Clinical Aspects and Applications of High–Dose-Rate Brachytherapy
Brachytherapy has the advantage of delivering a high radiation dose to the tumor while sparing the surrounding normal tissues. Brachytherapy procedures were initially performed by inserting radioactive material directly into the tumor (“hot” loading), thereby giving high radiation exposure to the physicians performing the procedure. Manually afterloaded techniques were introduced to increase accuracy and reduce the radiation hazards. In afterloaded techniques, hollow needles, catheters, or applicators are first inserted into the tumor then loaded with radioactive materials. The introduction of remote-controlled insertion of sources eliminated radiation exposure to visitors and medical personnel. In this technique, the patient is housed in a shielded room and the radiation therapist controls the treatment from outside the room. Hollow applicators, needles, or catheters are inserted into the tumor and connected by transfer tubes to the radioactive material, which is stored in a shielded safe within the high–dose-rate afterloader. The radiation source is driven through the transfer tubes and into the tumor by remote control.
Remote-controlled brachytherapy can be performed using low–dose-rate (LDR), medium–dose-rate (MDR), or high–dose-rate (HDR) techniques. Although the International Commission on Radiation Units and Measurements (ICRU) definition No. 38 of HDR is >12 Gy/hour,1 the usual dose rate employed in current HDR brachytherapy units is about 100 to 300 Gy/hour. HDR has the added advantage that the treatments take only a few minutes, and therefore can be given on an outpatient basis with minimal risk of applicator movement and minimal patient discomfort. Additionally, use of a single-stepping source, as used in most modern HDR afterloaders, allows optimization of dose distribution by varying the dwell time at each dwell position. However, it should be emphasized that while optimization can improve the dose distribution, it should not be used to substitute for a poorly placed implant. Nag and Samsami2 have provided examples of inappropriate optimization strategies that can lead to suboptimal dosimetry plans and clinical problems. HDR is normally given as a course of a number of fractionated HDR treatments, although it can be given as a single treatment, as in intraoperative HDR brachytherapy, if doses to the normal tissues can be sufficiently reduced by displacement or shielding. The advantages and disadvantages of HDR in comparison to LDR are enumerated in Table 25.1.
The previously mentioned advantages have led to increased use of HDR worldwide; however, training and expertise are required for proper administration of these treatments. Guidelines and recommendations for the use of HDR at various sites have been published by the American Brachytherapy Society (ABS), much of which is summarized in this chapter,3–11 and the reader should refer to these publications for details. Controlled clinical trials are needed to critically evaluate the efficacy of these procedures.
TABLE 25.1 ADVANTAGES AND DISADVANTAGES OF HIGH–DOSE-RATE COMPARED WITH LOW–DOSE-RATE BRACHYTHERAPY
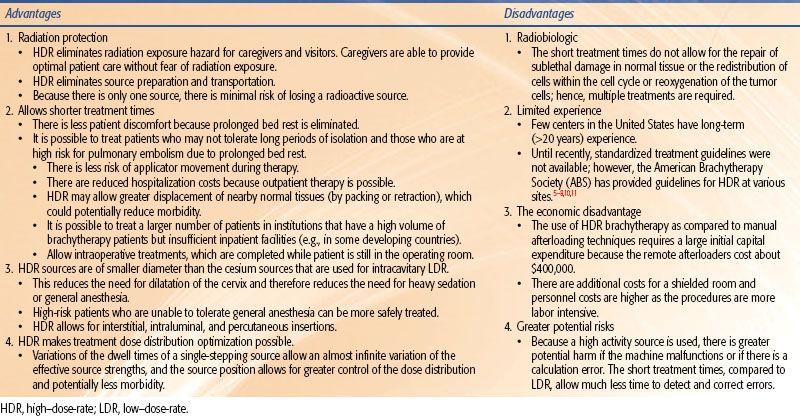
RADIOBIOLOGIC PRINCIPLES OF HIGH–DOSE-RATE BRACHYTHERAPY
Most radiation oncologists are familiar with LDR brachytherapy. LDR (at 30 to 50 cGy/hour) can be added to external-beam radiation therapy (EBRT) doses (at 2 Gy/day) to obtain equivalent total doses. HDR brachytherapy is distinct from LDR brachytherapy, and radiation oncologists who are accustomed to LDR techniques must realize that experience in LDR cannot be automatically translated into expertise in HDR. It is important to review the current literature and survey the experiences of centers that have been performing HDR. When converting from LDR to HDR, one must keep the other parameters (chemotherapy, EBRT field/dose, dose specification point, applicators, patient population, and so forth) the same, changing only the LDR to HDR.
Fractionation schemes for HDR are widely variable, and many radiation oncologists are not very familiar with the resultant biologic effects. Empirical methods such as the NSD (nominal standard dose), TDF (time-dose factor), or a dose reduction factor of 0.6 have been used in the past to convert HDR doses to LDR equivalent doses. The linear-quadratic (LQ) equation can be used to guide development of HDR doses and fractionation schedules.12 However, the LQ mathematical calculations are tedious and may not be practical on a day-to-day basis. Hence, a simplified computer program was developed by Nag and Gupta13 to obtain the isoeffective doses to be used for HDR. The clinician needs only to enter the EBRT total dose and dose/fraction, HDR dose, and the number of HDR fractions. The computer program will automatically calculate the isoeffective doses for tumor and normal tissue effects. Isoeffective doses are expressed in clinically familiar terms, as if given at 2 Gy per fraction (EQD2), rather than as biologically equivalent doses (BEDs), which are unfamiliar to clinicians. Furthermore, a dose-modifying factor (DMF) is applied to the normal tissues to account for the fact that doses to normal tissues are different from the doses to the tumor, thus providing a more realistic equivalent normal tissue effect. This program can be used to determine HDR doses that are equivalent to LDR brachytherapy doses (EQD2) used to treat various cancers. Alternatively, the program may be used to express the isoeffective dose of different HDR dose fractionation regimens. Although the LQ biomathematic model can be helpful in determining isoeffective doses, it has many limitations that must be kept in mind when using the program. The LQ model accounts for the repair of sublethal damage, but it does not account for reoxygenation of hypoxic cells, reassortment within the cell cycle, or repopulation of tumor cells. These factors are generally small under normal circumstances. However, large doses per fraction do not allow reoxygenation of hypoxic tumor cells or reassortment of tumors from radioresistant S phase. Hence, a large radiation dose will preferentially kill radiosensitive cells, leaving a high number of hypoxic, radioresistant cells. Therefore, the computer program will overestimate the tumor effect of a single large dose per fraction (unless a resensitization factor is introduced).
The LQ equation does not take into account the proliferation of tumor cells. This factor is small if the treatments are performed over a short duration. However, if the treatments are highly protracted (e.g., there is a long time interval between EBRT and HDR), or in cases of tumors with high proliferation rates, the LQ model will overestimate the actual tumor effect. It also must be noted that individual α/β values are very variable. The α/β values for early reactions vary from 6 to 13 (the default in the program is set at 10); the α/β values for late reactions vary from 1 to 7 (the default being set at 3), while α/β values for tumors vary from 0.4 to 13 (the default being set at 10). However, α/β values for a particular patient are not known and may vary even within the same tissue. The isoeffective doses obtained will therefore depend on the α/β values used for that particular calculation. The LQ model assumes complete repair between fractions. If the time interval between fractions is too short (<6 hours) or the half-time of repair is very long, the repair of normal tissues will be incomplete, and the LQ formula will underestimate the biologic effect. Hence, it is important to have a sufficient time interval (at least 6 hours) between treatment fractions.
The infinite variation of the dwell times that is possible with HDR (or pulsed-dose rate) allows better optimization of the doses than can be achieved with LDR. Better packing or retraction of normal tissues is possible with HDR, due to the short treatment duration. This factor is not usually taken into account in the LQ model (unless the DMF is altered). Another difference not accounted for in the LQ model is that the dose stated in brachytherapy is generally the minimum tumor dose. The doses within the tumor are much higher. Hence, the effective dose (for tumor control probability) is much higher for brachytherapy than for EBRT.
In view of the many limitations of the LQ model, it must be stressed that, as with any mathematical model, the LQ model should be used judiciously only as a guide and should always be correlated with clinical judgment and outcome results. Due to the reasons mentioned earlier, caution is especially warranted whenever large fraction sizes, a short time interval between fractions, or highly protracted treatments are used.
TABLE 25.2 GEC-ESTRO IMAGE-BASED PLANNING VOLUME DEFINITIONS FOR CERVICAL CANCER
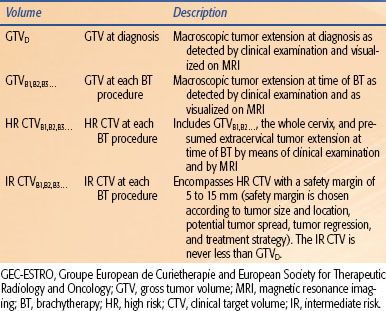
Common Uses of High–Dose-Rate Brachytherapy
Although HDR brachytherapy has been used in almost every site in the body, it is now most commonly used to treat cancers of the cervix, endometrium, prostate, and breast. Less commonly treated sites for HDR include the lung, esophagus, bile duct, rectum, head and neck, skin, soft tissues, and blood vessels (coronary and peripheral arteries). HDR is generally used as a component of multimodality treatment that includes EBRT and/or chemotherapy and surgery. A summary of the clinical uses of HDR is included in this chapter, while the details of the physics and radiobiology of HDR are provided in other chapters.
Carcinoma of the Cervix
Brachytherapy is a necessary component in the curative treatment of cervical cancers.8 HDR brachytherapy has been utilized more frequently compared to LDR brachytherapy over the past two decades due to the ability to deliver therapy on an outpatient basis, avoidance of long-term bed rest, and avoidance of cervical dilation. Additionally, greater sparing of the rectum and bladder by temporary retraction, dose optimization, and integration with EBRT to the pelvis is possible.14 These advantages must be counterbalanced with the greater number of treatments required (typically four to six treatments, lasting approximately 10 to 15 minutes each). A recent international survey revealed that 85% of centers now utilize HDR brachytherapy.15
The ABS recommends keeping the total duration of treatment (EBRT and HDR) to <8 weeks because prolongation adversely affects local control and survival.8 To maintain this treatment duration, the HDR is interdigitated during the course of EBRT. However, it should be noted that neither EBRT nor chemotherapy is given on the day of an HDR treatment. Typically, if the tumor is small and the vaginal geometry is optimal, HDR brachytherapy begins approximately 2 weeks after starting EBRT. HDR is then continued one time per week with the EBRT given on the other 4 days of the week. If large tumor volume requires delaying the start of HDR brachytherapy, it may be necessary to perform two implants per week after the EBRT has been completed to keep the total treatment duration to <8 weeks.
The insertion of the applicator is usually performed under intravenous sedation. However, if the cervix needs to be dilated, general or spinal anesthesia will likely be required. It should be emphasized that rectal and bladder retraction (by the use of packing or retractors) is essential in HDR as it is for LDR. The use of an external immobilization device (EID) to fix the position of the applicator is controversial; some centers prefer to use an EID to fix the position of the applicators, while others feel that the use of the EID is detrimental. Various types of applicators (tandem and ovoid being the most common) have been used and depend on the preference of the radiation oncologist.
Treatment Planning
Traditionally the HDR dose was prescribed to an arbitrary applicator-based definition of point A.4,8 Unfortunately, point A does not necessarily reflect the dose to the tumor. Given this fact and in conjunction with the proliferation of improved imaging modalities, there has been a shift toward image-based treatment planning in brachytherapy for carcinoma of the cervix. It is well established that magnetic resonance imaging (MRI) is superior to other current imaging modalities in delineating gross tumor involving the cervix and adjacent normal tissues.16 The use of MRI can lead to better delineation of the target volume, which then could translate to dose escalation for better tumor control while simultaneously limiting dose to normal tissues.
A gynecologic working group was formed by the Groupe European de Curietherapie and European Society for Therapeutic Radiology and Oncology (GEC-ESTRO) in 2000 to formulate and describe new terminology regarding three-dimensional (3D) image-based treatment planning in cervical cancer brachytherapy. Their recommendations are summarized in Table 25.2.17 This working group recognized that most patients with cervical cancer are treated with combined-modality therapy including external radiotherapy, chemotherapy, and brachytherapy and that there is significant change of gross tumor during such treatment. Because of this, a fluid description of treatment volumes during the course of treatment is required to have a better understanding of dose-to-volume relationships throughout treatment and to more accurately compare treatments between institutions and patients. They devised definitions of gross tumor volume (GTV) at diagnosis and at each brachytherapy procedure as well as high-risk and intermediate-risk clinical target volumes (CTVs). Each of these volumes would be determined via clinical examination and MRI (preferably T2 weighted) at their respective times during the course of treatment. The CTVs are based on tumor load and represent risk of recurrence. Thus, “high risk” (HR-CTV) is that which includes macroscopic tumor, “intermediate risk” (IR-CTV) is that which includes significant microscopic disease, and “low risk” (LR-CTV) is that which includes potential microscopic tumor spread. It is assumed that the low-risk region is successfully treated with surgery and/or external-beam radiotherapy. Their recommendations were expanded in 2006 to include descriptions of dose–volume parameters for organs at risk and a stepwise procedure for transition from the traditional dose prescription (i.e., point A) to the 3D image-based volume prescription.18 The group recommended for organs at risk that the minimum dose in the most irradiated tissue volumes of 0.1 cm3, 1 cm3, and 2 cm3 be reported.
The recommended combined EBRT and HDR dose to at least 90% (D90) of the HR-CTV is an isoeffective dose of 75 to 90 Gy.17,18 The lower dose range can be utilized for early-stage disease (defined as nonbulky stage I or II <4 cm in diameter) and the higher dose range is used for advanced stage disease (defined as stage I or IIA >4 cm in diameter or stage IIIB). The total pelvic sidewall dose recommendations are 50 to 55 Gy for smaller lesions and 55 to 60 Gy for larger ones. The ratio of EBRT to brachytherapy is dependent on the stage, with a larger EBRT dose used for the more advanced stages. Most centers use a schedule of about 6 to 8 Gy per fraction in four to six fractions (a smaller number of fractions is used by those using larger doses per fraction).8,14,19–24 The HDR dose is also dependent on the stage of the disease and the dose of pelvic EBRT.
The University of Vienna has extensive experience with image-based dosimetry for intracavitary brachytherapy for cervical cancer. Their evaluations have resulted in suggestions to keep the D2 mL dose to the rectum <75 Gy and the D2 mL to the bladder to <100 Gy.25,26 The optimal dose constraint for the sigmoid is less clear, but applying the same constraint as used for the rectum is reasonable. As an example, in a patient who has received 45 Gy of EBRT and is planned to undergo five intracavitary brachytherapy treatments, the fractional HDR D2 mL dose to the rectum and bladder should be kept to approximately <4.2 Gy and <6.1 Gy, respectively. These doses would need to be altered based on the total EBRT dose and number of planned intracavitary brachytherapy treatments.
In certain clinical situations (e.g., a narrow fibrotic vagina, bulky tumors, the inability to enter the cervical os, extension to the lateral parametria or pelvic sidewall, lower vaginal extension, and suboptimal applicator placement), the normal tissue tolerance may be exceeded if the aforementioned doses are used. In these situations, either repacking or reoptimization may be attempted. If this fails to reduce the normal tissue doses, the HDR fraction size can be decreased (which requires an increase in the fraction number), or the EBRT dose increased while decreasing the HDR total dose. At our centers, we prefer to use an interstitial implant (either LDR or HDR) in these situations. In the event of reduced vaginal capacity and if an interstitial technique is not available, Sharma et al.27 have described an intracavitary technique that utilizes a central tandem with a single ovoid alternating with the contralateral ovoid with subsequent insertions.
Results of 3D Image-Based Treatment Planning
The ABS distributed a survey to its members in 2007 focusing on the utilization of 3D treatment planning for intracavitary brachytherapy of cervical cancer that was subsequently published in 2010.28 Fifty-five percent of respondents utilized computed tomography (CT), while 43% used plain film and only 2% used MRI for treatment-planning purposes. Point A was still utilized as the primary prescription point in just over 75% of respondents, while 14% used a 3D clinical target volume only. A survey distributed to the international community and published in 2011 showed similar results.15
Over the last few years there has been accumulating clinical evidence supporting image-guided brachytherapy for cervical cancer. Potter et al.29 reported their results of 145 patients with stages IB to IVA cervical cancer treated with EBRT and four image-guided brachytherapy treatments with or without cisplatin chemotherapy. MRI was used at each brachytherapy treatment and target volumes and organs at risk were contoured as per the GEC-ESTRO guidelines. Three-year local control and overall survival was 85% and 58%, respectively, with an overall mean HR-CTV D90 of 86 Gy. They further divided their experience into an early period (1998–2000) and late period (2001–2003) to better reflect the treatment-planning changes employed with the release of the GEC-ESTRO guidelines. At 3 years, complete remission was 96% for tumors 2 to 5 cm in both time periods but increased from 71% to 90% for tumors >5 cm between the two time periods. Additionally, overall survival for patients with tumors >5 cm increased from 28% to 58% between the two time periods. Grade 3 and 4 gastrointestinal and genitourinary complications decreased from 10% to 2% between the two time periods. Dimopoulos et al.30 performed a further analysis of 141 of the original 145 patients and found that an HR-CTV D90 >87 Gy resulted in a local recurrence rate of 4% versus 20% if the D90 was <87 Gy.
Additional studies have compared 3D image-guided brachytherapy to previous cohorts treated with traditional two-dimensional (2D) planning and have shown improvements in local control31,32 and reductions in toxicity31,33 with 3D planning. Appropriate concerns to the feasibility of MRI-based treatment planning for brachytherapy include the increased cost of obtaining serial MRIs during a course of treatment (on average at least four to six during a normal course of treatment) and the limited availability and high cost of MRI-compatible applicator instrumentation for brachytherapy procedures. While early results are encouraging, further studies are needed to show that the increase in cost will be offset by improvements in local control and quality of life for patients with cervical carcinoma.
Results of HDR Versus LDR Brachytherapy
LDR brachytherapy has been used with good results in carcinoma of the cervix for almost 100 years. Hence, it is important to critically analyze how the results obtained with HDR brachytherapy, which has a much shorter history, compare with those obtained with LDR. Unfortunately, most of the published reports have been nonrandomized studies. The earlier studies showed good local control and survival rates that compared well to those obtained with LDR; however, initially some increased late complications were noted. Subsequent studies using improved techniques demonstrated good control comparable to LDR without increased morbidity.20,21,23,24,34–35,37,38 Table 25.3 summarizes the HDR fractionation and results of the most recently published retrospective literature.
Meta-analyses and published literature reviews have compared HDR with LDR brachytherapy. Orton et al.14 published the classic meta-analysis comparing HDR and LDR obtained from a survey of 56 institutions treating 17,068 patients with HDR and 5,666 patients with LDR. Five-year survival data were available for 6,939 HDR patients and 3,365 LDR patients and was 82.7% versus 82.4% for stage I, 66.6% versus 66.8% for stage II, and 47.2% versus 42.6% for stage III, respectively. In 1999, Petereit and Peracey22 published a literature review that included 5,619 patients treated with HDR brachytherapy and demonstrated 5-year pelvic control rates of 91%, 82%, and 71% in stage I, II, and III patients, respectively. Five-year overall survival by stage and complication rates was similar to previous meta-analyses. Statistically quantifiable conclusions cannot be drawn from comparisons of the results of uncontrolled studies using different methodologies from various centers; however, this anecdotal evidence suggests that the results of HDR are at least as good as those achieved by LDR brachytherapy. Table 25.4 summarizes the HDR fractionation and results by stage of the meta-analyses and review literature.
Four randomized studies have compared the use of HDR with the use of LDR brachytherapy in carcinoma of the cervix.39–42,43 Shigematsu et al.42 reported on 143 patients treated with HDR brachytherapy compared to 106 patients treated with LDR for stage IIB and III disease. Although the randomization technique was suboptimal (i.e., some patients who were randomized to LDR were actually treated with HDR because of limited LDR availability), the HDR arm achieved superior local control with no difference in survival as compared to the LDR arm. In a randomized study of LDR versus HDR brachytherapy reported from India, there was no difference in local control, survival, or severe complications between the two treatment modalities in 482 patients.41 However, there was a statistical difference in rectal complications, with a 20% rate in the LDR group and only a 6% rate in the HDR group. In 2002, Hareyama et al.39 reported their results of 132 patients with stage II and IIIB cervical carcinoma. The 5-year disease-specific survival of LDR versus HDR for stage II was 87% versus 69%, respectively, and for stage IIIB 60% versus 51%, respectively. The difference between survival rates was not statistically significant. The most recent randomized study was reported from Thailand in 2004.40 In this trial, 237 patients were evaluated and there was no difference in overall survival, relapse-free survival, or pelvic control between LDR and HDR brachytherapy. In addition, there was no statistical difference in complication rates for the rectum, bladder, or small bowel. A recent meta-analysis of these randomized studies demonstrated that there was no significant difference between HDR and LDR brachytherapy regarding overall survival, local control, and treatment-related complications.44 Tables 25.5 and 25.6 summarize the results and complications, respectively, of the available randomized studies.
In summary, the available data from randomized trials, retrospective analyses, and meta-analyses suggest that survival and local control of HDR treatments are probably equivalent to that of LDR, with similar or lower morbidity.
TABLE 25.3 SUMMARY OF RETROSPECTIVE ANALYSIS OF HIGH–DOSE-RATE BRACHYTHERAPY IN THE TREATMENT OF CERVICAL CANCER
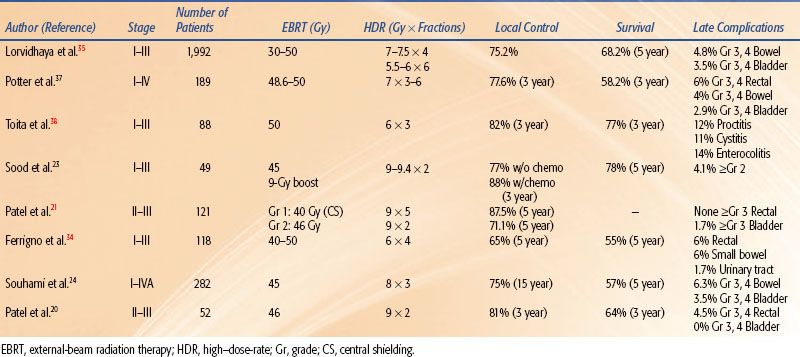
TABLE 25.4 SUMMARY OF META-ANALYSIS AND REVIEW LITERATURE OF HIGH–DOSE-RATE VS. LOW–DOSE-RATE BRACHYTHERAPY IN CERVICAL CANCER
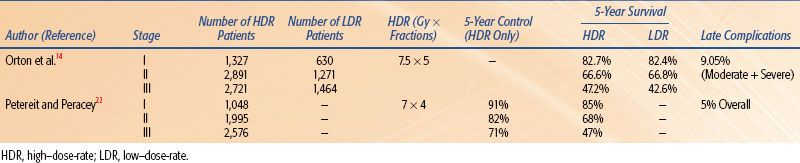
CARCINOMA OF THE ENDOMETRIUM
HDR brachytherapy is commonly used for adjuvant treatment of the vaginal cuff after hysterectomy in patients with an intermediate or high risk for vaginal recurrence (high-grade, deep myometrial invasion or advanced stage). Additionally, brachytherapy may be used for primary treatment in inoperable endometrial carcinoma and for treatment of recurrences after hysterectomy.
Vaginal Cuff Irradiation
The standard management for operable carcinoma of the endometrium is total abdominal hysterectomy with bilateral salpingo-oophorectomy (TAH-BSO). Patients at high risk for vaginal recurrences (deep myometrial invasion, high histologic grade and stage, lymphovascular invasion, cervical or extrauterine spread, squamous cell or papillary histology) should receive radiation therapy. Two previous published randomized trials, Post Operative Radiation Therapy in Endometrial Carcinoma (PORTEC-1)45,46 and Gynecologic Oncology Group (GOG)-99,47 demonstrated general benefit of postoperative pelvic radiation therapy compared to observation in regards to local control with early-stage endometrial carcinoma and intermediate- to high-risk features for recurrence. Neither trial demonstrated improvement in survival. Following these two studies PORTEC-248 was undertaken to better answer how best to deliver adjuvant radiotherapy. As the majority of the recurrences in PORTEC-1 and GOG-99 occurred at the vaginal cuff in the observation arms, PORTEC-2 compared whole-pelvic radiation therapy to vaginal cuff brachytherapy. Eligible patients for PORTEC-2 included patients 60 years or older with inner half myometrial invasion and histologic grade 3, or outer half myometrial invasion and histologic grade 1 or 2. Those with cervical glandular involvement and grade 1 or 2 histology, or cervical glandular involvement and grade 3 histology, and <50% myometrial invasion of any age were also eligible. The results of PORTEC-2 were published in 2010 and demonstrated that vaginal cuff recurrence was equal (1.8% vs. 1.6% at 5 years) in both arms. Additionally, it demonstrated that there were fewer acute grade 1 and 2 gastrointestinal toxicities with vaginal cuff brachytherapy (12.6% vs. 53.8%). This has led to an increase in the utilization of vaginal cuff brachytherapy alone in patients with a high intermediate risk of recurrence. There still remains some controversy of which patients fall into the high intermediate-risk group as the three mentioned studies had subtle differences in patient eligibility. Thus, clinical judgement is needed when evaluating patients potentially eligible for vaginal cuff brachytherapy alone.
TABLE 25.5 SUMMARY OF RESULTS OF RANDOMIZED TRIALS OF HIGH–DOSE-RATE VS. LOW–DOSE-RATE BRACHYTHERAPY IN CERVICAL CANCER
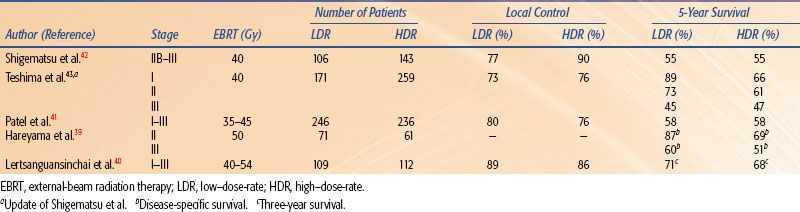
TABLE 25.6 SUMMARY OF COMPLICATIONS OF RANDOMIZED TRIALS OF HIGH–DOSE-RATE VS. LOW–DOSE-RATE BRACHYTHERAPY IN CERVICAL CANCER
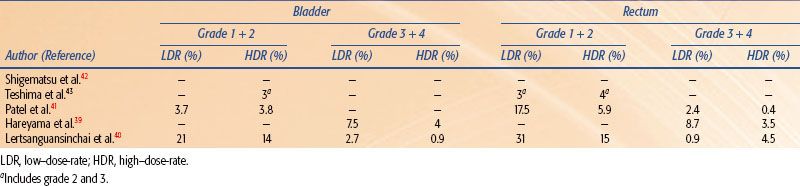
Technique
A vaginal cylinder is commonly used to deliver HDR brachytherapy. The largest-diameter cylinder that comfortably fits the vagina should be used to increase the depth dose. The length of vaginal vault treated varies. Some treat the superior 3 or 5 cm, while others treat the superior half or two-thirds of the vagina.5,48,49–51 For serous and clear cell histologies, treatment of the entire vaginal canal should be considered. The use of a single-line iridium 192 (192Ir) source creates a dose inhomogeneity at the vaginal apex due to source anisotropy. However, the clinical significance of source anisotropy is debatable. The use of ovoids, circular rings, or an angled source may reduce the dose inhomogeneity at the apex created by the 192Ir source anisotropy.5 The applicator should be placed in the midline, as horizontal as possible and parallel to the longitudinal axis of the body for appropriate dose distribution. Placement of a radio-opaque seed or clip at the vaginal apex helps to verify that the applicator is in contact with the vaginal apex on fluoroscopy or radiographs. However, these clips or seeds can sometimes fall off or migrate deep to the mucosa and, therefore, may not always indicate the position of the apex. Some centers prefer to use an external immobilization device to minimize movement; however, this is not mandatory. The use of a multichannel vaginal applicator such as the Miami applicator or the inflatable Capri balloon applicator may allow the radiation oncologist to better sculpt the desired radiation dose distribution for the individual patient compared to a single-channel device.
The dose distribution should be optimized to deliver the prescribed dose either at the vaginal surface or at a 0.5-cm depth, depending on the institutional policy. It is important to place dose optimization points not only along the lateral aspect of the vaginal wall but also at specified points about the dome of the vaginal cylinder to avoid higher vaginal apex doses, which can lead to vaginal vault necrosis.2 Regardless of the prescription method, doses at both the vaginal surface and the 0.5-cm depth should be reported.5
The dose per fraction used has varied from 4.5 to 16 Gy, and the number of fractions has varied from two to seven, with the interval between fractions of 1 to 2 weeks.49–53 The lower doses per fraction are usually given using either a larger number of fractions or in combination with EBRT to the pelvis. Sorbe et al.54 evaluated two fractionation schemes (2.5 Gy × 6 vs. 5 Gy × 6) in a randomized trial and found no difference in locoregional recurrence rates but an increase in vaginal shortening, mucosal atrophy, and bleeding in the 5-Gy-per-fraction arm. The ABS dose suggestions5 for HDR alone or in combination with 45 Gy EBRT are given in Table 25.7. Because some institutions specify the dose to the vaginal surface and others specify the dose at 0.5-cm depth, suggested HDR doses have been given for both specification methods.
From retrospective analysis, the 5-year survival rates of HDR therapy vary from 72% to 97%, depending on the stage, grade, and depth of myometrial invasion.49–53,55,56 The severe (grade III or IV) late complication rate is usually <2% and depends on the dose per fraction.49,50,52,57,58 The incidence of vaginal shortening is also very much dose dependent, reportedly ranging from as high as 70% when 9 Gy per fraction was prescribed at 1-cm depth to 31% when the dose was reduced to 4.5 Gy per fraction.59 Other factors that increase morbidity include the use of a small (2-cm) diameter vaginal cylinder, the addition of pelvic external-beam radiation, and a dose specification point beyond 0.5 cm.60
Petereit and Peracey22 published a literature review analyzing 1,800 cases of postoperative HDR brachytherapy alone in patients with low- to intermediate-risk endometrial cancer. They found an overall vaginal control rate of 99.3%. Late morbidity was significantly higher with isoeffective doses for late-responding tissues exceeding 100 Gy. Patient education regarding the use of frequent vaginal dilation following HDR brachytherapy is important to minimize vaginal stenosis and sexual dysfunction.61,62 The National Forum of Gynecological Oncology Nurses (NFGON) has published best practice guidelines for the use of vaginal dilators following radiation therapy treatments.63
Treatment of Recurrences at the Vaginal Cuff
A combination of pelvic EBRT and brachytherapy is generally used to treat recurrences at the vaginal cuff. With distal vaginal recurrences, the entire vagina and medial inguinal nodes are included in the EBRT field. Intracavitary vaginal brachytherapy should be used only for nonbulky recurrences (thickness <5 mm after the completion of EBRT).5 Interstitial brachytherapy is to be used for bulky recurrences (thickness >5 mm after the completion of EBRT) and for previously irradiated patients. Apical recurrences are often more extensive superiorly than can be judged on physical examination, thus favoring the use of interstitial brachytherapy. These patients are best treated at centers with considerable experience in interstitial brachytherapy. Radio-opaque marker seeds or surgical clips should be placed at the margins of gross disease to delineate disease extent. If the relapse is limited to one wall of the vagina, consideration should be given to limiting the dose to the opposite wall. The ABS-suggested doses for HDR brachytherapy (in combination with 45 Gy EBRT) are provided in Table 25.8.5
Inoperable Endometrial Carcinoma
Patients with adenocarcinoma of the endometrium who are not candidates for surgery because of severe medical problems are treated with radiation therapy. A combination of pelvic EBRT and brachytherapy is preferred whenever possible. However, many of the conditions that do not allow surgery in these cases are also relative contraindications for EBRT and for LDR brachytherapy. In such cases, these patients may be treated with HDR alone.
Numerous applicators can be used for treatment of primary endometrial cancer. The tandem and ovoid applicator, while often used, does not irradiate the uterine fundus homogeneously. Others have therefore used a curved tandem, turning it to the left and right in alternate insertions. A Y-shaped applicator irradiates the fundus more evenly. Other possibilities include modified Heyman capsules or multiple tandems. The dose is commonly specified at 2 cm from the source, although CT- or MRI-based treatment planning to ensure a more homogeneous dose to the entire myometrium is preferred. The dose per fraction has ranged from 5 to 12 Gy, and three to six fractions are commonly employed.64,65–68 The dose and/or the dose per fraction are reduced if EBRT can be added. The ABS-suggested doses for HDR brachytherapy alone or in combination with 45 Gy EBRT are given in Table 25.9.5 The survival at 5 years for stage I is about 70% to 80%, which is slightly lower than that obtained by surgery. Coon et al.69 reported a 5-year cause-specific survival of 87% for patients treated with 35 Gy HDR (treated twice a day) in five fractions without EBRT or with 20 Gy HDR in five fractions along with EBRT. The toxicity is higher (about 7%) when patients are treated with high doses per fraction.59
TABLE 25.7 AMERICAN BRACHYTHERAPY SOCIETY–SUGGESTED DOSES OF HIGH–DOSE-RATE BRACHYTHERAPY ALONE OR IN COMBINATION WITH PELVIC EXTERNAL-BEAM RADIATION THERAPY TO BE USED FOR ADJUVANT TREATMENT OF POSTOPERATIVE ENDOMETRIAL CANCER
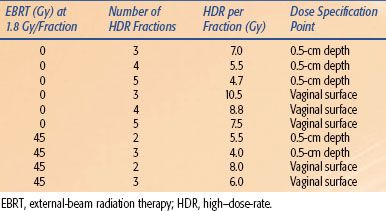
TABLE 25.8 AMERICAN BRACHYTHERAPY SOCIETY–SUGGESTED DOSES OF HIGH–DOSE-RATE BRACHYTHERAPY TO BE USED IN COMBINATION WITH PELVIC EXTERNAL-BEAM RADIATION THERAPY FOR TREATING VAGINAL CUFF RECURRENCES FROM ENDOMETRIAL CANCER
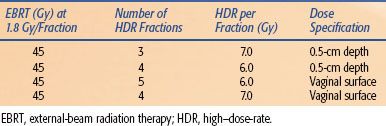
TABLE 25.9 AMERICAN BRACHYTHERAPY SOCIETY–SUGGESTED DOSES OF HIGH–DOSE-RATE BRACHYTHERAPY ALONE OR IN COMBINATION WITH EXTERNAL-BEAM RADIATION THERAPY FOR TREATMENT OF INOPERABLE PRIMARY ENDOMETRIAL CANCER
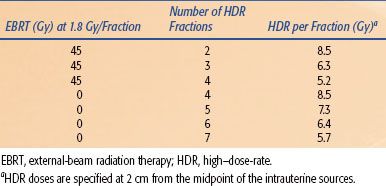
Carcinoma of the Prostate
Currently, permanent implantation of iodine 125 (125I) or palladium 103 (103Pd) seeds is the most common type of prostate brachytherapy. However, several centers have used HDR brachytherapy as a boost to EBRT70–85 for the treatment of prostate cancer with encouraging results (Table 25.10). Galalae et al.76 reported the results of 611 patients (some of which are included in Table 25.10) treated at three institutions (Kiel, Germany; William Beaumont Hospital; Seattle Prostate Institute) with EBRT and HDR brachytherapy for localized prostate cancer. Different fractionation schemes were used as seen in Table 25.11. Five-year biochemical control for low-risk, intermediate-risk, and high-risk patients was 96%, 88%, and 69%, respectively. One of the major advantages of HDR is that the dose distribution can be intraoperatively optimized by varying the dwell times at various dwell positions, potentially allowing reliable and reproducible delivery of the prescribed dose to the target volume while keeping the doses to normal structures (i.e., rectum, bladder, and urethra) within acceptable limits.86 Another potential advantage of HDR brachytherapy in prostate cancer is the theoretical consideration that prostate cancer cells behave more like late-reacting tissue with a low α/β ratio and they should, therefore, respond more favorably to higher-dose fractions rather than to the lower-dose rate delivered in LDR brachytherapy.87,88,89
Patients with stages T1b to T3b prostate cancers without evidence of distant metastases are candidates for HDR brachytherapy as a boost to EBRT. Patients with distant metastases, with a life expectancy of <5 years, or who are medically unfit for anesthesia or in whom it is technically not feasible to implant the entire prostate should be excluded. Relative contraindications include large gland size (>60 mL), significant urinary obstructive symptoms, recent transurethral resection of the prostate (TURP) within the last 6 months, large TURP defects, infiltration of the external sphincter of the bladder neck, pubic arch interference, and a rectum–prostate distance on transrectal ultrasound of <5 mm.86 These patients have, however, been implanted by experienced brachytherapists by using modified techniques.
Various implant techniques and treatment-planning methods have been used. As in permanent seed implantation, the procedure is performed under general or spinal anesthesia. Transrectal ultrasound is utilized for catheter placement and cystoscopy may be performed to exclude the urethra and bladder. At some institutions real-time transrectal ultrasound-guided treatment planning is performed. Other institutions use CT-based treatment planning. Fiducial markers are placed in the prostate so that radiographic comparison of the markers to catheter location can be confirmed prior to each delivery of each treatment.90 Typically, 15 to 17 catheters are placed (although some institutions have used fewer) with fraction doses ranging from 3 to 15 Gy prescribed to the prostate (CTV 1) depending on the clinical situation. Additionally, it is recommended to identify the peripheral zone (CTV 2) and any areas of macroscopic tumor (CTV 3) so that these areas can potentially receive a higher fractional dose. As in permanent seed implantation, the D90, D100, V100, V150, and V200 should be reported. Simultaneously, the organs at risk should be contoured and the D32cm of the rectum and bladder and D30.1cm of the urethra should be reported.86 The interested reader should review the GEC/ESTRO guidelines for more specific information.86
Standard fractionation EBRT of 39.6 to 50.4 Gy or hypofractionated EBRT of 40 Gy in 16 fractions is given before, concurrently with, or after HDR brachytherapy. The minimum volume treated should include the entire prostate and seminal vesicles with a margin, with or without pelvic lymph nodes. The HDR dose is given in multiple fractions in one or two implant procedures. A variety of dose and fractionation schemes may be appropriate for same-stage disease as shown in Table 25.11.70,72,73,75,77,79–80,81 The HDR fractions are generally given twice a day with a minimum of 6 hours between fractions. The most commonly encountered acute genitourinary (GU) morbidities include urinary irritative symptoms, hematuria, hematospermia, and/or urinary retention, similar to LDR permanent implants.
HDR brachytherapy is also being used as monotherapy in a few centers, but long-term results are still forthcoming (Table 25.12).90,91–92,93 HDR doses of 38 Gy delivered in four fractions (two times daily over 2 days), 54 Gy in nine fractions given twice a day over 5 days, and 42 Gy in six fractions over two separate implants have all been reported.90,91,93 Demanes et al.90 reported the combined results of 298 patients with low- to intermediate-risk prostate cancer treated at California Endocurietherapy (CET) or William Beaumont Hospital (WBH) between 1996 and 2005. HDR brachytherapy was delivered in either four fractions to a total dose of 38 Gy over 2 days in one implant (WBH) or a total dose of 42 Gy over six fractions with two implants 1 week apart (CET). With a median follow-up of 5.2 years, the 8-year biochemical control was 97%. Grade 3 genitourinary toxicity was 3% (urinary retention), while gastrointestinal toxicity was <1%. These results compare favorably to traditional permanent seed implant.90
Treatment of recurrent prostate cancer as well as treatment of de novo prostate cancer in patients who have previously received pelvic radiation is challenging. HDR brachytherapy has been used as salvage therapy after prostatectomy, EBRT, and permanent seed implant.94–97 Overall patient numbers are low, so firm conclusions of its effectiveness are difficult; however, it does appear feasible in various clinical situations. Niehoff et al.96 have reported their results of using HDR brachytherapy along with EBRT as salvage treatment for local recurrences after radical prostatectomy. Thirty-five patients were treated with either 30 or 40 Gy EBRT plus an HDR brachytherapy dose of 15 Gy in two fractions. Thirty-four patients had a decrease in prostate-specific antigen (PSA), and at a mean follow-up of 27 months 91% were alive. Mean duration of biochemical nonevidence of disease was 12 months, and there were no reported Radiation Therapy Oncology Group (RTOG) grade III or IV side effects.
HDR brachytherapy as a boost to EBRT for localized prostate cancer is being used more commonly and its role has been well defined. Its use as monotherapy is increasing and the early results are promising. Further studies are needed to more clearly define the role of HDR brachytherapy for recurrent disease after radical prostatectomy, previous EBRT, or permanent seed implant.
TABLE 25.10 RESULTS OF TREATMENT WITH INTERSTITIAL HIGH–DOSE-RATE BRACHYTHERAPY AS A BOOST TO EXTERNAL-BEAM RADIATION THERAPY FOR PROSTATE CANCER
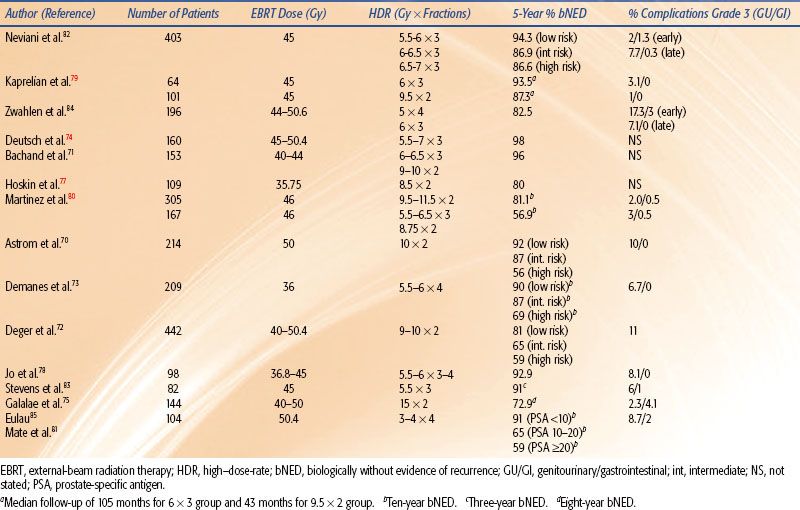
TABLE 25.11 DOSE FRACTIONATION AND ISOEFFECTIVE DOSES (AS IF GIVEN AT 2 GY/FRACTION) OF COMBINED EXTERNAL-BEAM RADIATION THERAPY AND HIGH–DOSE-RATE BRACHYTHERAPY DOSES USED FOR PROSTATE CANCER
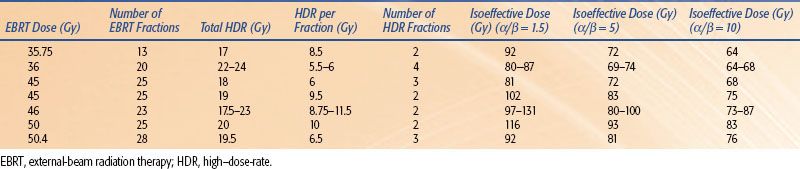
TABLE 25.12 RESULTS OF TREATMENT WITH INTERSTITIAL HIGH–DOSE-RATE BRACHYTHERAPY AS MONOTHERAPY FOR PROSTATE CANCER
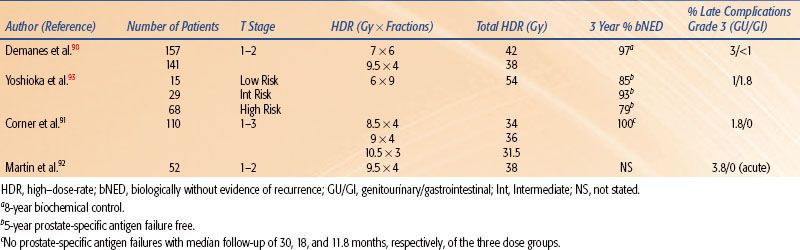
TABLE 25.13 BRACHYTHERAPY IN THE CONSERVATIVE MANAGEMENT OF BREAST CANCER
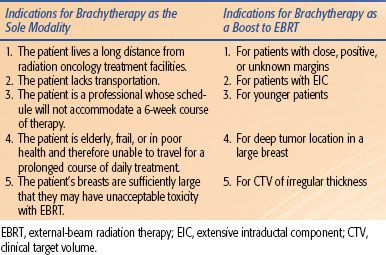
TABLE 25.14 RESULTS OF BREAST-CONSERVING THERAPY WITH LUMPECTOMY PLUS HIGH–DOSE-RATE BRACHYTHERAPY OR AS A BOOST TO EXTERNAL-BEAM RADIATION THERAPY
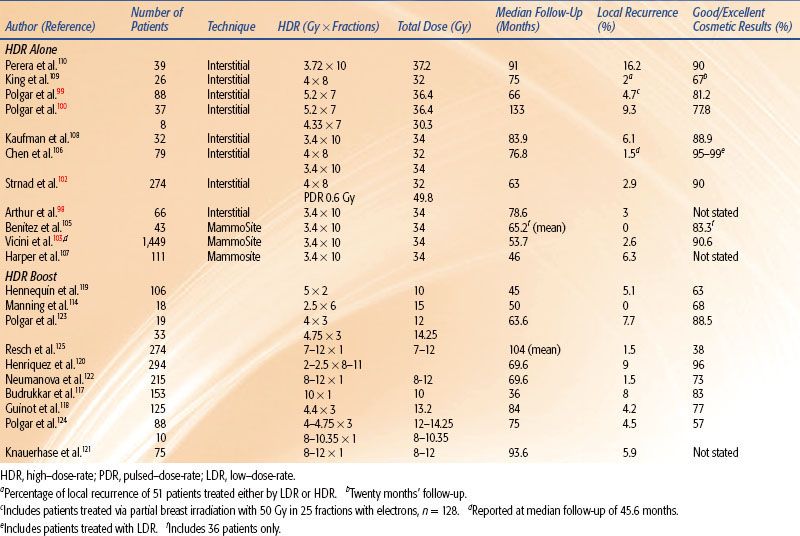
Breast
EBRT is the standard radiation modality used after lumpectomy in the conservative management of breast cancer. Over the past decade there has been an increase in use of brachytherapy as the sole modality of treatment98–100,101,102–103 to decrease the 6-week treatment duration required for a course of EBRT to about 5 days. Table 25.13 lists the patients in whom an accelerated (4 to 5 days) brachytherapy treatment course can be an attractive alternative to 6 weeks of EBRT.104 The ABS recommends a total dose of 34 Gy in 10 fractions to the CTV (lumpectomy site with 1- to 2-cm margin) when HDR brachytherapy is used as the sole modality.104 The HDR treatments of 3.4 Gy are generally given at two fractions per day separated by at least 6 hours. This was also the dose used in a phase II RTOG trial.98 as well as in the phase III National Surgical Adjuvant Breast and Bowel Project (NSABP) B-39 trial. Other prescriptive dosimetric parameters to be met recommended by the ABS include (a) ≥90% of the CTV should receive ≥90% of the dose; (b) V150 and V200 should be <50 cm3 and <10 cm3, respectively, for balloon catheters; and (c) maximum skin isodose should be <145% for balloon catheters.104
The results of HDR brachytherapy as the sole modality are included in Table 25.14.98–100,102,103,105–110 Depending on the selection criteria, final pathologic assessment is necessary to completely evaluate a patient for partial breast brachytherapy, and, therefore, the ABS does not advocate intraoperative treatment delivery at this time.3 The use of a single-channel MammoSite applicator has simplified the brachytherapy procedure.101 Benitez et al.105 reported a good to excellent cosmetic result of 83% and no local recurrences at a median follow-up of 65.2 months in 43 patients treated with the MammoSite applicator (Cytyc Corporation, Marlborough, MA). In addition, a multi-institutional registry trial performed by the American Society of Breast Surgeons to evaluate the clinical use of the MammoSite applicator reported a good to excellent cosmetic result of 90.6% of the 371 patients with 60-month follow-up. Of the total 1,449 cases with a median follow-up of 53.7 months, the ipsilateral local recurrence rate was 2.6%.103 Newer devices such as the SAVI applicator (Cianna Medical, Aliso Viejo, CA) and the Contura applicator (SenoRx Inc., Irvine, CA) are multichanneled and can allow improved dose shaping.
In March 2005, the RTOG, in conjunction with the NSABP, opened a phase III randomized study (NSABP B-39) investigating standard whole-breast radiotherapy versus partial-breast radiotherapy after lumpectomy for women with early-stage breast cancer. The partial-breast treatment arm consists of three therapeutic options: intensity-modulated radiation therapy; HDR brachytherapy via MammoSite, Contura, or SAVI; and HDR brachytherapy via a multicatheter interstitial implant. The required dose for the brachytherapy treatment is 34 Gy given in 10 fractions over 5 days. Target accrual is 4,300 patients, and as of June 2011, 3,980 patients have been enrolled. It is hoped that over time the data will shed some light on the usefulness of partial-breast irradiation and brachytherapy as a sole modality of treatment. Various medical societies have published consensus statement guidelines for the selection of appropriate patients for breast brachytherapy as a sole modality treatment with slight variations.104,111 According to the American Society of Therapeutic Radiology and Oncology (ASTRO) consensus statement, “suitable” patients for accelerated partial-breast irradiation include women 60 years of age and older, with unifocal tumors 2 cm in size or less, negative margins, positive estrogen receptor status, invasive ductal histology in the absence of ductal carcinoma in situ, and absence of nodal involvement. The consensus statement also outlined criteria for “cautionary” and “unsuitable” candidates. The reader is encouraged to review the original consensus statement for full details.111 Thus far local control and cosmesis are similar to whole-breast EBRT with one group quoting 12-year follow-up.100 However, further clinical studies are required to define the most appropriate candidates for breast brachytherapy as a sole modality treatment and to determine the best delivery method of brachytherapy (multicatheter interstitial implant vs. balloon brachytherapy) in such patients.
A newer device, the Axxent (Xoft Inc., Sunnyvale, CA), uses a miniaturized x-ray source to deliver low-energy x-rays within a needle or catheter, thereby mimicking HDR brachytherapy. The reduced radiation protection required for these devices due to limited penetration is a great advantage because it allows brachytherapy to be delivered in a nonshielded procedure room or a regular (unshielded) hospital operating suite. Although longer follow-up is needed to assess the risk of recurrence, early results with the use of this device for accelerated partial-breast irradiation reveal that the procedure is tolerated well and grade 3 adverse events are minimal, with 100% excellent or good cosmetic results at 1 year in one study evaluating 69 patients.112,113 Brachytherapy has been used to boost the EBRT dose in select high-risk patients.114,115–116
Data on the use of HDR as a boost are limited (see Table 25.14).114,117–125 Polgar et al.123 reported the results of a randomized trial involving 207 women with stage I or II breast cancer treated with breast-conserving surgery and whole-breast radiotherapy and subsequently randomized to either no further therapy or radiation boost to the tumor bed. The radiation boost consisted of either 16 Gy of electron irradiation or 12 to 14.5 Gy fractionated HDR brachytherapy. Fifty-two patients were treated with HDR brachytherapy and the 5-year local tumor control rate was 91.4%. Excellent to good cosmesis was reported in 88.5% of patients. Similar results were noted in the group of patients receiving an electron irradiation boost. Because brachytherapy is an invasive procedure, it should be used selectively as a boosting technique. Situations in which brachytherapy may be advantageous as a boost are listed in Table 25.13. The brachytherapy boost can be given before or after EBRT, usually with a 1- to 2-week gap between EBRT and brachytherapy. The ABS recommends a dose fractionation scheme that yields early and late effects approximately equivalent to those of 10 to 20 Gy LDR following 45 to 50 Gy EBRT.7 Biomathematic models are often used to estimate equivalent HDR regimens.12,13 For example, an HDR regimen of five fractions of 310 cGy per fraction should approximate the early and late effects of 20 Gy LDR delivered at 0.5 Gy/hour. Although biomathematic models can be used to estimate the appropriate dose, there is no standardized HDR fractionation schedule that can be recommended for the use of HDR as a boost. Controlled clinical studies are required to further define the most appropriate doses to be used for boost treatment.
Use of interstitial HDR brachytherapy as neoadjuvant treatment in select patients not amenable to breast-conserving surgery at presentation has been reported by Roddiger et al.126 Fifty-three patients who were unable to undergo breast-conserving surgery either because of initial tumor size or an unfavorable breast–tumor ratio were treated with systemic chemotherapy and HDR brachytherapy with 5 Gy twice per day for 3 days (total dose 30 Gy). Of these patients 56.6% went on to receive breast-conserving surgery, and with a median follow-up of 56 months the local recurrence rate was 2%. Further studies are needed to fully define the role of interstitial HDR brachytherapy as neoadjuvant treatment, but these results are encouraging.
Another clinical situation in which HDR brachytherapy has been evaluated is in the setting of salvage therapy to continue maintaining the breast in previously irradiated patients.127,128 Current standard of care of recurrent disease following breast conservation is total mastectomy. Guix et al.127 reported their series of 36 patients who had previously been treated with lumpectomy, external-beam radiation, and HDR brachytherapy boost who subsequently developed an ipsilateral breast recurrence and underwent excision only of the recurrence. Following excision, all patients underwent an HDR brachytherapy implant delivering 30 Gy in 12 fractions over 5 days. With a median follow-up of 89 months, 10-year local control was 89.4% and 10-year disease-free survival was 64.4%. They reported a 90.4% satisfactory cosmetic result. Again, while numbers are small, the possibility of continuing to provide breast conservation in the setting of recurrent disease is encouraging.
Endobronchial Radiation
The use of HDR brachytherapy is well established for palliation of cough, dyspnea, pain, and hemoptysis in patients with advanced or metastatic lung cancer. The use of brachytherapy as a boost to EBRT in curative cases should be restricted to a select group of patients who have predominantly endobronchial disease, are medically inoperable, or have small/occult carcinomas of the lung.
An initial bronchoscopy is performed to evaluate the airway and locate the site of obstruction. Either a 5- or 6-French (Fr) catheter (inserted through the brush channel of the bronchoscope) can be used to deliver the brachytherapy. Use of a 6-Fr catheter allows the HDR source to negotiate tight curves, which is not possible with the 5-Fr catheter. If a 6-Fr catheter is used, a large bronchoscope (with brush channel diameter of at least 2.2 mm) is required. The bronchoscope can be connected to a teaching head or a video monitor so that the radiation oncologist can also visualize the lesion and the catheter. It is extremely important to note the distances between the proximal extent of the tumor and fixed structures such as the carina. The catheter is inserted through the brush channel of the bronchoscope, passed through the tumor, and lodged in one of the smaller bronchi. Fluoroscopic confirmation of the catheter’s position is desirable. The radiation oncologist then pushes the afterloading catheter in while the pulmonologist slowly withdraws the bronchoscope. The use of fluoroscopy assists in keeping the catheter in place during this push–pull technique of bronchoscope removal. The catheter is then secured with tape at the nose, and its position is marked in ink to alert the radiation oncologist in case of displacement. As an additional precautionary measure, the external length of the catheter from the tip of the nostril is noted. If multiple catheters are to be used, the procedure is repeated, taking care to clearly label each catheter. Localization x-rays with radio-opaque dummy wires in the catheter are then obtained. The location of the obstruction and the target length are marked on the x-rays to determine the length to be irradiated and the initial dwell position.
The dose has been prescribed at various points from 0.5 to 2 cm, although 1 cm from the source is commonly used.6 The length to be irradiated usually includes the endobronchial tumor and 1.0- to 2.0-cm proximal and distal margins. If a single catheter is used and there is minimal curvature of the catheter in the area to be irradiated, it is possible to minimize the treatment-planning time by using preplanned dosimetry. For example, Ohio State University has precalculated treatment plans for 3-, 5-, 7-, and 10-cm lengths to be irradiated to 5 or 7.5 Gy at 1 cm from the source using equal dwell times. This allows the treatment to be performed without any delay if standard lengths and doses are used. Individualized image-based treatment planning must be performed if multiple catheters are used.
Palliative Endobronchial Brachytherapy
Candidates for palliative endobronchial brachytherapy include:6,129–131
1. Patients with a significant endobronchial tumor component that causes symptoms such as shortness of breath, hemoptysis, persistent cough, and other signs of postobstructive pneumonitis. Tumors with a predominantly endobronchial component are considered suitable, as opposed to extrinsic tumors that compress the bronchus or the trachea. Endobronchial brachytherapy can generally give quicker palliation of obstruction than EBRT. Furthermore, brachytherapy can be more convenient than 2 to 3 weeks of daily EBRT.
2. Patients who are unable to tolerate any EBRT because of poor lung function.
3. Patients with previous EBRT of sufficient total dose to preclude further EBRT.
A variety of doses have been successfully used by various centers. Total doses ranging from 15 Gy to 30 Gy HDR in one to five fractions calculated at 1 cm have been reported.129,130 The ABS suggests using three weekly fractions of 7.5 Gy each or two fractions of 10 Gy each or four fractions of 6 Gy each prescribed at 1 cm when HDR is used as the sole modality for palliation.6 These fractionation regimens have similar radiobiologic equivalence using the linear-quadratic model,13 and there is no evidence of superiority for one regimen over the other. The benefits of fewer bronchoscopic applications should be weighed against the risks of higher dose per fraction. Additional treatments or doses higher than those suggested can be considered for nonirradiated patients or those who have received limited radiation. When HDR is used as a planned boost to supplement palliative EBRT of 30 Gy in 10 to 12 fractions, the ABS suggests using two fractions of 7.5 Gy each or three fractions of 5 Gy each or four fractions of 4 Gy each (prescribed at 1 cm) in patients with no previous history of thoracic irradiation.6 The interval between fractions is generally 1 to 2 weeks. The brachytherapy dose should be reduced when aggressive chemotherapy is given. Concomitant chemotherapy should be avoided during brachytherapy, unless it is in the context of a clinical trial.
The results from various centers (summarized in Table 25.15) show clinical improvement from 50% to 100% and bronchoscopy response from 59% to 100%.132–145 Comparison of these results is difficult because of the differences in patient population and the variability in dose and fractionation employed. Complications include radiation bronchitis and stenosis, which may occur after endobronchial brachytherapy,146 necessitating close follow-up. Another more serious complication is fatal hemoptysis. The hemoptysis could be a radiation therapy complication resulting from the high dose delivered to the area of the pulmonary artery, or it could represent the failure of treatment due to the progression of disease.147 Multiple courses of brachytherapy, a high previous external-beam radiation dose, a left upper lobe location, or long irradiated segments increase the rate of hemoptysis.148,149 Incidence of fatal hemoptysis varies from 0% to 50% with a median value of 8%.130
TABLE 25.15 SUMMARY OF HIGH–DOSE-RATE ENDOBRONCHIAL BRACHYTHERAPY FOR PALLIATION
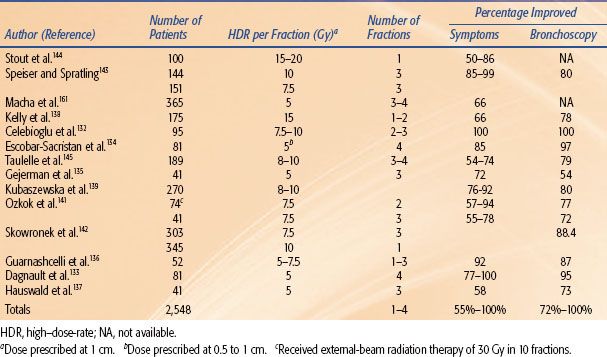
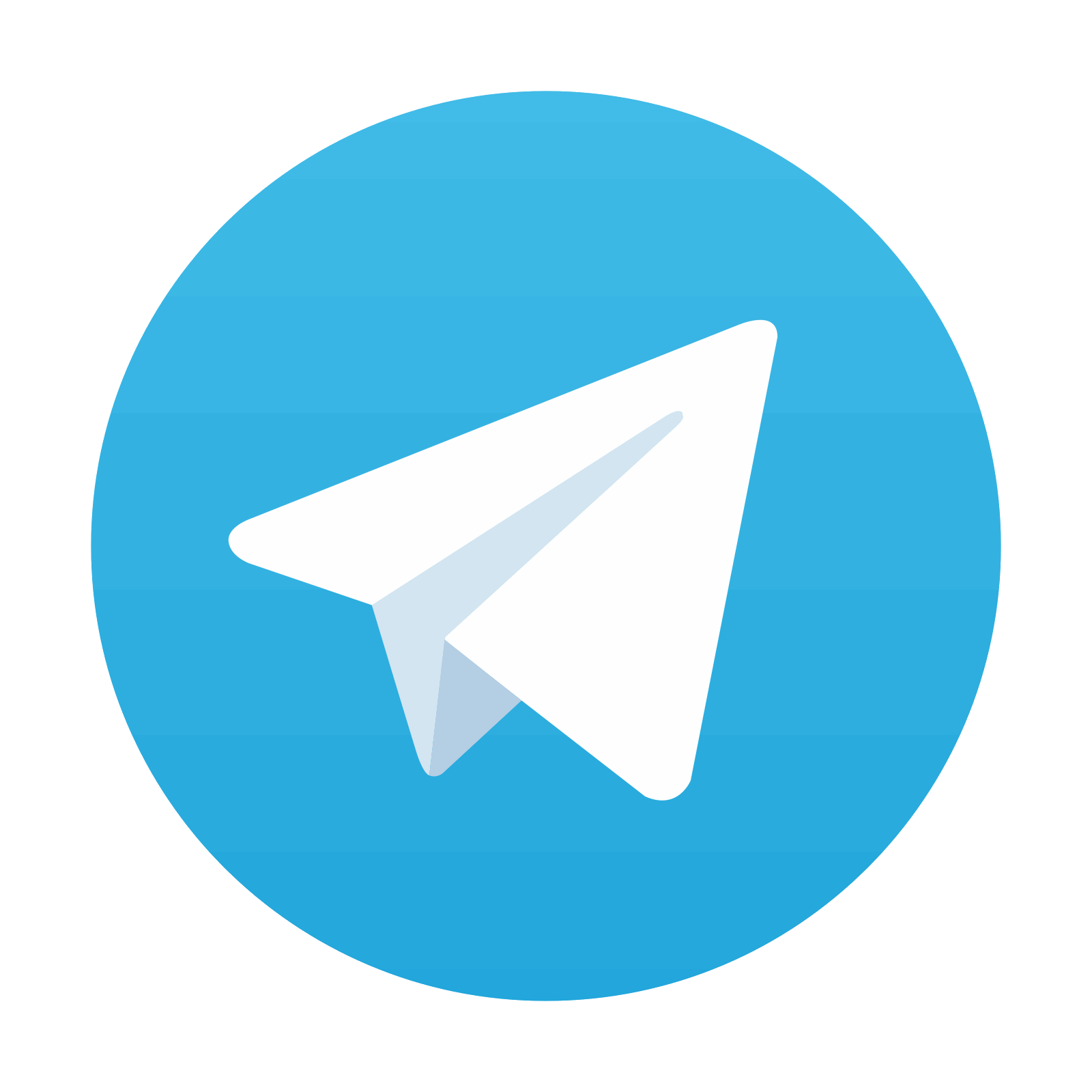