH1 gene encoding GH are of nearly normal size at birth. Conversely, those with deletions of the gene for IGF-1 demonstrate intrauterine growth restriction. In contrast, insulin serves as the main hormone in promoting fetal growth. Insulin-like growth factor-2 (IGF-2), which is not under the regulation of GH, is also an important endocrine mediator of fetal growth. All these hormones have their own set of regulatory hormones and factors, such as growth-hormone–releasing hormone (GHRH) and somatostatin for GH and thyrotropin-releasing hormone (TRH) and thyroid-stimulating hormone (TSH) for thyroxine (T4) and triiodothyronine (T3).
Deficiencies of the regulatory hormones have much the same effects on growth as deficiencies of the primary or effector hormones. Some hormones also play less direct roles, primarily promoting processes important for homeostasis, but without which growth cannot proceed. Examples of these include the hormones required for normal calcium and bone mineralization such as parathyroid hormone (PTH), 25-hydroxyvitamin D, and 1,25-dihydroxyvitamin D, and fibroblast growth factor-23 (FGF-23). Hormones, particularly estrogens, also actively mediate the physiologic cessation of growth during adolescence, as indicated by the failure of growth plate fusion in an individual with an estrogen receptor mutation and in those carrying mutations in the gene for the aromatase enzyme, critical for the synthesis of estrogen.1,2
Reproduction is an essential function of every species, and the endocrine system plays critical roles in both the reproductive process itself and in the anatomical and physiologic development essential for reproduction to occur in both males and females. Developmental defects of the internal and external genitalia and errors of puberty are also unique to pediatric endocrinology and comprise a significant percentage of referrals of patients seen in clinical practice. In the area of reproduction, as in other endocrine systems, there is a large degree of cross-talk between different hormones and their receptors. Beginning with sexual differentiation, a coordinated network of genetic events results in the synthesis and secretion of testosterone and anti-müllerian hormone (AMH, also called müllerian-inhibiting substance [MIS]) that, interacting with their specific receptors, initiate the differentiation of nonspecific or bipotential reproductive structures into either typical male or female features. Participating in this process are regulatory hormones such as human chorionic gonadotropin (hCG), luteinizing hormone (LH), follicle-stimulating hormone (FSH), and inhibin A and B. This activity of the hypothalamic-pituitary-gonadal axis continues into the first few months of life, a time known as the “minipuberty of infancy.” The reproductive system is typically quiescent during the mid-childhood years, but at the time of puberty, gonadal activity restarts in both males and females under regulatory mechanisms involving the central nervous system (CNS) as well as the gonadotropins, LH, and FSH. Whereas AMH appears to be less critical for normal gonadal function in postnatal life, other gonadal factors such as the inhibins remain important. In the male, testosterone secretion during puberty causes various secondary sex characteristics such as muscularity, body hair, and voice deepening, and estrogen secretion in females causes breast development and the establishment of a female body habitus. Thus in the case of the reproductive system, across the life of a developing child, a given hormone may have very different effects, depending on the physiologic and developmental state of the individual, be it fetal or adolescent.
Although regulatory mechanisms are generally fixed, the reproductive system features one of the few variable feedback loops. Whereas gonadotropin secretion in sexually mature females causes ovarian estrogen secretion that leads to suppression of gonadotropin production by negative feedback, at the midpoint of the menstrual cycle this changes to a positive feedback system and results in the LH surge that causes ovulation.
Homeostasis refers to the maintenance of the metabolic milieu of the individual at steady state, and this is arguably the most important function of endocrine systems in general. Homeostasis is not a single process; it consists of many very different aspects. Hormones tightly regulate diverse physiologic features such as maintenance of normal serum osmolality, normal bone mineral content, normoglycemia, metabolic rate, and many others.
Osmoreceptors in the vasculature in part control the release of vasopressin (antidiuretic hormone [ADH]) from the posterior pituitary gland, which subsequently controls free water excretion and regulates water balance and serum osmolality. The renin-angiotensin-aldosterone system provides separate but overlapping regulation of sodium and potassium balance.
PTH and 1,25-dihydroxyvitamin D, the activated form of vitamin D, maintain bone mineral content, serum calcium, and serum phosphorus concentrations at a steady state. Other endocrine factors directly influencing this system include FGF-23, PTH-related protein (PTHrP), and calcitonin.
Glucose availability is critical for energy homeostasis, and a far-reaching system of apparently unconnected hormones (see the “Glucose Control” section later in this chapter) controls plasma glucose levels. Basal metabolic rate, defined as the rate of oxygen consumption at rest, is partially under the influence of thyroid hormones, including the regulatory hormones, TRH and TSH, and the effector hormones, T4 and its activated form, T3. It is T3 that binds to the intracellular thyroid hormone receptor to alter the transcription of genes controlling metabolic rate.
Endocrine regulation is essential for handling of the body’s energy needs, including the immediate use and storage of nutrients such as glucose, proteins, and lipids. Insulin permits the uptake of glucose into muscle cells where it is converted to energy in the form of adenosine triphosphate (ATP). At the same time, insulin suppresses the oxidation of fatty acids and promotes energy storage as glycogen and triglycerides. Deficiency of insulin, as occurs in type 1 diabetes, leads to hyperglycemia, with lack of normal glucose utilization, as well as excessive lipolysis, fatty acid oxidation, and protein breakdown. These pathophysiologic processes result in the polyuria, polydipsia, weight loss, and ketoacidosis seen in untreated diabetes. Other hormones also influence energy storage and delivery, including cortisol and GH. A complex neuroendocrine system controls food intake with remarkably fine regulation of body weight. This regulatory system allows for stable weight over a period of years, using leptin as the primary adipocyte-derived endocrine hormone leading to satiety and ghrelin as a peripheral signal of hunger.3
As seen from the previous discussion, endocrine control of physiologic processes often features a rich interweaving of hormone systems, with a great deal of redundancy. Table 1–1 and Figure 1-1 illustrate this overlap, with several of the hormone systems, such as the GH-IGF-1 and thyroid axes, being involved in more than one aspect of endocrine function. Such integration of multiple hormone systems in the regulation of a body process increases the robustness and stability of the system and allows for smoother and finer regulation. Two examples of this hormone integration are growth and the regulation of plasma glucose levels.
Human growth involves an array of other endocrine axes in addition to the classic GH-IGF-1 axis consisting of the hypothalamic regulatory hormones; GHRH and somatostatin; GH itself; IGF-1; and various binding hormones, carrier proteins, and receptors4 (see Figure 1-1). Thyroid hormone is essential for normal GH secretion and efficacy. During adolescence, the hypothalamic-pituitary-gonadal axis is also essential for normal growth. Delivery of adequate amounts of glucose, amino acids, and lipids to cells is clearly necessary for normal metabolism, and insulin, cortisol, and leptin are required for this to occur. Bone metabolism also needs to flourish for proper skeletal development, and this requires multiple steps of endocrine input, including PTH, FGF-23, and vitamin D metabolites, as well as input from GH and IGF-1. All of this must occur on a background of normal electrolyte balance and normal serum osmolality.
Plasma glucose concentration is finely regulated within a relatively narrow range, and this also occurs through a complex interaction of numerous endocrine processes. Insulin provides the primary regulation within the normal physiologic range. If a large influx of glucose occurs, putting upward pressure on the glucose concentration, insulin secretion also increases, promoting entry of glucose into muscle cells, glycogen synthesis, and lipogenesis. Insulin secretion is modulated by incretin hormones such as glucagon-like peptide-1 (GLP-1). Plasma glucose levels declining into the hypoglycemic range, however, suppress insulin secretion, leading to lipolysis and fatty acid oxidation which provide additional substrate for energy. Hypoglycemia also activates counter-regulatory hormone secretion. These counter-regulatory hormones consist of those with rapid actions, such as epinephrine and glucagon, and those with delayed actions, such as GH and cortisol. These counter-regulatory hormones cause increased hepatic glycogen breakdown and gluconeogenesis. Proteolysis releases amino acids for use in gluconeogenesis, again providing for system redundancy.
The signal transduction pathways of the endocrine system are very elaborate.5 However, the processes are actually based on a simple concept. Hormone binding to its receptor causes a conformational change of the receptor, which transmits a signal that initiates the physiologic response. The specificity of the receptor for its hormone, the specific cell type, and the specific signaling system in the cell all contribute to the specific response that is seen. There are three major classifications of hormones based on chemical structure: proteins or peptides, steroids, and amines (Table 1–2). The structure of the hormone determines both the location of the hormone receptor (membrane or nuclear) and the half-life of the hormone in circulation. Because peptide hormones are water soluble, peptide hormones bind to membrane receptors. Most amine hormones also bind to membrane receptors, except for thyroid hormone, which binds to nuclear receptors. Steroid hormones also bind to nuclear receptors. Thyroid hormones have the longest half-life, which is measured in days, but other amine hormones have the shortest half-life of only a few minutes. Peptide and steroid hormones fall in the middle, with half-lives ranging from a few minutes to several hours. Hormones that circulate in low concentrations rapidly associate and dissociate from their receptors, such that receptor occupancy is a function of both hormone concentration and receptor affinity.
Peptide Hormones | Steroid Hormones | Amine Hormones |
---|---|---|
GHRH | Cortisol | Epinephrine |
Somatostatin | Estradiol | Norepinephrine |
GH | Testosterone | Dopamine |
IGF-1 | 25-hydroxyvitamin D | T4 |
TRH | 1,25-dihydroxyvitamin D | T3 |
TSH | ||
GnRH | ||
LH | ||
FSH | ||
hCG | ||
MIS | ||
Prolactin | ||
Insulin | ||
ACTH | ||
CRH | ||
MSH | ||
PTH | ||
PTHrP | ||
ADH |
The majority of hormones are peptide hormones.5 Peptide hormones vary greatly in size from as small as three peptides to large multi-subunit glycoproteins. Examples of peptide hormones are listed in Tables 1-2 and 1-3. Because peptide hormones are not fat soluble, they do not easily cross the cell membrane and thus bind to membrane receptors. When protein hormones bind to their receptors, there is an immediate induction of the intracellular signaling pathway associated with that particular receptor. Depending on the specific receptor, the signaling cascades may activate or inhibit cyclic adenosine monophosphate (cAMP) production, activate calcium or potassium channels, or regulate other proteins by phosphorylation. The signaling pathways for many membrane receptors are depicted in Figure 1-2. The membrane receptor families and their associated signaling pathways are shown in Table 1–3.
Figure 1-2
Membrane receptor signaling. MAPK, mitogen-activated protein kinase; PKA, -C, protein kinase A, C; TGF, transforming growth factor. For other abbreviations, see text. (From Jameson JL. Principles of endocrinology. In: Jameson JL, ed. Harrison’s Endocrinology. New York, NY: McGraw-Hill; 2006:1-15.)
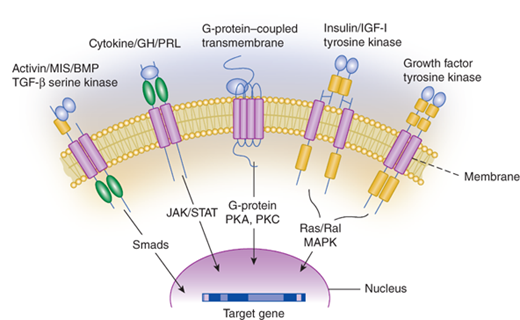
Receptors | Effectors | Signaling Pathways |
---|---|---|
G-protein–coupled-seven transmembrane (GPCR) | ||
β-Adrenergic | Gsα, adenylate cyclase | Stimulation of cAMP production, protein kinase A |
LH, FSH, TSH | Ca2+ channels | |
Glucagon | ||
PTH, PTHrP | Calmodulin, Ca2+-dependent kinases | |
ACTH, MSH | ||
GHRH, CRH | ||
α-Adrenergic | Giα | Inhibition of cAMP production |
Somatostatin | Activation of K+, Ca2+ channels | |
TRH, GnRH | Gq, G11 | Phospholipase C, diacylglycerol-IP3, protein kinase C, voltage-dependent Ca2+ channels |
Receptor tyrosine kinase | ||
Insulin, JGF-1 | Tyrosine kinases, IRS-1 to IRS-4 | MAP kinases, Pl 3-kinase, RSK |
EGF, NGF | Tyrosine kinases, ras | Raf, MAP kinases, RSK |
Cytokine receptor–linked kinase | ||
GH, PRL | JAK, tyrosine kinases | STAT, MAP kinase, Pl 3-kinase, IRS-1, IRS-2 |
Serine kinase | ||
Activin, TGF-β, MIS | Serine kinase | Smads |
The glycoproteins are structurally the most complex group of peptide hormones.6 The carbohydrate moiety constitutes 15% to 35% of the hormone by weight. These hormones include LH, FSH, TSH, and hCG. Glycoprotein hormones are composed of two subunits joined by noncovalent forces. They share a common α-subunit and a hormone-specific β-subunit. Although the β-subunits are unique, they are homologous, with the β-subunits of hCG and LH sharing the most homology. In order for hormones to affect the target organ, they must bind to and activate their specific receptors. In the physiologic state, hormones bind only to their specific receptor. However, in the case of hormone excess, a hormone may also bind to closely related receptors. This is demonstrated by the use of synthetic hCG by endocrinologists to stimulate the LH receptor during testing of testicular function in prepubertal boys. This also may be demonstrated in cases of severe hypothyroidism. At very high levels, TSH may bind to the FSH receptor, activating the gonads and causing testicular enlargement in boys and ovarian stimulation in girls (van Wyk-Grumbach syndrome).7 The receptors for the glycoprotein hormones are G-protein–coupled receptors (GPCRs) that activate the adenylyl cyclase and inositol triphosphate-diacylglycerol pathways.5,8
Steroid hormones are derived from cholesterol (Figure 1-3).5 Steroid hormones include estrogen, testosterone, cortisol, vitamin D, and retinoids. Because of their lipophilic nature, steroid hormones readily cross the cell membrane and bind to nuclear receptors. Once hormone is bound to its nuclear receptor, the receptor binds to the DNA response element, usually as a dimer. Steroid hormone binding to its receptor results in activation or repression of gene transcription (Figure 1-4). Thus, the effects of steroid hormones are delayed compared to peptide hormones. However, because gene transcription is altered, the effect of steroid hormones is sustained.
Figure 1-3
Hormones of the adrenal cortex. The principal hormones synthesized and released by the adrenal cortex are glucocorticoids (cortisol), mineralocorticoids (aldosterone), and androgens (dehydroepiandrosterone). These steroid hormones are derived from cholesterol. (From Molina PE. Adrenal gland. In: Molina PE. Endocrine Physiology. 2nd ed. New York, NY: McGraw-Hill; 2006:123-156.)
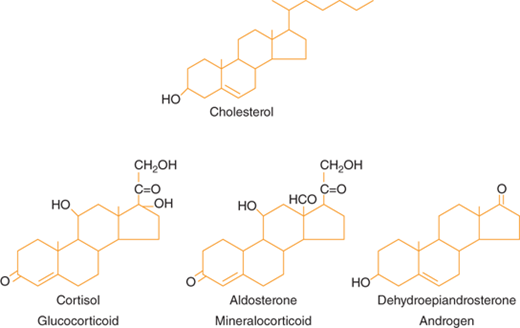
Figure 1-4
Nuclear receptor signaling. AR, androgen receptor; DAX, dosage sensitive sex-reversal, adrenal hypoplasia congenita, X-chromosome (now called NR0B1, nuclear receptor subfamily 0, group B, member 1); ER, estrogen receptor; GR, glucocorticoid receptor; HNF4α, hepatic nuclear factor 4α; PPAR, peroxisome proliferator activated receptor; PR, progesterone receptor; RAR, retinoic acid receptor; SF-1, steroidogenic factor-1; TR, thyroid hormone receptor; VDR, vitamin D receptor. (From Jameson JL. Principles of endocrinology. In: Jameson JL, ed. Harrison’s Endocrinology. New York, NY: McGraw-Hill; 2006:1-15.)
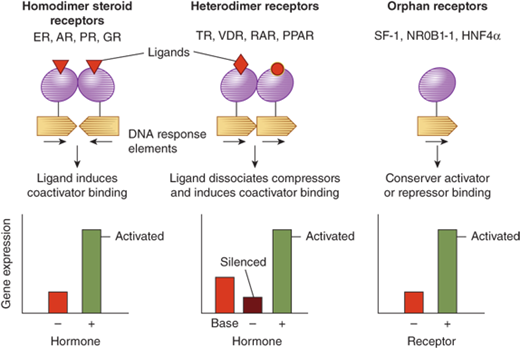
Amine hormones are derived from the amino acid, tyrosine.8 This class includes dopamine, epinephrine, norepinephrine, and thyroid hormones. Like the peptide hormones, the amine hormones usually bind to membrane receptors. Thyroid hormones, however, bind to nuclear receptors. Similar to steroid hormones, it takes longer to see the effects of increases in thyroid hormones, but the effects are sustained. Epinephrine, norepinephrine, and dopamine induce rapid- and short-lived changes, even more rapidly than those seen with the peptide hormones.
There are numerous steps involved in the production of the active hormone. Some hormones are directly synthesized in the active form and others require further processing to the active hormone.
No matter which class a hormone belongs to, the rate-limiting step in hormone activation is usually the synthesis of the hormone.
The synthesis of peptide hormones is dependent on classic gene expression.5 The gene for a peptide hormone is transcribed into messenger RNA (mRNA) which is then translated into a protein. The protein is further modified by posttranslational processing. Synthesis of peptide hormones is regulated by DNA regulatory elements found on many genes. However, the peptide hormone genes also contain specific hormone response elements, resulting in very precise control of synthesis. This is exemplified by direct repression of TSH synthesis by binding of thyroid hormones to a thyroid hormone response element on the TSH gene. Peptide hormones are synthesized in the endoplasmic reticulum and then transferred to the Golgi apparatus, where they are packaged into secretory granules (Figure 1-5). Mature secretory granules sit immediately beneath the plasma membrane. Typically, the signal for granule release causes a change in intracellular calcium, resulting in fusion of the granule to the plasma membrane and, finally, release of its contents.
Figure 1-5
Peptide hormone synthesis. Peptide hormones are synthesized as preprohormones in the ribosomes and processed to prohormones in the granular endoplasmic reticulum (ER). In the Golgi apparatus, the hormone or prohormone is packaged in secretory vesicles, which are released from the cell in response to an influx of Ca2+. The rise in cytoplasmic Ca2+ is required for docking of the secretory vesicles in the plasma membrane and for exocytosis of the vesicular contents. The hormone and the products of the posttranslational processing that occurs inside the secretory vesicles are released into the extracellular space. Examples of peptide hormones are ACTH, insulin, growth hormone, and glucagon. (From Molina PE. General principles of endocrine physiology. In: Molina PE. Endocrine Physiology. 2nd ed. New York, NY: McGraw-Hill; 2006:1-24.)
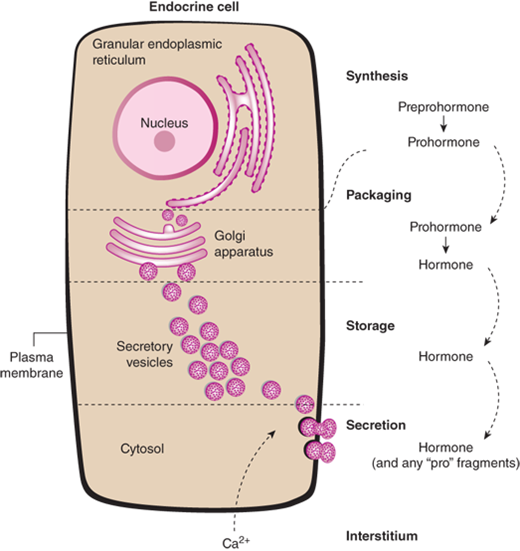
Steroid hormones are derived from cholesterol.9 The first and rate-limiting step in hormone synthesis is the cleavage of cholesterol to pregnenolone in the inner mitochondrial membrane by the enzyme P450scc (cholesterol side-chain cleavage). The hormone precursors are shuttled back and forth between the endoplasmic reticulum and the mitochondria until the final product is produced. The basic steroid backbone is depicted in Figure 1-3. There is very little storage of steroid hormones, with the finished product usually diffusing into circulation after synthesis.
The amine hormones are derived from tyrosine.8 Thyroid hormone synthesis is critically dependent on iodine ingestion.10 Thyroglobulin is a large glycoprotein that contains multiple tyrosine residues. It is synthesized in thyroid follicular epithelial cells. Thyroglobulin is secreted into the follicular lumen where it undergoes posttranslational modification. TSH binding to its receptor on the basolateral membrane of thyroid follicular epithelial cells results in stimulation of the enzymatic steps involved in thyroid hormone synthesis, thyroid hormone release, and growth of the thyroid gland.10 In hypothyroidism, the high levels of TSH stimulate thyroid growth, resulting in a goiter. In hyperthyroidism, stimulation of the TSH receptor by the thyroid antibodies results in thyroid growth and development of a goiter. The thyroid hormones, T4 and T3, are both secreted from the thyroid gland. However, T4 is produced in far excess compared to T3.
Catecholamines are synthesized from tyrosine in the adrenal medulla.9 Tyrosine is actively transported into the cell, where the first and rate-limiting step is catalyzed by tyrosine hydroxylase. A total of four enzymatic reactions produce the final product, epinephrine. Dopamine and norepinephrine are precursors to the final epinephrine product.
Many hormones are first synthesized as preprohormones or prohormones and then posttranslationally modified to the active hormone. Typically, hormones that are to be secreted contain an N-terminal signal sequence that directs the prohormone to the endoplasmic reticulum, where this signal sequence is then cleaved.
In the follicular lumen of the thyroid cells, thyroglobulin undergoes iodination of multiple tyrosine residues followed by coupling of the iodotyrosines in a reaction catalyzed by thyroid peroxidase.11 After coupling, thyroglobulin is processed in lysosomes to produce T4 and T3.
PTH is also synthesized as a preprohormone.8,12 The initial translational product of the PTH gene is preproPTH, which contains hydrophobic amino acids on the N-terminus that are critical for efficient transport of the hormone into the endoplasmic reticulum for further processing. In the endoplasmic reticulum, the preproPTH is quickly cleaved to proPTH that contains six amino acids at the N-terminal end in addition to the bioactive 84-amino acid PTH.
Other peptide hormones are made up of subunits that must be associated before the hormone is active. The initial insulin translational product, preproinsulin, consists of an α- and β-subunit connected by C-peptide.13 During insertion into the endoplasmic reticulum, the signal peptide is cleaved, producing proinsulin. Prohormone convertases cleave the C-peptide, generating the active insulin hormone. C-peptide and insulin are packaged together in secretory granules in the Golgi apparatus and released together. Thus, in certain instances, both C-peptide and insulin are measured to distinguish endogenous causes of hypoglycemia from exogenously administered insulin.
LH, FSH, hCG, and TSH are composed of a common α-subunit and unique β-subunits, encoded by distinct genes.5
Some genes encode a prohormone that can be cleaved to multiple hormones.5 The prohormone, proopiomelanocortin (POMC), is posttranslationally cleaved to several different hormones. POMC is cleaved to ACTH; β-endorphin; and α-, β-, and γ-melanocyte–stimulating hormone (MSH). This is thought to be why patients with Addison disease who have supraphysiological levels of ACTH (and POMC) are hyperpigmented.
Glycoproteins are the largest hormones and consist of a common α-subunit and a unique β-subunit.5 The carbohydrate moiety that is cotranslationally attached to the glycoprotein hormone protects the nascent polypeptide from intracellular degradation. Carbohydrate attachment is also essential for correct folding and combination of the subunits. The carbohydrate attachment is also required for secretion of the mature hormone from the cell. After secretion of the hormone, the carbohydrate moiety is important in stabilizing the hormone in circulation and appears to be important for receptor signaling, but it does not affect affinity for the hormone receptor. The glycoprotein hormones bind the GPCRs.
Some hormones are modified after secretion into the circulation. Although testosterone is the major androgen in the circulation of men, dihydrotestosterone (DHT) is the more potent androgen.14 In the peripheral tissues (primarily the skin and prostate), DHT is formed by the actions of two isoenzymes, 5α-reductase types 1 and 2. In males, the majority of circulating estradiol is produced by aromatization of testosterone by the aromatase enzyme, primarily found in adipocytes as well as Leydig cells and placenta. The increase in adipocyte-derived aromatase is largely responsible for gynecomastia seen in overweight men.
Vitamin D is either absorbed from the gut (vitamin D2) or synthesized in the skin from 7-dehydrocholesterol and the actions of sunlight (vitamin D3).15 These two forms are converted to 25-hydroxyvitamin D in the liver, which is the major circulating form of vitamin D. In the kidney, the 25-hydroxyvitamin D form is hydroxylated to the active form, 1,25-dihydroxyvitamin D.
Although both T4 and T3 are secreted from the thyroid, the majority of T3 comes from postsecretion conversion of circulating T4 to T3.10 This occurs primarily in the liver by deiodination of T4 to form T3.
The half-life of a hormone in circulation is dependent on its synthesis rate, amount of binding to proteins, and rate of degradation.5 Some hormones, such as somatostatin, vanish quickly whereas other hormones, such as TSH, have a prolonged half-life. Because somatostatin has effects on almost every organ, its short half-life allows for more local control of its activity. Modification of the somatostatin structure to lengthen its half-life has allowed development of analogs of somatostatin for treatment of diseases such as acromegaly and hyperinsulinism.
Most peptide hormones have a relatively short half-life of 20 minutes or so. Hormones such as GH, ACTH, LH, PTH, and prolactin have sharp peaks of secretion and decay. Therefore, to accurately measure these hormones, they must be either measured frequently (every 10-20 minutes) over a long duration (8-24 hours) or in response to a stimulatory event. The short half-life of PTH has proven to be advantageous in being able to detect intraoperatively whether or not a parathyroid adenoma has been removed. The long half-life of T4 (7 days) allows replacement therapy to be dosed once daily. However, this also requires 4 weeks for steady-state levels to be reached. T3, on the other hand, has a half-life of only 1 day, requiring dosing two to three times per day.
Hormones may be degraded through enzymatic modifications in the liver.8 These modifications include hydroxylation, oxidation, glucuronidation, sulfation, and reduction with glutathione. They may also be degraded at their target site by lysosomal degradation of the hormone with recycling of the receptor. The inactivated hormone is then excreted through the feces or the urine.
Many hormones circulate bound to proteins, protecting hormones from rapid degradation.