COMPARING AND CONTRASTING THE THEORIES OF AGING
PEARLS
❖ Hayflick and Moorhead conducted a landmark study that showed that in culture human fibroblasts have a limited life span.
❖ Neuroendocrine and hormonal theory regards functional decrements in neurons and their associated hormones as central to the aging process.
❖ According to the free radical theory, free radicals accumulate with age and cause destruction to important biological structures through an oxidative process.
❖ The caloric restriction theory prescribes that an individual gradually loses weight until a point of maximum metabolic efficiency is reached for maximum health and life span.
❖ The error theory specifies that any error in the process of making proteins will cascade into multiple effects.
❖ The somatic mutation theory hypothesizes that genetic damage will result from radiation and that radiometric agents accumulate and create functional failure and death of the organism.
❖ In the cross-linkage theory, cross-linkage of macromolecules is responsible for secondary and tertiary aging.
The search for the arcanum of that nemesis called old age has enticed many scientists to theorize and experiment with possible explanations for the mechanisms involved in the aging process. What determines how we age? Are there some biological prognosticators that determine how quickly and/or how well we age? Is aging programmed or unprogrammed in our genetic makeup? How do external factors affect our predisposed genetic makeup? The study of gerontology is a relatively new discipline, and the excitement of exploring its territory has seduced many scientific minds to explore potential explications of how the human species ages. Several theories of aging have been proposed; the most prominent theories will be reviewed and compared in this chapter. With the decoding of the human genomes, many new theories are evolving related to the potential of correcting gene coding that potentiates disease in old age and the possibility of extending the length of life. This critical look at current theories will provide a basic framework for understanding and critically evaluating the subsequent chapters on normal and pathological aging as well as clinical observations and strategies for managing the care of older individuals.
HISTORICAL PERSPECTIVE
The study of aging has a long history. Much of the research in the biology of aging has focused on prolongation studies rather than on the actual mechanisms of aging. Until recently, nonprogrammed theories of biological aging were popular because of the widespread perception that the evolution process could not support the development and retention of programmed aging in mammals. However, newer evolutionary mechanics theories including group selection, kin selection, and the evolvability theory support mammal programmed aging, and multiple programmed aging theories have been published based on the new mechanics. Some proponents of nonprogrammed aging still contend that their nonprogrammed theories are superior despite the new mechanics concepts. However, as summarized here, programmed theories provide a vastly better fit to empirical evidence and do not suffer from multiple implausible assumptions that are required by nonprogrammed theories. This issue is important because programmed theories suggest very different mechanisms for the aging process and therefore different mechanisms behind highly age-related diseases and conditions such as cancer, heart disease, and stroke.1,2 In a comprehensive historical monograph by Freeman,3 research on aging over the past 2500 years is presented. Subsequent historical reviews of theories of aging are reported by Austad2 and Harman.4 The reader is referred to these sources for a more in-depth review on the history and progress of research on aging.
Research on aging began around the turn of the 20th century.3 Metchnikoff introduced the concept that aging was caused by the continuous absorption of toxins from intestinal bacteria, and he received the Nobel Prize in 1908 for his contributions to biology and the study of aging. Systematic studies that described the aging phenomenon in terms of cell morphology, physiology, and biochemistry began around 1950.5 There was an improvement in experimental designs that led to more accurate definitions of valid and reliable hypotheses. Population aging studies revealed an opportunity (ie, interventions) to disrupt the fundamental biology of aging that could significantly delay the onset of age-related conditions as a group and, as a result, extend the healthy life span, or health span. There is now considerable evidence that cellular senescence is an underlying mechanism of aging and age-related conditions.5 Cellular senescence is a process in which cells lose the ability to divide and damage neighboring cells by the factors they secrete, collectively referred to as the senescence-associated secretory phenotype. Theorists have discussed the concept of cellular senescence; reviewed the evidence that implicates cellular senescence and the senescence-associated secretory phenotype in age-related deterioration, hyper-proliferation, and inflammation; and proposed that this underlying mechanism of aging may play a fundamental role in the biology of frailty. Two major groups of aging theories have evolved from these studies. The first group of aging theories is termed fundamentalist or developmental genetic theories. These theories are based on the premise of programmed “wear and tear”6 because aging is attributed to pathological decrements that are tissue specific (eg, connective, nervous, vascular, and endocrine tissue). The second group of aging theories views aging as an epiphenomenon in which environmental insults such as gravity, toxins, and cosmic rays result in the aging process. These theories are termed nongenetic unprogrammed or stochastic theories of aging. Many additional theories not contained in these 2 theoretic groups (although they could be arguably forced into one of the preceding categories) view aging as a continuum with development and morphogenesis,7 whereas others relate aging to a cessation of somatic cell growth8 and energy depletion or energy excess.9
Current modified versions of these theories have been introduced that involve the immune system, the neuroendocrine system, failures in DNA repair, random mutation in somatic cells, errors in protein synthesis, and random damage from free radicals.10 Specific theories related to sleep and the rate of aging, growth hormone (GH), dehydroepiandrosterone (DHEA), telomeres, and cell culture models of aging involving specific proteins have evolved. The most recent theories identify genetic characteristics (genotypes) and their effects on survival and the rate of change.11
These theories, although often presented as separate from each other, are not mutually exclusive. Clearly, there has not been one theory that fully identifies all of the causes, mechanisms, or bases of aging. The processes regulating the rate of aging may be different in different cell types and different tissues, and the combined effects of environmental damage and intrinsic pathologies may further obscure a fundamental mechanism for aging. Clear mechanisms of aging depend on interdependent sets of variables and causes.
From a historical perspective, the concept of aging as a cell-based phenomenon vs organismic aging is comparatively new. The emphasis on the distinction between somatic cells that age and germ cells that do not age, which suggested that the inability of somatic cells to replicate indefinitely was the reason for the limited life span in humans,8 was brought under serious questioning because of a flawed series of experiments by Carrel et al12–14; the study of cellular aging was set back by this historic event in biological science. In 1912, Carrel and Ebeling13 began a series of experiments using normal chick embryo fibroblasts cultured in vitro. Based on this series of experiments, it was thought that fibroblasts could replicate indefinitely and were immortal. The findings were considered to be compelling evidence that individual cells could live forever. This hypothesis dominated gerontology until 1950, and it was an accepted fact that cells did not characteristically age. It was thought that cells were immortal in isolation and that it was the tissues that were involved in aging. Scientists later discovered that the method of preparation of the chick embryo extract by Carrel was contaminated with the continual addition of fresh embryonic cells. The result was erratic miotic activity coinciding with the periodic addition of chick embryo extract.15 In summary, these cells lived forever because young embryo cells were mixed with the older prepared culture. Many theories have evolved since.
In 1961, a landmark study by 2 then-unknown cell biologists, Hayflick and Moorhead,16 turned the study of senescence of cultured cells completely around. They concluded from their in vitro studies of fetal fibroblast cells (lung, skin, muscle, and heart) that human fibroblasts have a limited life span in culture. Hayflick and Moorhead reported that there was a period of rapid and vigorous cellular proliferation consistently followed by a decline in proliferative activity and characteristic senescent changes (ie, decreased replication and wasting). They proposed that aging was a cellular as well as an organismic phenomenon. The loss in functional capacity of the aging person reflected the summation of the loss of critical functional capabilities within individual cells. For instance, the loss of type II fibers in the muscle with aging results in atrophy and a decrease in the muscle force production, thereby decreasing functional capacity. The Hayflick and Moorhead experiment altered the direction and interpretation of aging research and cellular biology. In essence, they were among the first scientists to change the philosophy of modern biology and gerontology. From this basis, the subsequent theories discussed evolved.
ANIMAL MODELS OF AGING
The tortoise and lobster exhibit remarkably few features of aging, albeit for reasons not yet known. In fruit flies, the activation of the Methuselah gene confers approximately a 30% extension of longevity. In the laboratory, neuronal sprouting can be stimulated by either apolipoprotein E3 or E4, whose production is increased by estrogen.17 Apolipoprotein E transgenic knockout mice (mice without these apolipoprotein) do not exhibit neurite outgrowth in response to estrogen. This finding may be pertinent to estrogen’s presumed role in the prevention of neuronal aging and Alzheimer disease. Estrogen also induces glial fibrillary acidic protein in astrocytes and their abutment and retraction from regulatory gamma-aminobutyric acid–producing terminals that synapse on gonadotropin-releasing hormone neurons in the young female rat but not in older animals that have lost the capacity to generate a luteinizing hormone (LH) surge.17 The important new concept of defective hormone-sensitive neuronal plasticity with aging will require further experimental appraisal.
Theories of dietary restriction (DR) determined that restricting dietary intake potentially increased life span in many types of animals.18 It has been suggested that the response to chronic DR may be an adaptation to environments with variable food levels. The ability to slow aging when food levels are low purportedly postpones reproductive senescence and therefore allows reproduction when food levels are high.19 In early studies, Harrison and Archer19 predicted that the response to DR should be strongest in species with short reproductive life spans because these species should be more likely to encounter periods of low food that are longer than their normal reproductive life spans. In contrast, Phelan and Austad20 noted that reproductive senescence may be uncommon in nature and suggested that the increase in life span in response to DR is not an adaptation to variable food levels but is instead a “secondary consequence of its effect in delaying age at maturity and decreasing the subsequent rate of reproduction.” They predicted that the response to DR should be strongest in those species with early maturity and high reproductive rates. Recent investigations into animal models of aging with DR found that most species, but not all, responded to DR by increasing the mean life span, maximum life span, reproductive life span, mortality rate doubling time, and initial mortality rate.18 Intertheory-specific comparisons did not show the predicted correlations between the strength of the response to DR and the reproductive life span, age of first reproduction, or total reproduction. There was support for the idea that the response to chronic DR is associated with changes in reproductive allocation during short-term periods of starvation. In other words, species that reduced reproduction when starved increased their life span under DR, whereas species that continued to reproduce when starved decreased their life span under DR.18
Specific gene studies provide a foundation that provides convincing proof for age- and genotype-specific effects on gene expression and longevity. Studies reveal key similarities in genetic makeup among the growing number of animal models of decelerated aging.21 Wang and colleagues21 recently refined the RNA population model emphasizing the roles of noncoding sequences and repetitive sequences in gene expression regulation as it produces the effects of aging. It is a simple model referred to as the RNA population model, which can effectively explain multiple biological phenomena and has the potential to explain research on aging, functional genomics, and the functions of noncoding RNA sequences, as will be discussed in subsequent chapters.
FUNDAMENTAL CONSIDERATIONS
A discussion of the theories of aging must include a review of the observations and correlations associated with the aging process. A great deal is unknown about the nature of the mechanisms involved in aging; therefore, the study of aging is intrinsically difficult. The myriad of aging scenarios and trajectories that occur in nature, the different combinations of environmental and intrinsic changes, the lack of measurable transition points or biomarkers to define the kinetics of the process of aging, and the lack of a measurable means for aging other than death all serve to obscure any unifying principles.
It is even difficult to define aging, although it is characterized by an increasing vulnerability to environmental changes, and it is known that increasing chronological age results in functional losses and increases the probability of death.22 There are several underlying assumptions related to theoretic gerontology. The following fundamental considerations are an important basis upon which to build further knowledge in the studying of aging.
Aging is developmental. This concept is very simple—we do not suddenly age. Our aging time capsules do not ignite at the age of 65. We evolve into mature adults and grow older developmentally, not chronologically. Aging is unique among all developmental stages. A 70-year-old person in chronological age may have the physiological makeup of a 50-year-old person. Yet, a 50-year-old person with chronic diseases may parallel the physiological decline of a 90-year-old person.
Old age is a gift of 20th century technology and scientific advancement. Many gerontologists and researchers in aging purport the notion that the extended life expectancy that we now have is really a gift of modern medicine and technology. Some biologists argue that the survivorship kinetics of biological aging may be an artifact of civilization and domestication. In nature, populations of species (including humans) only recently began to live long enough to show the characteristic kinetics of biological aging. Since the discovery of insulin, vaccination for diseases, improved sanitation, a decline in infant mortality, and the development of modern surgical techniques and advanced treatment modes for formerly fatal diseases, humans now experience longer lives. We are also staying older longer (not younger longer) throughout the 20th century. The whole phenomenon of aging is new. Now that we have entered the 21st century, it is expected that longevity will significantly increase to the point where humans can expect to live well into their 9th and 10th decades.2,6,7
The effects of normal aging vs pathological aging must be differentiated if possible. A confounding problem in understanding aging is the fact that there is a vast spectrum of aging changes. The process of aging is probably multifactorial in its regulation; however, it is virtually impossible to tell which changes are primary to a senescence-regulated event and which are secondary. Often we assume that a functional decline is due to aging. However, disease may often cause functional decline, which is not a normal aging process. For example, if one has adult-onset diabetes, then the probability of cardiovascular disease increases as a result of the effect of the diabetes. It is not “normal” to get diabetes; it is a function of lifestyle and heredity. Confusion exists between aging, disease, and dying. Aging characteristically brings a loss in homeostasis and with it increased vulnerability to diseases, some of which result in death. Death has been used as the end-point measurement of aging; however, death can occur from many causes, some of which are related to the aging process only secondarily and in some cases totally unrelated to the process, as is true of accidents.
There is no universally accepted theory of aging. Aging does not occur in all species or in all organisms of the same species in exactly the same way. While one tissue may be losing functional capacity rapidly, others may be comparatively quite “young” functionally. (A more comprehensive discussion of this is presented in Chapter 3.) Although aging is a universal phenomenon, no one really knows what causes it or why we age at different rates. Chronological age is a much less useful and definitive measure of functional capacity than scientists would like.
Amid all these confusing assumptions of aging, a set of consistent aging characteristics has been identified. First, there is an increased mortality with age.23 Second, consistent changes in the biochemical composition and biomechanical aspects of the body with age have been well documented,24 including a decrease in lean body mass and an increase in fat. There are characteristic increases in lipofuscin in certain tissues and increased cross-linking in matrix molecules such as collagen.24 Third, a broad spectrum of progressive deteriorative changes have been demonstrated in cross-sectional and longitudinal studies.25 (These changes will be discussed in detail in Chapter 3.) The availability of a set of biomarkers that really reflect underlying aging processes would be of much value in disentangling age-associated pathology from specific aging mechanisms. In a review by Engelfriet and colleagues,25 a survey of the literature was performed to identify promising biochemical markers of aging, with a particular focus on using them in longitudinal studies of aging in humans that entail repeated measurements on easily obtainable material, such as blood samples. This search strategy was a 2-pronged approach, one focused on general mechanisms of aging and one including studies on clinical biomarkers of age-related diseases.25 Fourth, there is a reduced ability to respond adaptively to environmental change (perhaps the hallmark of aging). This can be demonstrated at all levels from an individual molecule to the complete organism.26,27 Thus, the changes of age are not so much the resting pulse rate or the fasting serum glucose but rather the ability to return these parameters to normal after physiological stress. Lastly, there is an increased vulnerability to many diseases with age,27,28 which occurs at the cellular level. Aging is a process that is distinct from disease. The fundamental changes of aging provide the substratum in which age-associated diseases can flourish.
Aging theories can be divided into 2 major categories: genetic (programmed) and nongenetic (nonprogrammed). Genetic theories focus on the mechanisms for aging located in the nucleus of the cell, whereas nongenetic theories focus on areas located elsewhere, such as in organs, tissues, or systems within the body, or extrinsic environmental causes. In order to understand both of the theories of aging, a basic understanding of 3 somatic cell types is necessary.
Not all somatic cells age at the same rate nor do they have similar aging characteristics. Somatic cells are divided into 3 categories: (1) continuously proliferating or mitotic cells, (2) reverting postmitotic cells, and (3) fixed postmitotic cells.27 Continuously proliferating mitotic cells never cease to replicate themselves, and injury done to these cells is healed through regeneration. Such cells can be found as superficial skin cells, red blood cells, cells of the lining of the intestine, and bone marrow cells. Reverting postmitotic cells have a slower rate of division than continuously proliferating cells, but when there is injury, the rate of division is sped up and regeneration is possible. An example of these are kidney and liver cells. The final type of somatic cells, fixed postmitotic cells, never replicate once the cells reach maturity.15,27 Muscle cells and nerve cells are primary examples of fixed postmitotic cells. Humans have all of the fixed postmitotic cells they will ever get once they have reached biological maturity (eg, for women at the time of menses and for men at the time they stop growing).
In our adult life, nerve and muscle cells replicate and repair themselves only if the nucleus is intact. Because the postmitotic cell will not replicate itself, no new vital cells are produced. Therefore, the need for residual fixed somatic cells to remain vital is crucial to the well-being and life expectancy of the individual.
THEORIES OF AGING
Historically, one of the major problems in gerontology is the ease and frequency with which new theories have appeared.28 There is not one theory that fully explains the process of aging. Researchers have viewed aging as an event that happens rather than as a period in the life of organisms that begins at maturity (or conception) and lasts for the rest of the life span. It seems that aging should be considered a developmental process. Numerous primary and secondary changes occur during development and aging. Some changes are programmed and directed within the body, whereas some changes are caused by the environment. Understanding aging and formulating coherent, testable hypotheses require that various aspects of aging be dissected from each other and examined critically. Different authors use different classification systems for the theories of aging. All are useful, and all have inherent difficulties. One effective way to present this information is to group the multiplicity of theories into the 2 classes of theories based on their fundamental conceptual basis, as described previously, and then describe prominent examples of each of these categories. The reader is reminded that this classification is operational only and that neither the categories of theories nor the theories themselves are mutually exclusive.
Developmental Genetic Theories
The developmental genetic theorists consider the process of aging to be part of a continuum with development genetically controlled and programmed. Opposition to this idea comes from 2 sources. Some researchers believe that there is a selection for aging mechanisms through evolution. In other words, aging processes are controlled extrinsically by environmental influences. The second source is the result of an intuitive sense that the diverse scenarios and trajectories of aging are not likely to be controlled by one process whose mechanisms regulate the precise sequence of development29 (ie, aging is not dependent on a single developmental process but rather influenced by multifactorial ascendants). It ascribes senescence to unremitting, nonprogrammed change or remodeling forced upon the adult soma by postmaturation expression of developmental gene(s) affecting dynamic transformation of the single-celled origin into a complex, multicellular organism. Although persistent somatic change is necessary for development to proceed normally, it also has the potential to erode homeostasis in adults after maturation is complete. Thus, developmental inactivity is the primary cause of senescence, whereas decay of internal order and integrated function among interdependent systems of the body is the general mechanism by which aging progresses over time. Accordingly, this global pathogenic process creates an environment in which the many recognized, age-associated physiological and metabolic sequelae can arise as consequences of senescence rather than causes of it. Paradoxically, the genes that promote somatic remodeling essential for development and survival also guarantee aging and death by the same action whose outcomes differ only by the time it is expressed relevant to maturation.29
There is no question that environmental factors can regulate or modify mortality and in some cases influence the rate of aging (eg, the aging of the skin is accelerated by exposure to the sun); however, the dimension of aging controlled by genetic processes clearly has some solid foundations. Gerontologists agree that the maximum life span and the rate of aging are regulated intrinsically. The primary evidence of this is the species-specific maximum life span. Variation in life span is far greater among species than within species. Because maximum life span is a species characteristic, it would seem evident that this is genetically determined. Further supporting evidence for the genetic basis of aging and maximal life span comes from the recognition of genetic disease of precocious aging, such as in genetic progeroid syndromes.30 Because premature aging (progeroid) syndromes, including progeria, mimic many of the characteristics of human aging, research into these conditions has proven to be very useful not only to identify the underlying causal mechanisms and identify treatments for these pathologies but also for the study of physiological aging. The classic progeria—Werner syndrome, Hutchinson-Gilford syndrome, and Down syndrome—are among these diseases.30 The precise mechanism of human aging is not replicated at an accelerated rate in these individuals; however, many of the commonly recognized aging changes occur more rapidly. These diseases are important probes in the study of aging.
Of interest are studies that compare the longevity of monozygotic and dizygotic twins and nontwin siblings. It has been shown that there is a remarkable similarity in longevity between human fibroblast cell cultures in monozygotic twins that is not demonstrated in the other 2 groups.31 A greater similarity of cell behavior and replicative life span is observed in these twins when compared with nontwin, age-matched controls.31 One could argue that genetics governs susceptibility to certain diseases but not to aging per se. Alternatively, a certain “vigor” could be inherited that protects against the developmental susceptibility to a wide variety of diseases. It clearly is difficult to distinguish between the 2 mechanisms. Nonetheless, there is circumstantial evidence for genetically controlled mechanisms of aging that potentially operate in a similar way to developmental processes.
Hayflick Limit Theory
Hayflick and Moorhead16 were able to show that a deterioration in cells (eg, mitotic and mitochondrial activity) was not dependent on environmental influences but rather that cell aging was intrinsic to the cells. They found that there was a limited number of cell population doublings or replications ranging from 40 to 60, with the average doubling being 50 per life cycle of the cell. The developmental senescence process of cultured cells includes 3 phases. Phase I is the beginning stage of cell life, phase II involves rapid cell proliferation, and the final cessation of cell division occurs in phase III. Hayflick and others have repeatedly shown that phase III is nearly always between 40 and 60 population doublings for embryo cells. Hayflick noted alterations and degeneration occurred within the cells before their growth limit,32 which were evident in the cell organelles, membranes, and genetic material. Hayflick demonstrated that functional changes within cells are responsible for aging and that the cumulative effect of improper functioning of cells and the eventual loss of cells in organs and tissues is probably responsible for the aging phenomenon. Transformation can occur at any point in the life of the cell. Cells acquire a constellation of abnormal characteristics, including chromosomal abnormalities and an indefinite life span (eg, properties of tumor cells). The generality of the Hayflick phenomenon is that, in the absence of transformations in the cells, senescence always occurs.
Hayflick’s limit of cellular life has served as a model to other investigators who have shown that cell replication potential is a function of donor age.33,34 In other words, an inverse correlation exists between donor age and the population doubling potential,34 and this correlation is directly related to the maximum life span of the species.35 Evidence of the Hayflick limit has also been seen in cultures taken from individuals with progeria (eg, Hutchinson-Gilford syndrome and Werner syndrome). Because progeria is a premature aging syndrome, the decreased life span of the donor cells shows a lower Hayflick limit, as expected.30 The length of the longest life span for different species and the number of cell replications are correlated. For example, the Galápagos tortoise has a maximum life span of 175 years with a maximum doubling of 125, whereas the human maximum life span is 110 years with a maximum doubling of 60.36
The relationship between the cellular aging phenomenon in culture and aging of the individual is not specific to fibroblasts. It has been demonstrated in smooth muscle cells, endothelial cells, glial cells, and lymphocytes. Several changes that occur during in vitro senescence are parallel to those that occur in vivo with advancing age. However, it is not clear if the observed cellular aging in culture actually contributes to what we recognize as the in vivo aging phenomenon of the organism. There is no evidence to support that the in vitro replication life span of mesenchymal cells is important in determining the life span of the organism. Contrary to this is that living cells maintain their functional capacity through processes of replacement and repair over long periods of time. Proliferative homeostasis needs to be evaluated with regard to the ability of the organism to respond to environmental stresses. It is also important to keep in mind that the aging of proliferating or mitotic cells may be quite different from that of fixed postmitotic or reverting postmitotic cells. The results of the studies cited earlier suggest that, underlying the effects of various proposed “master timekeeping” systems, cells have individual “clocks” that ultimately limit their life span.15,30,32
Evolutionary Theory
The evolutionary theory of aging is an expansion of the concept of natural selection as Darwin proposed decades ago.37 This theory predicts that the frequency for deleterious mutations of genes will reach equilibrium from one generation to the next. In other words, there will be an increase in the age of onset of mutation of a gene because of postponed selection. This makes each successive generation more resistant to mutations, and when mutations do occur, they occur at a later age.38 According to the mutation hypothesis, one would expect the genetic variability for survival to increase with age. The life expectancy of the human species has clearly increased since the 1900s. However, the importance of confounding variables (eg, better sanitation, immunization, and technological advances) must be considered. Often the theory of evolution and a concurrent hypothesis may apply to the aging of the same individual.39,40 A delay in the onset of mutations may also be dictated by the health of the organism or, as Darwin hypothesized, survival of the fittest.
One theory under the evolutionary model, the network theory of aging, extends the argument that a global reduction in the capacity to cope with a variety of stressors and a concomitant progressive increase in proinflammatory status are major characteristics of the aging process.41 This phenomenon, which Franceschi and associates41 refer to as inflamm-aging, is hypothetically provoked by a continuous antigenic load and stress. On the basis of evolutionary studies, these researchers argue that the immune and stress responses are equivalent and that antigens are nothing other than particular types of stressors. This theory proposes that the persistence of inflammatory stimuli over time represents the biological background (first hit) favoring the susceptibility to age-related diseases/disabilities. A second hit (the absence of robust gene variants or the presence of frail gene variants) is likely necessary to develop overt organ-specific age-related diseases having an inflammatory pathogenesis, such as atherosclerosis, Alzheimer disease, osteoporosis, and diabetes.41,42 Following this perspective, these researchers found several paradoxes in healthy centenarians, such as an increase of plasma levels of inflammatory cytokines, acute-phase proteins, and coagulation factors. Therefore, they concluded that the beneficial effects of inflammation devoted to the neutralization of dangerous and harmful agents early in life and adulthood become detrimental late in life.41,42
Stress Theory
Along this same line of thought, the stress theory of aging is also an evolutionary model. According to this theory, aging is considered in the context of the abiotic (ie, not containing or supporting life) stresses to which free-living organisms are normally exposed.43 Stress is defined in terms of adequate nutrition and exposure to environmental stresses. Assuming that the primary target of selection of stress is at the level of energy carriers, trade-offs under the rate of living theory of aging predict increased longevity from selection for stress resistance. Changes in longevity then become incidental to selection for resistance. Parsons44 suggests that the primary trait inherited is resistance to stress. Consequently, at extreme ages those with inherited resistance to abiotic stress should dominate, and the reduction in homeostasis manifested by the deteriorating ability to adapt to abiotic stress as aging proceeds should be slowest in those surviving the longest.43 In other words, survival to old age is enhanced by high vitality and resilience associated with substantial physiological and morphologic homeostasis.27 This is underlaid by genes for stress resistance, which confer high metabolic efficiency and hence adaptation to the energy costs of physiological and environmental stress.44
Neuroendocrine and Hormonal Theory
The endocrine system regulates body composition, fat deposition, skeletal mass, muscle strength, metabolism, body weight, and physical well-being. Multiple endocrine changes evolve with aging in all species, and, not surprisingly, some of the physiological manifestations of aging are related to the effects of declining hormone levels.
The ensemble view of the neuroendocrine axis in aging highlights the role of the hypothalamic-pituitary axis. The central nervous system regulates the pituitary gland, which secretes hormones to target tissues that, in turn, produce substances that feed back on the hypothalamic-pituitary axis. This feedback control network can be assessed via novel entropy statistics, which assess the ensemble synchrony of a feedback axis. E ntropy calculations quantify the progressive age-related loss of orderliness of single-hormone secretion of GH, a drenocorticotropin hormone (ACTH), LH, and insulin, as well as the erosion of coordinate 2-hormone secretion for ACTH and cortisol, LH and testosterone, LH and follicle-stimulating hormone, and LH and prolactin.45 LH secretion, sleep stage transitions, and nocturnal penile tumescence oscillations also exhibit marked loss of synchrony with aging.45
In humans, aging is associated with a decrease in the gonadal production of estrogen in women (menopause) and testosterone in men (andropause), the adrenal production of DHEA and DHEA sulfate (DHEA-S) (adrenopause), and a decrease in the activity of the GH–insulin-like growth factor axis (somatopause).46 (See Chapter 3 for further discussion of these “pauses.”) As a result, hormone replacement regimens are being developed as a strategy to delay or prevent some of the consequences of aging. However, in some cases, the use of hormone replacement therapy for this purpose is controversial.
Unknown mechanisms link aging-related parallel declines in the GH–insulin-like growth factor 1 (IGF-1) axis (somatopause), the gonadal axis (gonadopause), and adrenal androgen secretion (adrenopause). Epidemiologic studies reveal consistent decrements in adrenal androgen (DHEA and DHEA-S) secretion in aging men and women.47–49 However, neither the basis for nor the medical implications of this attrition in the adrenal zona fasciculata function are known.
The group of theories purporting the neuroendocrine basis of aging regards functional decrements in neurons and their associated hormones as central to the aging process. Given the major interactive role of the neuroendocrine system in physiology, this is an attractive approach.50 T his theory purports that the center of aging is located in the brain.41 Denckla51 bases his theory on past studies of hypothyroidism, a disease that mimics mature aging (eg, a depressed immune system, wrinkling of the skin, gray hair, and a slowed metabolic rate). Hypothyroidism can be fatal if untreated with thyroxine, inasmuch as all the manifestations of aging are evidenced.
Another aspect of the neuroendocrine basis of aging depends on the role of the pituitary hormones.51 The anterior pituitary gland controls the thyroid gland by the thyroid-stimulating hormone and thus the secretion of thyroxine. Thyroxine is the master rate-controlling hormone within the body for metabolism and protein synthesis. The focus of this theory is the proposed ability of the anterior pituitary to release a blocking hormone labeled DECO (decreasing oxygen consumption) that blocks the cell membrane from taking up thyroxine as a check balance in the human system. Denckla has not isolated the anti-aging serum, but what Denckla has given us is an alternative philosophic point of view in theoretic gerontology—the in vivo vs the in vitro controversy. Denckla believes that the aging process in vitro is an artifact and unrelated in any meaningful way to true aging. Denckla says, “I don’t care what happens to cells in tissue culture. What is important is what people die of.” He believes that for good evolutionary reasons—to ensure the turnover of generations so that mutations can take place that enable a species to adapt to a changing environment—nature cannot trust to chance (wear and tear). There must, therefore, exist “an absolutely failsafe killing mechanism without which the species would not survive.”28
An important version of Denckla’s theory proposes that the hypothalamic-pituitary-adrenal (HPA) axis is the master timekeeper for the organism and the primary regulator of the aging process. Functional changes in this system are accompanied by or regulated by decrements throughout the organism. For example, the cascade effect of functional decrements in the hypothalamus and their potential sequelae have been documented in humans.52 The neuroendocrine system regulates early development, growth, puberty, the control of the reproductive system, metabolism, and, in part, the activities of all the major organ systems. Support of the neuroendocrine and hormonal theories of aging is evidenced by the decline in reproductive capacity due to a decrease in the release of gonadotropin-releasing hormone by the hypothalamus, which appears to be the result of diminished activity of hypothalamic catecholamines.52 Similarly, it has been shown that the release of pulsatile GH declines with age.53 These changes would have profound effects on estrogen and progesterone release and subsequently on functional capacity. This theory suggests that there is a loss of neurons in discrete areas of the brain and a loss of neurotransmitter responsiveness by the remaining neurons.52,53
Satisfactory evidence to relate endocrine function to aging is not present to substantiate a significant contribution of endocrine gland function to the process of aging.53 In response to stress and trophic hormones, the adrenal cortex and thyroid gland remain intact. For women, menopause is a hormone-mediated event that chronicles but does not regulate aging.52,53 The ovary is the sole endocrine gland whose functional capacity predictably declines with normal aging. On the other hand, androgen production by the testes is not as predictable because there are individual differences.53
The importance of neuroendocrine research cannot be overemphasized. However, critics of these theories point out that the master timekeeper of aging, the neuroendocrine system, lacks universality. Many organisms that age (eg, higher vertebrates) have no complex neuroendocrine system. It can also be argued that the changes that occur in the neuroendocrine system are fundamental changes and occur in all tissues. However, aging of the brain produces additional secondary effects that, although not fundamental to aging, contribute to the development of the overall aging process. What the neuroendocrine system contributes to the aging process is evidenced by the effects of the lack of estrogen with respect to its effects on bone density and vascularity. Its contribution to other aging processes remains to be determined.
Theory of Intrinsic Mutagenesis
Another developmental genetic theory is referred to as the theory of intrinsic mutagenesis. This idea was first proposed in an attempt to reconcile stochastic theories of aging with the genetic regulation of maximum life span.54 This theory suggests that each species is endowed with a specific genetic constitution that “regulates the fidelity of the genetic material and its replication.”54 The degree of fidelity regulates the rate of appearance of mutations or errors, thereby affecting the life span. Alternatively, we can envision a case in which new “fidelity regulators” appear at different stages in an animal’s life. Each successive evolutionary set of regulators could have a decreased capacity allowing an increase in mutational events. Another aspect of intrinsic mutagenesis is concerned with the increase in DNA excision repair associated with maximal life span.54 There is evidence that the accuracy of DNA polymerase may decrease with age,55–57 but the research in support of both of these hypotheses is rather controversial.
Immunological Theory
The immunological theory of aging is another theory categorized as a developmental genetic theory. This theory was proposed by Walford58 and has 2 major observations upon which it is based: (1) the functional capacity of the immune system declines with age as a result of reduced T-cell function59 and a reduced resistance to infectious diseases, and (2) the fidelity of the immune system declines with age as evidenced by the striking age-associated increase in autoimmune diseases.58
As with the neuroendocrine theory, the immunological theory is attractive. The immune system has a primary integrative role and is of major importance in health maintenance. Conversely, life span differences could simply be due to the prevention of diseases. The same argument can be leveled at the immunological theory as the neuroendocrine theory. It lacks universality, and it is difficult to defend the immune system’s role as the primary timekeeper in the biology of all organisms. The inability to distinguish between primary and secondary effects on aging and the possibility that changes in the immune system are no different from changes in other cell types make interpretation of this theory difficult.
Free Radical Theory
There are more than 300 theories to explain the aging phenomenon. Many of them originate from the study of changes that accumulate with time.60–62 Among all the theories, the free radical theory of aging, which was postulated by Harman,63 is the most popular and widely tested. It is based on the chemical nature and ubiquitous presence of free radicals. This review aims to recapitulate various studies on the role of free radicals in DNA damage, both nuclear as well as mitochondrial; the oxidative stress they impose on cells; the role of antioxidants; the presence of autoantibodies; and their overall impact on the aging process.
What is a free radical reaction? Biochemical insults arise within aging cells, in part from the action of reactive oxygen species generated and scavenged incompletely throughout the cell cycle. Aging-associated changes occur between and among cells via alterations in the intercellular matrix, the intercellular exchange of trophic factors, the release of inflammatory cytokine mediators, and the degree of infiltration by other cell types (eg, glial interactions with neurons). It is not known why free radical damage does not adversely affect certain types of cells (eg, gonadal germ cells).63
The free radical theory is another example of a developmental genetic theory. Proposed aging changes are due to damage caused by radicals according to this theory. Basically, free radicals are highly charged ions whose outer orbits contain an unpaired electron. Chemically, they are highly reactive species that are generated commonly in single-electron transfer reactions of metabolism. Free radicals have been shown to damage cell membranes, lysosomes, mitochondria, and nuclear membranes through a chemical reaction called lipid peroxidation. Both membrane damage and cross-linking of biomolecules result from free radical chain reactions.64 The net result of free radical reactions, as summarized by Leibovitz and Siegel,65 is a decline in cellular integrity caused by reduced enzyme activities, error-prone nucleic acid metabolism, damaged membrane functions, and the accumulation of aging pigments (lipofuscin) in lysosomes.
Free radicals are rapidly destroyed by protective enzyme systems, such as superoxide dismutase. However, according to this theory, some free radicals escape destruction and cause damage that accumulates in important biological structures. This accumulation of damage eventually interferes with function and ultimately causes death. The accumulation of age pigments does not refer to the dark brown spots on one’s hands. Age pigments (lipofuscin) are seen at microscopic levels in self-selected tissues of the body, such as nerve and muscle tissue.66 Lipofuscin is the oxidation product of free radical action on polyunsaturated fatty acids. The rate of accumulation of age pigments is a good index of chronological age and is perhaps one of the few aging phenomena universally demonstrated in mammals. Age pigments as an entity are examples of degenerative change. When accumulated in tissue, they cut off oxygen and nutrient supplies to surrounding areas, causing further degeneration and eventual death of tissue.66
There is much support for free radical reactions and their implications in the aging process, as well as their probable pathologic effects as a hypothesized cancer-causing and atherosclerosis-causing agent. This is an appealing theory because it provides a mechanism for aging that does not depend on tissue-specific action but is fundamental to all aerobic tissues. Although this theory has an aspect of random damage, it is not included in the nongenetic stochastic theories because some observations about free radicals are more suggestive of the developmental genetic theory.67
Another observation is that life span is a function of body size.68 Larger animals live longer. This suggests an inverse relationship between metabolic rate and life span. Researchers have speculated that each species is capable of burning a given number of calories in its lifetime. Metabolic rate is related directly to free radical generation and inversely to life span. It is reasonable to hypothesize that the rate of free radical production is in some way related to life span determination or senescence. Proponents of this theory suggest that caloric restriction can increase the mean and maximal life span of a species. The notion is that caloric restriction lowers the metabolic rate and therefore decreases free radical production.68
Caloric Restriction Theory
Walford69 is a staunch proponent of caloric restriction, also known as energy restriction. Dr. Walford himself serves as living evidence of the in vivo experiment and that a lifestyle committed to the high-nutrient/low-calorie diet, moderate vitamin and mineral supplementation, and a regular exercise regimen is beneficial. Caloric restriction and its effects on life span extension are perhaps one of the most promising probes of the mechanisms of aging. Caloric restriction may exert its effectiveness through the neuroendocrine system.
This high-nutrient/low-calorie diet is a result of years of animal in vivo experimentation exploring longevity and maximum life span potential. Dr. Walford’s experiments have been well received and respected, and other noted theorists, such as Leonard Hayflick, have acknowledged Walford’s works.70
Walford’s caloric restriction program prescribes that an individual gradually loses weight over several years until a point of maximum metabolic efficiency is reached for maximum health and life span.71 Despite more recent reports associating being a little overweight as “healthier” than being underweight, the most recent results from the National Institutes of Health Nutrition Committee and the Centers for Disease Control and Prevention have concluded that “the weights associated with the greatest longevity are below average weights of the population,” as long as such weights are not associated with diseases.71 The result of the high-quality but low-caloric diet serves to retard aging in the sense that one would be chronologically old but functionally younger.
The calorie-restricted diet influences the aging rate and disease susceptibility.68 It has been said that the immune system is the pacemaker of aging,70 and caloric restriction has been shown to affect the immune system. For example, it slows down the immune system’s decline and inhibits the increased autoimmune reaction.68 What is the relationship of energy restriction to other aging theories? Caloric restriction influences widely diverse phenomena, particularly those whose mechanistic possibilities include effects on the immune system, cell basal state and proliferation potential, metabolic rate, DNA repair, levels of free radical scavengers, chromatin structure, and protein synthesis and turnover.72
Stochastic Theories
The second category of theories is described as stochastic theories. These theories purport that aging at the molecular level is caused by an accumulation of insults from the environment. The result of these insults is that the organism eventually reaches a level incompatible with life.73 There is limited evidence (although this is evolving via the genome studies discussed subsequently) that genetics plays a role in the aging process per se although the genomic complement regulates longevity. Aging at the molecular level can be viewed as a stochastic process resulting from increased disorderliness of intra- and intercellular regulatory mechanisms. This results in reduced robustness of the organism to stress and disease. The notion of greater disorderliness in aging is also evident at the whole organismic level, as illustrated by the erosion of the orderly neuroendocrine feedback regulation of the secretion of GH, LH, follicle-stimulating hormone, and ACTH.46
Error Theory
The error theory, also known as the error catastrophe theory, was first presented by Orgel in 1963.74 It states that, although random errors in protein synthesis may occur, the error-containing protein molecule will be turned over, and the next copy will be error free. However, if the error-containing protein is one that is involved in the synthesis of the genetic material or in the protein-synthesizing machinery, then this molecule could cause further errors. If this is the case, the number of error-containing proteins expands to result in an “error crisis” that would be incompatible with proper function and life. The theory specifies that “any accident or error in either the machinery or the process of making proteins would cascade into multiple effects.”75 A decrease in the fidelity or accuracy of protein synthesis was specifically hypothesized to be caused by errors in proper initiation of the pairing of messenger RNA codon with an anticodon of transfer RNA. However, “it may not be possible to distinguish between contributions to cellular aging caused by errors in protein synthesis from those due to accumulation of somatic mutations.”76 It is questionable whether accurate protein synthesis is distinguishable from inaccurate DNA synthesis and whether the accuracy of both processes are dependent on the fidelity of the other. The interdependence and cumulative effects of errors remain deeply intertwined and somewhat indistinguishable.75
Recent experiments contradict Orgel and no longer lend support to the error theory. In human cell cultures fed 2 amino acids (p-fluorophenylalanine and ethionine) early in their life spans, the short-term, high-dose cultures and the long-term, low-dose cultures had essentially the same replicative life spans.76 This result seems irreconcilable with the error theory. It is an appealing theory because of its apparently straightforward testability through the detection of mis-synthesized proteins. Experiments have shown that not all older cells accumulate mis-synthesized protein molecules and that aging is not necessarily accelerated when mis-synthesized protein molecules are purposely introduced.76 Despite numerous reports of altered proteins in aging,74 no direct evidence of age-dependent, malsynthesis has yet been obtained. Altered proteins do occur in aging cells and tissues; however, at present it seems that the accuracy of the protein-synthesizing mechanism does not decrease with age. Rather, the capacity of the protein removal machinery in old cells is compromised.74,75 It may follow that old cells that contain many modified copies of functionally important proteins could have impaired functional capacity. From a historical viewpoint, the error theory was important to test these hypotheses in order to move toward a more plausible theory.
Redundant DNA Theory
Another dimension of the error theory is the premise that the ability to repair damage to the genetic material is somehow associated with aging or the rate of aging.77 Medvedev78 suggested that biological age changes are a result of errors accumulating in functioning genes. As these errors accumulate, reserve genetic sequences with identical information take over until the system’s redundancy is exhausted. This theory is known as the redundant message theory. Medvedev78 writes that different species’ life spans may be a function of the degree of repeated genetic sequences. If an error occurred in a nonrepeated gene sequence, the chance of preserving a final intact gene product during evolution or a long life span would be diminished. Researchers obtained evidence that the ability to repair DNA cells after ultraviolet damage in cell cultures derived from various species of different maximum life spans was directly correlated to maximum life span potential.79
The mitochondrial theory of aging, based on the redundant DNA theory, states that the slow accumulation of impaired mitochondria is the driving force of the aging process.80 The discovery of age-related mitochondrial DNA deletions has shown that damaged mitochondria have a decreased degradation rate, resulting in an increase in the accumulation of defective mitochondria. The underlying mechanism of the accumulation of defective mitochondria remains unclear; however, this hypothesis solves inconsistencies of the current model of redundancy.80,81
Somatic Mutation Theory
One of the most prominent theories in the stochastic theories category is the somatic mutation theory of aging.82–84 This theory emerged following World War II as a result of increased research in the area of radiation biology. The theory hypothesizes that mutations or genetic damage result from radiation and that radiomimetic agents accumulate and eventually create functional failure and death of the organism. The somatic mutation theory is based on the scientific observation that exposure to ionizing radiation shortens the life span. Researchers demonstrated that exposure to radiation was recessive. It requires 2 or more “hits” from radiation to inactivate a given locus, and the exposure must occur in a sufficient number of cells for the damage to be manifested.82,84 There are several arguments against the somatic mutation theory. Life span shortening by radiation does not define whether the life span mechanism bears any relationship to the normal mechanism of aging.84 Numerous treatments that would shorten life span are not related to normal aging (eg, radiation treatment). Also, inbred animals would have a longer life span than outbred animals because inbred animals would be homologous at most genetic loci and, therefore, more resistant to random damage. In fact, the opposite is true, as exemplified in the well-known phenomenon of hybrid vigor. Some of the most compelling experiments addressing somatic mutation were done on the effects of radiation and life span comparing haploid and diploid animals.85 Although the haploid animals were much more sensitive to ionizing radiation, both haploids and diploids had the same life span when radiation was withheld. This finding is difficult to reconcile in light of the somatic mutation theory.
Cell senescence, or somatic mutation of cells, limits cell divisions in normal somatic cells and may play a central role in age-related diseases. In other words, cell senescence underlies and is a pivotal event in human aging and disease. Cell senescence is the cellular process in which normal diploid cells cease to replicate and is a major driving force for human cancers and aging-associated diseases. Recent studies on cell senescence have identified many new genetic components and pathways that control cell aging.86 However, there is no comprehensive resource for cell senescence that integrates various genetic studies and relationships with cell senescence, and the risk associated with complex diseases such as cancer is still unexplored. There are in vitro and ex vivo data to support the theory that this cell senescence leads to pathologies such as arthrosclerosis, osteoarthritis, immune senescence, skin aging, Alzheimer disease, and cancer. Such data, if confirmed, have stunning therapeutic (as well as commercial) consequences.82
Interestingly, the caloric restriction theory supports the theory of somatic mutation. A key prediction of the somatic mutation theory of aging is that there is an invariant relationship between life span and the number of random mutations. A number of studies have shown that somatic mutations of a variety of types accumulate with age and that DR prolongs life span by slowing the accumulation of mutant genes.71,72
Cross-Linkage Theory
A theory related to the redundant DNA theory is based on cross-linking in macromolecules. In 1942, Johan Bjorksten first related the concept of cross-linkage to developmental aging.86 Before the 1940s, cross-linking was used as a method to stabilize macromolecules for individual purposes, such as vulcanizing rubber. Although cross-linking is not restricted to proteins, most experimental research has been on collagen and elastin because these molecules are accessible, do not readily turn over, and show increased cross-linking with age. Bjorksten looked at large reactive protein molecules within the body, such as collagen, elastin, and DNA molecules, and surmised that their cross-linkage was responsible for secondary and tertiary causes of aging.86 According to Bjorksten87:
Cross-linking reactions result in the union of at least two large molecules. A bridge or link between these is usually formed by a cross-linking agent: a small, motile molecule or free radical with a reactive hook or some other mechanism at both ends, capable of reacting with at least 2 large molecules. It is also possible for 2 large molecules to become cross-linked by the action of their own side chains or reactive groups present on one or both of them, or pathologically formed by ionizing radiation.
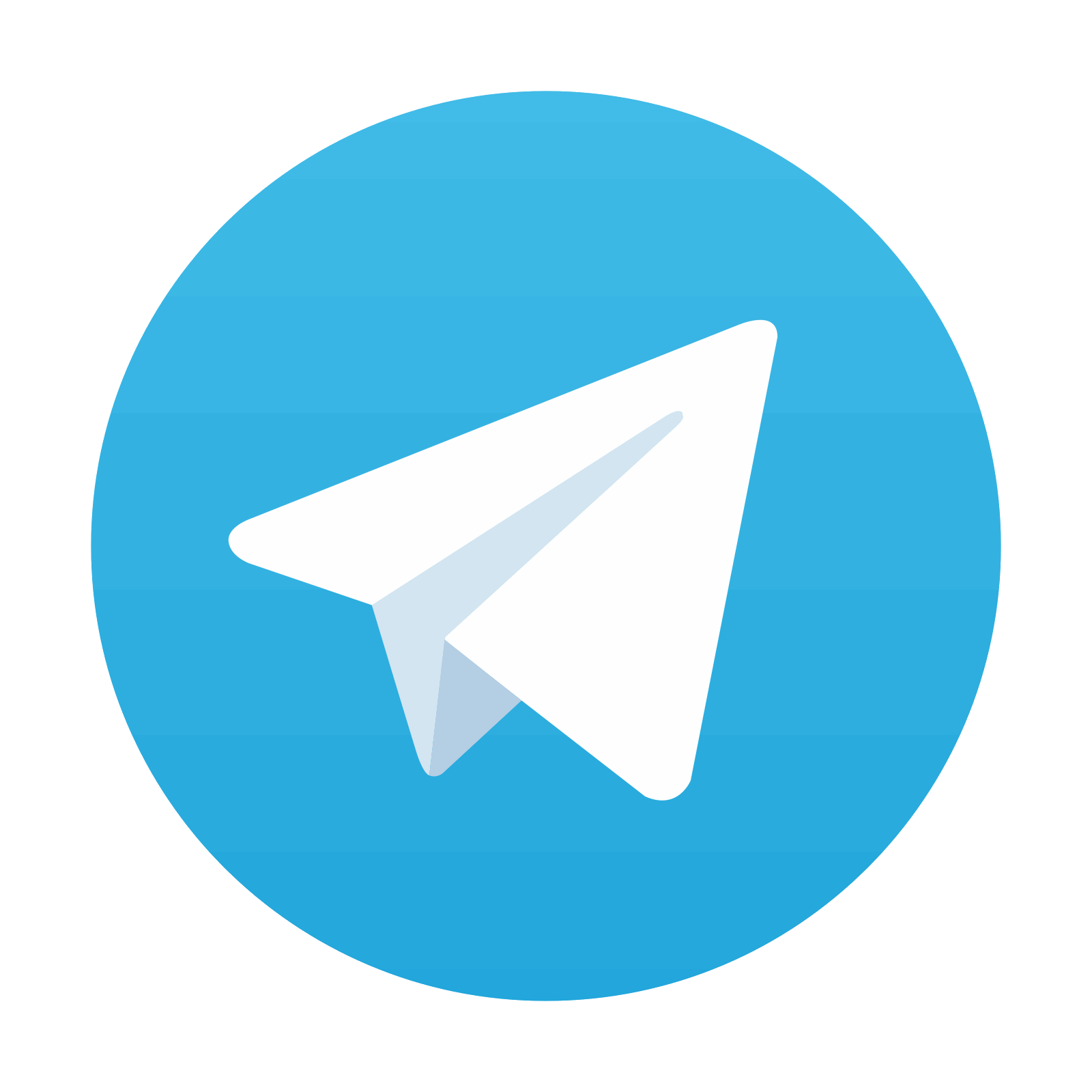
Stay updated, free articles. Join our Telegram channel

Full access? Get Clinical Tree
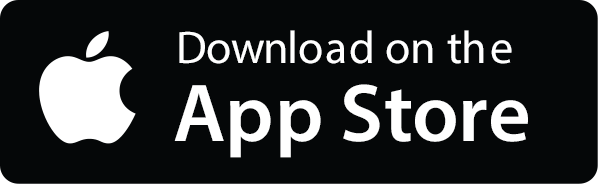
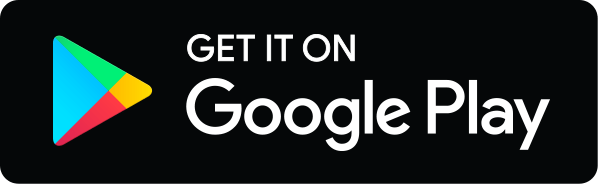