In crabs and lobsters, the sinus gland (a neurohemal organ that contains the nerve endings of the X-organs) acts as a storage and release site for neuropeptides, which are synthesized in peripheral neurons in the eyestalk [20]. The X-organ/sinus gland complex regulates important physiological functions, including molting, reproduction, metamorphosis, metabolism, osmoregulation, and pigmentation [21]. Some of the released neuropeptides are hormones, such as molt-inhibiting hormone, vitellogenesis-inhibiting hormone, crustacean hyperglycemic hormone, and mandibular organ-inhibiting hormone [22]. The androgenic gland controls sexual differentiation via production of the androgenic gland hormone [23], whereas the mandibular organ, via production and release of the juvenile hormone III analog methyl farnesoate, seems to be central for development of gonads [reviewed in 24,25]. This hormone also seems to be involved in regulating molting [26,27] and other important physiological processes in crustaceans [reviewed in 28]. The biosynthesis and secretion of methyl farnesoate is complex and seems to be at least partly under control of the mandibular organ-inhibiting hormone [29]. The Y-organ in turn synthesizes the ecdysteroids [reviewed in 16], a group of polyhydroxylated ketosteroids (392 isoforms identified so far) [30] essential for molting [15]. Being a precursor of ecdysteroids, cholesterol is the dominant sterol in crustaceans [31]. However, crustaceans are not able to synthesize cholesterol de novo [31], which means that the animals are in need of dietary intake of cholesterol. The Y-organ releases ecdysone into the hemolymph where it is converted into the active hormone 20-hydroxyecdysone (20-HE) by hydroxylation [32]. Additional suggestions for ecdysteroid production centers (in arthropods) are the ovary and the epidermis [reviewed in 16,33]. It seems that the ecdysteroids circulate freely and enter cells by diffusion [reviewed in 24], but there is some evidence of an energy-dependent entry of hormones in larger crustacean species [34, reviewed in 24].
5.2.2.1 Molting and Growth
Molting (ecdysis) is regulated by the Y-organ and X-organ/sinus gland complex in this way: In the absence of appropriate stimuli, the X-organ produces molt-inhibiting hormone, which is released by the sinus gland. The target of the molt-inhibiting hormone is the Y-organ. At high titers of molt-inhibiting hormone, the Y-organ is inactive. Under internal or external stimuli, molt-inhibiting hormone release is inhibited and the Y-organ releases ecdysone as a precursor to ecdysis [35]. The circulating titers of ecdysteroids vary along the molt cycle in larger crustaceans [reviewed in 36]. Immediately after ecdysis, the titer is low and generally remains so during intermolt. A major increase occurs at beginning of premolt, followed by a steep drop just before the actual molt. At least two other ecdysteroids are also released by the Y-organ: 3-dehydroxyecdysone and 25-deoxyecdysone, where the latter is a precursor to the active ponasterone A (PoA; 25-deoxy-20-hydroxyecdysone) [37,38, reviewed in 16]. PoA may also be involved in crustacean molt, since it has been found in high levels in premolt stages [39]. Another important hormone for growth in crustaceans seems to be the crustacean hyperglycemic hormone, the primary function of which is to utilize glucose for various metabolic processes, including timing of ecdysis, possibly by regulating water uptake necessary for successful molting [40,41].
In addition to these hormones, molting also seems to be partly regulated by methyl farnesoate. Tamone and Chang [27] have provided direct evidence for a stimulatory effect of methyl farnesoate on ecdysteroid secretion from crab Y-organ in vitro. They conclude that it is not surprising that growth is regulated not only by inhibitory factors, such as molt-inhibiting hormone, but also by stimulatory factors, such as methyl farnesoate. However, their results seem to contradict those by Mu and LeBlanc [42], who reported that methyl farnesoate has an inhibitory effect on ecdysteroid production in D. magna. Again, this reflects on the complexity and diversity of the crustacean endocrine system and indicates that there are still knowledge gaps in the understanding of pathways of crustacean hormones, in particular in smaller crustacean species.
5.2.2.2 Reproduction
Reproduction in crustaceans is only partly understood, and there seem to be great species differences in how it is regulated [reviewed in 18]. As mentioned, it is generally accepted that in larger crustacean species, the androgenic gland, which synthesizes the androgenic gland hormone, controls sexual differentiation [23,43]. Presence of androgenic gland hormone results in the development of the male reproductive system and secondary sexual characteristics. Absence of androgenic gland hormone results in the differentiation of ovaries whereas hormones inducing female secondary sexual characteristics seem to be of ovarian origin [reviewed in 23,44]. Except for the vitellogenin-inhibiting hormone (also called gonad-inhibiting hormone), which in females inhibits the development of oocysts, there is some evidence for a gonad-stimulating hormone produced by the brain and thoracic ganglia [reviewed in 45]. As mentioned, methyl farnesoate also has an important role in reproduction [26]. Laufer et al. [46] have, for instance, shown that methyl farnesoate stimulates ovarian growth in the red swamp crayfish, Procambarus clarkii. Even though the ecdysteroids mainly control ecdysis, it has been suggested that they may be involved in reproduction [reviewed in 16], for example, by synchronization of embryo hatching [47]. A few vertebrate-type estrogens have been identified in crustaceans [48,49], and earlier research has shown that treatment of crustaceans with vertebrate estrogens induces maturation of female reproductive organs [50–53]. Overall, however, the functional role of estrogens has not been fully established to date [23].
5.3 STATE OF THE ART: WHAT DO WE KNOW ABOUT ENDOCRINE DISRUPTION IN CRUSTACEANS?
5.3.1 General
Although the crustacean endocrine system is not so well known, especially among the smaller crustacean species often used for regulatory risk assessment purposes, quite a few studies have focused on the potential effects of known vertebrate endocrine disrupters and pesticides with a known mode of action in insects. In general, however, these studies have had difficulties in establishing causality between exposure and endocrine-mediated effects. This is likely due to the scarce knowledge about basic endocrinology but also because many of these endocrine disrupters do not appear to have an endocrine disrupting effect in crustaceans. Still, some evidence shows that the endocrine system of crustaceans can be interrupted by known environmental endocrine disrupters, although not necessarily always via expected modes of action. This section presents a number of studies that have focused on endocrine disruption in crustaceans, including end points that are reflective of endocrine disruption. The list is not complete, and the presented studies should be considered as illustrative examples.
5.3.2 Daphnia sp.
With the increasing number of studies focusing on endocrine disruption in fish some 15 years ago, it became evident [32] that endocrine disruption could also be an issue in other important animal groups. In this context, a set of studies focusing on endocrine disruption in the standard test species, Daphnia magna, were published in the open literature from the end of the 1990s on. As early as 1997, Zou and Fingerman [54,55] exposed D. magna to the nonsteroidal vertebrate estrogen diethylstilbestrol and the estrogenic pesticide endosulfan. The study was based on a hypothesis that there could be a structural resemblance between vertebrate and crustacean steroids. The study showed that molting was delayed at relatively high concentrations of both substances. Although these findings were not clear cut from an endocrine disruption point of view, the authors continued to explore the possibility that the observed effects were related to endocrine disruption. However, what in laboratory tests with D. magna was first believed to be an interaction of estrogenic substances with the ecdysteroid system was later found in the fiddler crab, Uca pugilator, to be an inhibition of chitobiase activity, a chitinolytic enzyme, which is important for digestion of the chitinous exoskeleton [56,57].
LeBlanc and McLachlan [58] exposed D. magna to the anti-androgen cyproterone acetate and studied growth, molting, sexual differentiation, and reproduction. Sublethal concentrations of cyproterone acetate reduced growth but not molting. Offspring production was also reduced. The authors speculated that the observed adverse effects could be endocrine mediated, but that further studies were required. Later Olmstead and LeBlanc [59] exposed D. magna to diethylstilbestrol, the steroidal vertebrate androgen androstenedione, and the insect juvenile hormone analog methoprene and studied the development of secondary sexual characteristics in males and females. Although these hormones and hormone mimics induced some alterations in sex characters, the authors could not establish that they were endocrine mediated.
In 2001, Baldwin et al. [60] published a paper in which D. magna were exposed to 20-HE and PoA, both having affinity to the ecdysteroid receptor (see Section 5.2). However, over 21 days of exposure, these active crustacean hormones had marginal effects on reproduction at relevant concentrations, whereas mortality was evident among adults. By studying the daphnids over a second generation, a weak effect on reproduction was observed for PoA but not for 20-HE. The authors speculated that this difference could have been related to either structural differences between the two hormones (with 20-HE being more prone to biotransformation) and/or that a higher affinity of PoA for the ecdysone receptor may have caused an effect on secondary vitellogenesis in developing daphnids, manifested as reduced reproduction because of smaller brood size. The authors could not establish a strong causality for these two active crustacean hormones.
Following this study, LeBlanc and coworkers continued the work on daphnids to provide evidence for potential endocrine disruption in crustaceans. For example, Olmstead and LeBlanc [61] claimed that methyl farnesoate was the likely endocrine factor regulating the development of male D. magna. Later on they also showed that an insect juvenile hormone analog, pyriproxyfen, could stimulate the production of males [62]. In line with these findings, Tatarazako et al. [63] showed that methyl farnesoate and pyriproxyfen, as well as three other juvenile hormone analogs—fenoxycarb, methoprene, and juvenile hormone-III—stimulated male production in D. magna. The five substances showed similar concentration-related responses, although fenoxycarb and pyriproxyfen were clearly more potent.
Mu and LeBlanc [64] demonstrated that ecdysteroids regulate critical processes in daphnid embryo development and that testosterone elicits embryo toxicity in daphnids by interfering with ecdysteroid activity. By using an ecdysone-responsive cell line, it was further shown that testosterone significantly antagonized the action of 20-HE. In a follow-up study, the same authors exposed daphnids to the agricultural fungicide fenarimol [65], which lowered endogenous ecdysone levels and delayed molting in a concentration-dependent fashion. The latter effect was mitigated by co-exposure to 20-HE exposure. Developmental abnormalities were also observed and could be associated with suppressed ecdysone levels in embryos. These abnormalities could also be prevented by co-exposure to 20-HE. Mu and LeBlanc [42] further examined the potential synergistic effects of a mixture of fenarimol and testosterone on the development of D. magna. Based on the findings from the two earlier studies, they hypothesized that fenarimol would lower ecdysone levels, which would increase the testosterone binding to the ecdysone receptor and, in turn, cause “greater than additive toxicity.” Their study confirmed the hypothesis as fenarimol enhanced the toxicity of testosterone, whereas testosterone had no effect on the toxicity of fenarimol.
5.3.3 Barnacles
At about the same time as the above-mentioned studies on daphnids reached the open scientific literature, reports on potential endocrine disruption in other crustacean species were also published. Among the first studies to introduce the concept of endocrine disruption, Billinghurst et al. [66] examined the effects of two estrogens, nonylphenol (an estrogen agonist) and 17β-estradiol, on larval settlement and production of a larval storage protein (cypris major protein, CMP) in Balanus amphitrite. Cyprids use CMP during settlement and early postsettlement development. Since CMP is closely related to vitellin, the hypothesis of the study was that estrogen exposure would cause enhanced cyprid settlement via an elevation in the levels of CMP. However, both estrogen-active substances inhibited settlement, and the authors concluded that there was no evidence for endocrine-mediated effects.
5.3.4 Copepods
A range of experimental studies on endocrine disruption have also been performed using different, predominantly marine copepod species. For instance, Hutchinson et al. [67,68] found that exposure to several steroids had no effect on the survival and development of the harpacticoid copepod Tisbe battagliai. At about the same time, Bechmann [69] showed that high levels (>62 μg/L) of nonylphenol were acutely toxic to T. battagliai but that exposure to a lower level (31 μg/L) did not affect any of the measured life-table variables (survival, sex ratio, fecundity). Breitholtz and Bengtsson [70] did not find evidence of endocrine disruption in the harpacticoid copepod Nitocra spinipes (see Figure 5.2) after exposure to the estrogens 17α-estradiol, 17α-ethynylestradiol, and diethylstilbestrol.
FIGURE 5.2 Female of the Harpacticoid Copepod Nitocra spinipes carrying an egg sac. Source: Illustration by Göte Göransson.
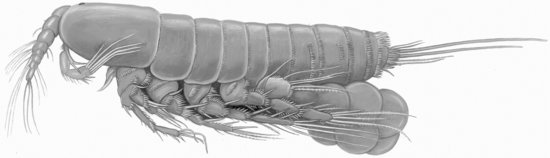
Dahl et al. [71] used the same species exposed to the pharmaceutical simvastatin and studied alterations in development, growth, and RNA content. Statins act as an HmG-CoA reductase (HMGR) inhibitor, an enzyme that regulates cholesterol synthesis in humans but is also a key enzyme in the biosynthesis of isoprenoids such as methyl farnesoate. For example, Li et al. [72] have shown that statins inhibit HMGR and thus the production of methyl farnesoate, which could result in developmental alterations [26]. In the study by Dahl et al. [71], the exposure to simvastatin resulted in significant changes in all end points studied, with a markedly increased development rate as compared to the control at very low concentrations of the pharmaceutical (ng/L level). At higher concentrations, this effect leveled off at the same time as mortality increased. The difference between the acute and subchronic toxicity was more than a factor of 5,000. This study indicates that endocrine disruption does take place in crustaceans. It also shows that endocrine disrupters may have effects at much lower concentrations than expected and that specific effects may be masked at too-high concentrations. N. spinipes was also used in a follow-up study with lindane [73]. This insecticide, apart from being a gamma-aminobutyric acid (GABA) inhibitor, has also been shown to have an ecdysteroid antagonistic effect (i.e., it blocks the ecdysteroid receptors so that native ecdysteroids are not able to integrate properly) [74,75]. A range of developmental variables, growth, RNA content, and ecdysteroid levels were studied. Both development and growth were strongly affected at sublethal concentrations. Whereas all other end points followed a clear concentration-related pattern, the ecdysteroid levels followed a somewhat bell-shaped curve, which has earlier been described for other endocrine disrupters [76]. Although the exact mechanisms behind the observed effects could not be fully established, this study indicates that both neural and endocrine disruption may take place simultaneously.
Chandler and coworkers have also studied potential endocrine disruption in another harpacticoid copepod species, Amphiascus tenuiremis [77,78]. For instance, they found that environmentally relevant concentrations of the GABA-inhibitor insecticide fipronil and its degradation products delayed the maturation of A. tenuiremis copepodites to adults and strongly reduced the production of nauplii. These effects were also integrated into a matrix model, which predicted declining population sizes even at the lowest tested concentration (i.e., 0.16 μg/L). Since fipronil is a GABA inhibitor, it is likely that the effects were mediated via disruption of the neural system. However, a follow-up study showed that only males were affected at low levels of fipronil, as indicated by lowered ecdysteroid levels, elevated male vitellogenin, and malfunction in male reproductive ability [78]. Like the study by Dahl et al. [73], this study indicates that both neural and endocrine disruption may take place simultaneously.
The calanoid copepod Acartia tonsa has been used for a number of studies related to endocrine disruption in crustaceans. For instance, Anderson et al. [79] studied alterations in early development and metamorphosis in A. tonsa exposed to 14 substances with known endocrine activity in crustaceans, other arthropods, or vertebrates: natural vertebrate hormones (17β-estradiol, estrone, testosterone, progesterone), natural invertebrate hormones (20-HE, juvenile hormone-III), hormone antagonists (flutamide, tamoxifen, hydroxyflutamide), xeno-estrogens (17α-ethynylestradiol, 4-octylphenol, bisphenol-A), and environmentally relevant compounds (nonylphenol ethoxylate, diethyl phthalate). Although for some substances effects on early development and metamorphosis were observed at concentrations far below those causing acute lethal effects, no clear evidence for endocrine disruption was established. Wollenberger and coworkers further presented research where synthetic musks and brominated flame retardants were studied using the same test with A. tonsa [80,81]. In these studies, sublethal effects were also recorded at concentrations far below the level of acute lethal toxicity, but no clear evidence for endocrine disruption could be established.
5.3.5 Amphipods
A few studies have been undertaken to study potential endocrine disruption in amphipods. For instance, Brown et al. [82] found that exposure to 4-nonylphenol reduced growth in Corophium volutator but concluded that this was likely a general toxic response rather than an interaction with the molting hormones. They also reported that males exposed to 4-nonylphenol had larger second antennae than those in control treatments and suggested that the compound may have acted on the androgenic gland. Ford et al. [83,84] have also suggested that intersexuality observed in field-collected Echinogammarus marinus could be linked to endocrine disruption. Jacobson and Sundelin [85] have tested the known endocrine disruptor fenarimol for effects on reproduction and hormone (ecdysteroid) levels in the deposit-feeding amphipod Monoporeia affinis. Although fenarimol had a negative effect on fertilization rate and male mating ability at 0.3 mg/L, no effect on ecdysteroid levels could be observed.
5.3.6 Mysids
Mysids have been used quite extensively for the purpose of investigating endocrine disruption in crustaceans. For instance, Verslycke et al. [86] studied testosterone metabolism by mysids (Neomysis integer) and examined the changes in energy and testosterone metabolism after exposure to exogenously administered testosterone. Among other things, they found that N. integer can produce 11 monohydroxy testosterone metabolites and two nonpolar metabolites (androstenedione and dihydrotestosterone) but not the vertebrate estrogen 17β-estradiol. They also found the anabolic steroid β-boldenone, which had not been previously reported in invertebrates [86]. However, as for other crustaceans, the function of these steroids in mysids is not clear. Verslycke and coworkers also exposed N. integer to tributyltin and studied testosterone metabolism; they found nonpolar and polar metabolite induction at the lowest tested concentration (i.e., 10 ng/L) [87]. However, there were no significant differences from the control at higher concentrations and tributyltin had no effect on the elimination of testosterone by glucose conjugation. In a follow-up study, they investigated a set of known endocrine disruptors (i.e., testosterone, flutamide, ethynylestradiol, precocene, nonylphenol, fenoxycarb, and methoprene) for acute toxic effects in the same species and found 96-hour LC50 values in the range of 0.32 to 1.95 mg/L [88]. In addition to these experiments, they also studied short-term sublethal effects of methoprene and nonylphenol on energy and steroid metabolism. Both compounds significantly affected energy and testosterone metabolism of N. integer at concentrations below acute toxicity levels. The authors accordingly suggested that these end points could be adequate for measuring endocrine disruption in mysids, although no evidence for endocrine disruption was established.
Ghekiere and coworkers [89–91] have used N. integer to study effects on vitellogenesis, molting, and embryonic development following exposure to methoprene. In Ghikiere et al. [89], the authors used a novel quantitative enzyme-linked immunosorbent assay (ELISA) for vitellin in N. integer to study vitellogenesis and its potential disruption by xenobiotics. In addition to methoprene, gravid mysids were exposed to nonylphenol and estrone for 96 hours. All mysids exposed to methoprene (nominal concentrations of 0.01, 1, and 100 μg/L) had lower vitellin levels compared to the control animals, but this effect was not statistically significant. Exposure to nonylphenol resulted in significantly induced vitellin levels at the lowest concentration (0.01 μg/L), whereas no effects were observed at higher concentrations. Estrone significantly decreased vitellin levels at the highest test concentration (1 μg/L). Based on these findings, the authors acknowledged that mysid vitellogenesis can indeed be disrupted following chemical exposure but, due to difficulties in interpreting chemical-specific and concentration-specific responses, there is a need for better understanding of endocrine regulation of crustacean vitellogenesis. Ghikiere et al. [90] exposed juveniles (< 24 h) for three weeks to the same nominal concentrations of methoprene as those just described for adults [89]. On a daily basis, molts were checked and growth measured. Methoprene significantly delayed molting at 100 μg/L by decreasing the growth rate and increasing the intermolt period. This resulted in a decreased wet weight of the organism. The anti-ecdysteroidal properties of methoprene on mysid molting were also evaluated by determining the ability of exogenously administered 20-HE to protect against the observed methoprene effects. However, co-exposure to 20-HE did not mitigate the effects of methoprene on molting. In Ghikiere et al. [91], embryos were exposed to the same nominal concentrations of methoprene as those described for adults and juveniles [89,90]. Average percentage survival, hatching success, total development time, and duration of each developmental stage were analyzed. Based on the fact that embryos exposed to 1 and 100 μg/L had significantly lower hatching success and lower survival rates, the authors suggested their methods as a valuable tool for studying the impact of endocrine disruptors in mysids.
Earlier, McKenney and Celestial [92] used another mysid species—Mysidopsis bahia (which has since been reclassified as Americamysis bahia)—to study the effects of methoprene on survival, growth, and reproduction. They found that mysids exposed to a sublethal concentration (62 μg/L) were smaller, experienced a longer time to the production of the first brood, and produced fewer young per female than control animals. Furthermore, release of the first brood was significantly delayed by as much as three days in mysids exposed in the low μg/L range, and the total number of young produced by groups of mysids during their first brood was significantly reduced when mysids were reared at concentrations ≥8 μg/L. The most sensitive response was, however, a significant reduction in number of young produced per female at concentrations ≥2 μg/L. The authors suggested that the effects shown were related to endocrine disruption by methoprene, but, as for most of the other studies presented in this section, no clear evidence for endocrine disruption was established.
Recently, Hirano et al. [93] investigated the effects of environmentally relevant concentrations (i.e., 0.3–30 μg/L) of nonylphenol on survival, growth, sexual development, and molting (including assay of 20-HE levels) of seven-day old Americamysis bahia exposed over a period of 14 days. An effect on body length was observed already at 1 μg/L. At higher concentrations (10 and 30 μg/L), the number of molts was also significantly decreased relative to the controls and the production of 20-HE in premolting mysids was lower than those in control groups. At 30 μg/L, the nonylphenol disrupted female molt cycles. According to the authors, these findings were indicative that environmentally relevant concentrations of nonylphenol can disrupt growth via inhibition of 20-HE levels and associated delay in molting.
5.3.7 Other Studies Using Larger Crustaceans
As pointed out earlier, most of the information that is available on crustacean endocrinology is derived from studies using decapod species. However, very few studies are available on potential disruption of endocrine systems and pathways in decapods. Snyder and Mulder [94] exposed individual lobster Homarus americanus
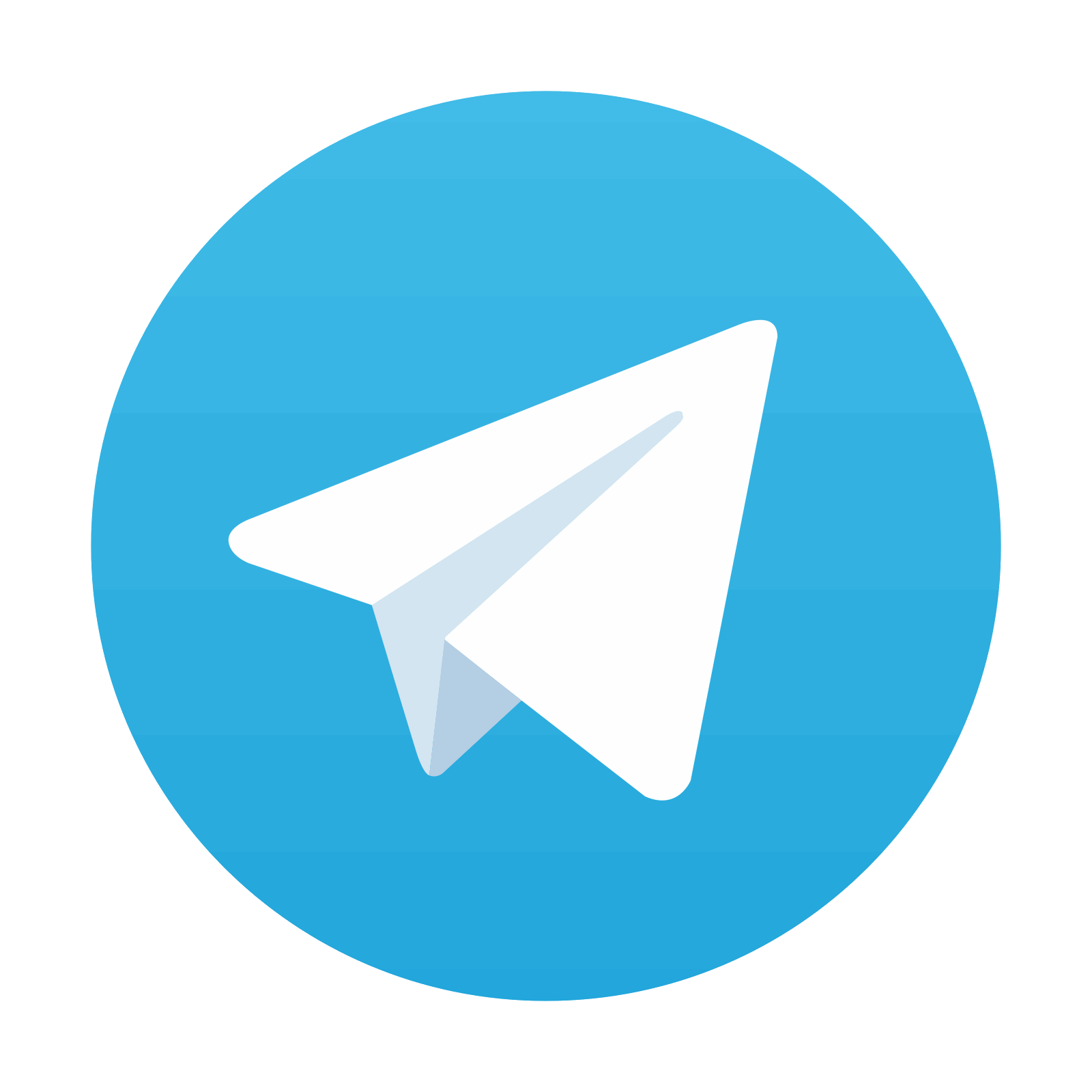
Stay updated, free articles. Join our Telegram channel

Full access? Get Clinical Tree
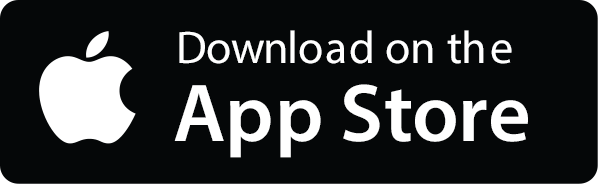
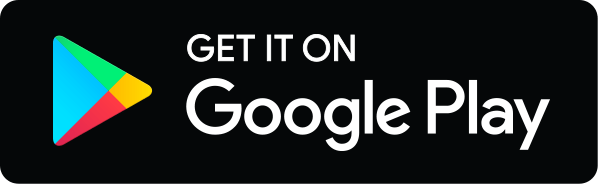