Critical Role of ABCG2 in ALA-Photodynamic Diagnosis and Therapy of Human Brain Tumor
Toshihisa Ishikawa*,†,1; Yoshinaga Kajimoto†; Yutaka Inoue‡; Yoji Ikegami‡; Toshihiko Kuroiwa† * NGO Personalized Medicine & Healthcare, Yokohama, Japan
† Department of Neurosurgery, Osaka Medical College, Takatsuki, Osaka, Japan
‡ Department of Drug Metabolism and Disposition, Meiji Pharmaceutical University, Tokyo, Japan
1 Corresponding author: email address: toshihisa.ishikawa.r@gmail.com
Abstract
Primary brain tumors occur in around 250,000 people per year globally. Survival rates in primary brain tumors depend on the type of tumor, patient’s age, the extent of surgical tumor removal, and other factors. Photodynamic diagnosis (PDD) is a practical tool currently used in surgical operation of aggressive brain tumors, such as glioblastoma and meningiomas, whereas clinical application of photodynamic therapy (PDT) to brain tumor therapy has just recently started. Both PDD and PDT are achieved by a photon-induced physicochemical reaction, which is induced by the excitation of porphyrins exposed to light. In fluorescence-guided gross-total resection, PDD can be achieved by the administration of 5-aminolevulinic acid (5-ALA) as the precursor of protoporphyrin IX (PpIX). Exogenously administered ALA induces biosynthesis and accumulation of PpIX, a natural photosensitizer, in cancer cells. However, ATP-binding cassette transporter ABCG2 plays a critical role in regulating the cellular accumulation of porphyrins in cancer cells and thereby its expression and function can affect the efficacy of PDD and PDT. In response to the photoreaction of porphyrins leading to oxidative stress, the nuclear factor erythroid-derived 2-related transcription factor can transcriptionally upregulate ABCG2, which may reduce the efficacy of PDD and PDT. On the other hand, certain protein kinase inhibitors potentially enhance the efficacy of PDD and PDT by blocking ABCG2-mediated porphyrin efflux from cancer cells. In this context, it is of great interest to develop ABCG2 inhibitors that can be applied to PDD or PDT for the therapy of brain tumor and other tumors.
1 Introduction
Brain tumors, in particular malignant gliomas, have a poor prognosis and are notoriously resistant to both chemotherapy and radiotherapy. Despite the development of concomitant chemotherapy and radiotherapy, the median survival time of glioblastoma patients is still limited to 14.6 months. The major cause of the poor prognosis of glioblastoma is due to the difficulties in performing the completely curative surgical resection and the intrinsic or acquired nature of tumor cells with chemo-/radioresistance.
In the 1960s, Lipson and Baldes introduced a hematoporphyrin derivative (HpD), a product derived following by the treatment of hematoporphyrin with a mixture of acetic and sulfuric acids and sodium hydroxide (Lipson & Baldes, 1960). Their development of the HpD established the basis of today’s photodynamic diagnosis (PDD) and photodynamic therapy (PDT; Gray, Lipson, Maeck, & Romeyn, 1967; Lipson, Baldes, & Gray, 1967; Lipson, Baldes, & Olsen, 1961, 1964; Sanderson, Fontana, Lipson, & Baldes, 1972). PDD and PDT are achieved by a photon-induced physicochemical reaction, which is induced by the excitation of photosensitizer exposed to light (Dolmans, Fukumura, & Jain, 2003; Dougherty et al., 1978).
In recent years, remarkable advances were made in PDD technology that makes it easier to reliably achieve complete excision of malignant glioblastomas (Floeth & Stummer, 2005; Shinoda et al., 2003; Stummer, Novotny, Stepp, Goetz, & Reulen, 2000) and meningiomas (Kajimoto et al., 2007). Fluorescence-guided gross-total resection has been developed and it reportedly prolonged the survival time of glioblastoma and meningioma patients (Floeth & Stummer, 2005; Kajimoto et al., 2007; Shinoda et al., 2003; Stummer et al., 2000, 2006; Stummer, Reulen, Vovotny, Stepp, & Tonn, 2003). Historically, two fluorescent agents, i.e., fluorescein sodium and protoporphyrin IX (PpIX) induced by 5-aminolevulinic acid (5-ALA) or its ester, have been used in glioma surgery. Because of its high tumor specificity and safety, ALA is particularly promising. It actively accumulates in the neoplasm and is converted to PpIX, which is fluorescent. This phenomenon has been clinically applied to the detection of neoplasms in the brain and other organs, such as the bladder, skin, and bronchus. Fluorescence-guided resection is considered beneficial for the removal of complicated or malignant tumors that have a high risk of recurrence.
2 Biosynthesis and Transport of Porphyrins
In our body, porphyrins and heme play critical roles in diverse biological processes, such as respiration and oxidative metabolism (Latunde-Dada, Simpson, & McKie, 2006; Tsiftsoglou, Tsamadou, & Papadopoulou, 2006). Heme is synthesized via an eight-stepped pathway that is spatially shared between mitochondria and cytoplasmic compartments (Fig. 1). Both biosynthesis and its intracellular concentration of cellular porphyrins and heme are tightly regulated. At the first step, ALA is synthesized from glycine and succinyl Co-A in a reaction catalyzed by ALA synthase and regulated by the intracellular-free heme pool. After ALA synthesis, a sequence of reactions occur leading to the production of various porphyrin compounds. Finally, as a result of ferrochelatase action, ferrous iron is incorporated into PpIX to form heme. Heme production, as well as the synthesis of its two immediate precursors (PpIX and protoporphyrinogen), occurs in the mitochondria. ABCB6, one of the human ABC transporters, reportedly transports coproporphyrinogen III from the cytoplasm to the mitochondria (Krishnamurthy, Xie, & Schuetz, 2007), whereas another ABC transporter ABCG2 is responsible for the cellular homeostasis of porphyrins and their related compounds (Wakabayashi, Tamura, Saito, Onishi, & Ishikawa, 2006). Disturbances in cellular porphyrin biosynthesis or metabolism are associated with several types of porphyria, which represent an elevation of the phototoxic hemeprecursors including PpIX (Dubakiene & Kupriene, 2006; Hindmarsh, 2003; Norman, 2005).
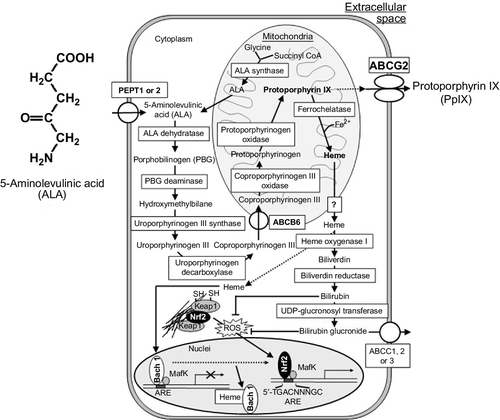
3 Enforced Biosynthesis of Protoporphyrin IX in Cancer Cells by ALA Administration
Exogenous ALA administration short circuits the first step of porphyrin biosynthesis (Fig. 1), where ALA is transported into cancer and normal cells by oligopeptide transporter 1 or 2 (PEPT1 or PEPT2; Döring et al., 1998; Novotny et al., 2000; Rodriguez et al., 2006; Whitaker et al., 2000). ALA induces the accumulation of detectable amounts of PpIX in certain types of cells, including cancer cells, making them photosensitive (Kennedy & Pottier, 1992). ALA-induced endogenous PpIX accumulation, thus, constitutes a photosensitization process in which the selectivity of neoplastic cells in synthesizing and/or accumulating PpIX may be exploited to enhance the efficacy of PDT and PDD.
ALA-induced PpIX biosynthesis has many other advantages. PpIX is an essentially monomeric compound with a high fluorescence yield (Schneckenburger, König, Kunzi-Rapp, Westphal-Frösch, & Rück, 1993) and photosensitizing capability due to its good singlet oxygen quantum efficiency (Pottier & Truscott, 1986; Weishaupt, Gomer, & Dougherty, 1976) and is rapidly metabolized in vivo (Loh et al., 1993). Animal and human studies have shown that ALA induces PpIX clearance from the skin within 24 h after systemic, topical, or intradermal administration (Kennedy & Pottier, 1992), whereas HpDs cause prolonged skin photosensitivity (1–2 months). In this context, ALA administration is advantageous with respect to drug safety.
4 PDD and Fluorescence-Guided Microsurgery
In PDD and fluorescence-guided neurosurgery (Floeth & Stummer, 2005; Kajimoto et al., 2007; Stummer et al., 2000, 2003, 2006), ALA is used for intraoperative labeling of the border regions of malignant gliomas infiltrated by alive clonogenic tumor cells and is helpful in precise resection of those regions. ALA is converted to PpIX in living cells and emits strong red fluorescence, with the excitation of blue–violet light. As PpIX preferentially accumulates in the tumor tissue in comparison with normal tissue, this red fluorescence becomes a good hallmark for discrimination between normal and tumor tissues, especially in malignant gliomas, which have infiltrative characteristics. Approximately 80–90% of the malignant gliomas show this red fluorescence in surgery (Fig. 2A), while only a limited number of metastatic brain tumor cases do not (Fig. 2B). In the surgery for metastatic brain tumor and lesionectomy for radiation necrosis and neurodegenerative disease, white matter around the lesion shows weak and vague fluorescence, which also provides us with a hallmark in the surgery. Additionally, in meningioma, some tumors showed the red fluorescence, which is especially helpful in the removal of the infiltrative portion in the bone and normal parenchyma (Kajimoto et al., 2007). Clinical data indicate that ALA-PDD-assisted resection of malignant gliomas results in statistically significant prolongation of postoperative survival (Stummer et al., 2003, 2006).
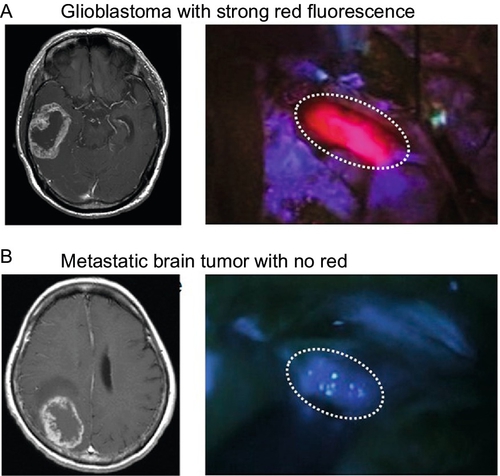
There is a question still remaining unanswered, namely: Why does PpIX accumulate in the tumor tissue more preferentially than in normal tissue? Until recently, little has been known about the molecular mechanisms underlying PpIX accumulation in clinical malignant brain tumors following 5-ALA administration. We have hypothesized that malignant brain tumors might exhibit distinct gene expression patterns associated with activated enzymes or transporters in the porphyrin biosynthesis pathway and that such differences in genetic expression patterns would represent the fluorescence intensity in malignant brain tumors. It has turned out that mRNA levels of PEPT2, ABCB6, and protoporphyrinogen oxidase elevated in many brain tumors, including brain tumor stem-like cells.
5 Oxidative Stress-Mediated Gene Expression in PDT
PDT utilizes porphyrin derivatives to generate singlet oxygen (1O2) and other reactive oxygen species (ROS) through visible light irradiation in cancerous tissues (Dolmans et al., 2003; Dougherty et al., 1998). Multiple signaling cascades are concomitantly activated in cancer cells exposed to photodynamic stress. Dependent upon the subcellular localization of cytotoxic ROS, those signals are transduced into adaptive or cell death responses (Buytaert, Dewaele, & Agostinis, 2007). Currently available evidence indicates that PDT can kill cancer cells directly by the efficient induction of both apoptotic and nonapoptotic cell death pathways (Dolmans et al., 2003). The identification of molecular effectors that regulate the cross-talk between cell death and cell protection pathways is an area of intense interest in the field of photodynamic killing of cancer cells.
In response to oxidative stress, nuclear factor erythroid-derived 2-related factor (Nrf2) is known to play a role in transcriptional upregulation of many target genes essential for cellular defense (Motohashi & Yamamoto, 2004; Toyoda et al., 2008). Nrf2 is a basic region leucine zipper (bZip)-type transcription factor (Itoh, Igarashi, Hayashi, Nishizawa, & Yamamoto, 1995; Moi, Chan, Asunis, Cao, & Kan, 1994), which targets the antioxidant-responsive element (ARE) containing the consensus sequence of 5′-A/GTGACNNNGC-3′ (Nguyen, Sherratt, Nioi, Yang, & Pickett, 2005). On the other hand, the Kelch-like ECH-associated protein 1 (Keap1) acts as a negative regulator of Nrf2 by retrieving it from the cytoplasm. Oxidative stress and/or electrophilic attack lead to the dissociation of Nrf2 from Keap1 and thereby activate Nrf2 for transcriptional regulation of ARE-dependent genes. The induction of ARE-regulated genes is under the control of Nrf2. Indeed, many genes encoding detoxifying and antioxidant enzymes were found to be regulated by Nrf2 (Kobayashi et al., 2006; Kobayashi & Yamamoto, 2006). It has recently been reported that mRNA levels of ABC transporters ABCC1, ABCC2, ABCC3, and ABCG2 were significantly elevated under oxidative stress and that translocation of Nrf2 into the nucleus was associated with the induction of ABCG2 (Adachi et al., 2007). Nrf2 interacts with the ARE located in the human ABCG2 gene promoter region. Nrf2-specific siRNA treatments suppressed the induction of ABCG2 and hemeoxigenase (HO-1) expression after the photoactivation of porphyrins in vitro. These findings strongly suggest that the induction of ABCG2 expression under oxidative stress is mediated, at least in part, by the Nrf2/Keap1 system.
The activation and nuclear translocation processes of Nrf2 seem to be more complex. Activation of the Nrf2 protein may involve at least three distinct pathways (Ishikawa et al., 2013), as shown in Fig. 3. Pathway 1: oxidation of critical cysteinyl residues of the Keap1 protein with concomitant inhibition of the ubiquitination activity of Keap1 (Kobayashi & Yamamoto, 2006; Yamamoto et al., 2008). Pathway 2: phosphorylation of the Nrf2 protein via protein kinases, such as p38MAPK, phosphoinositol-3-kinase (PI3K), protein kinase C (PKC), and RNA-dependent protein kinase-like ER kinase (PERK; Andreadi, Howells, Atherfold, & Manson, 2006; Bloom & Jaiswal, 2003; Cullinan & Diehl, 2004; Kang, Lee, Park, & Kim, 2002; Kang et al., 2007; Kocanova et al., 2007; Martin et al., 2004). Pathway 3: direct binding of heme to Bach1 and the facilitation of Nrf2/small Maf heterodimer formation (Hintze, Katoh, Igarashi, & Theil, 2007; Igarashi & Sun, 2006; Kitamuro et al., 2003; Ogawa et al., 2001; Oyake et al., 1996; Reichard, Motz, & Puga, 2007; Shan, Lambrecht, Donohue, & Bonkovsky, 2006; Sun et al., 2002, 2004; Suzuki et al., 2004; Zenke-Kawasaki et al., 2007). Thus, it is important to understand the molecular mechanisms underlying the activation of Nrf2 in cancer cells so as to understand the nature of ABCG2-mediated drug resistance of human cancer.
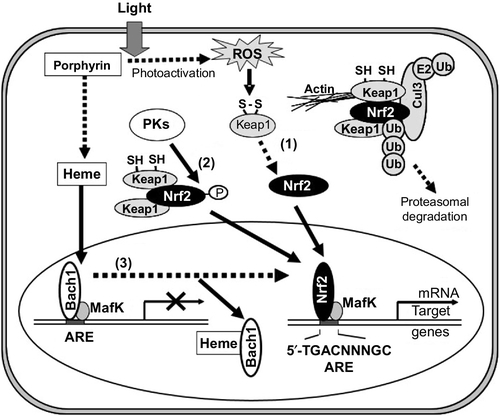
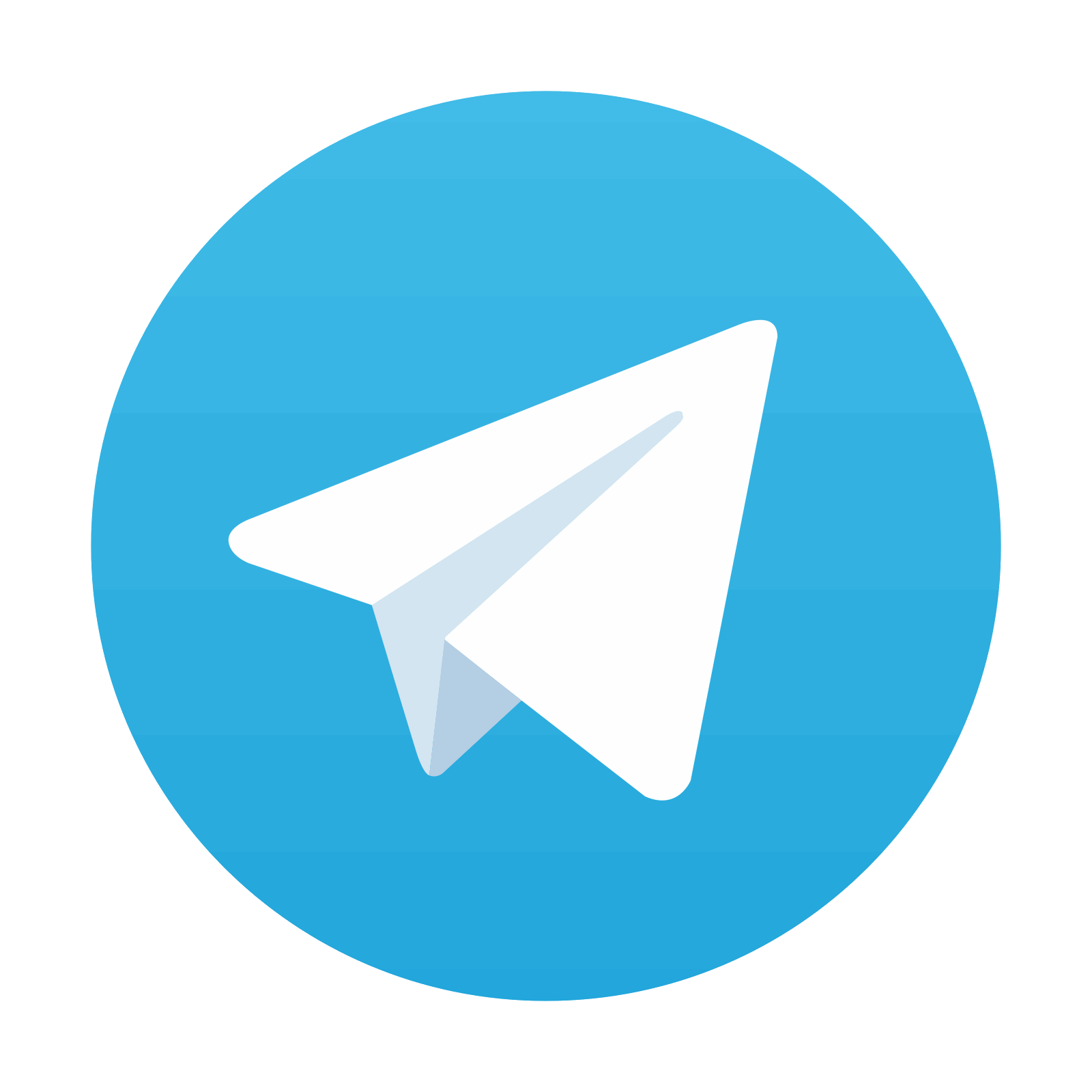
Stay updated, free articles. Join our Telegram channel

Full access? Get Clinical Tree
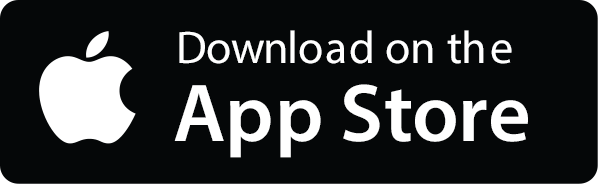
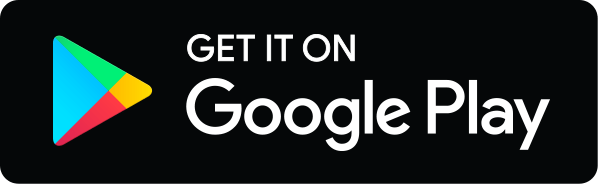