CLASSIFICATION OF HORMONES
Part of “CHAPTER 4 – HORMONAL ACTION“
A classification based on the location of receptors and the nature of the signal used to mediate hormonal action within the cell appears in Table 4-3, and general features of each group are listed in Table 4-4.
The hormones in group I are lipophilic. After secretion, these hormones associate with transport proteins, a process that circumvents the solubility problem while prolonging the plasma half-life by preventing the hormone from being metabolized and excreted. These hormones readily traverse the plasma membrane of all cells and encounter receptors in the cytosol or the nucleus of target cells. The ligand-receptor complex is assumed to be the intracellular messenger in this group.
The second major group consists of water-soluble hormones that bind to the plasma membrane of the target cell. These hormones regulate intracellular metabolic processes through intermediary molecules, called second messengers (the hormone itself is the first messenger), which are generated because of the ligand-receptor interaction. The second-messenger concept arose from the observation of Sutherland33 that epinephrine binds to the plasma membrane of pigeon erythrocytes and increases intracellular cAMP. This was followed by a series of experiments in which cAMP was found to mediate the metabolic effects of many hormones. Hormones that use this mechanism are shown in group IIA. Several hormones, some of which were previously thought to affect cAMP, appear to use cyclic guanosine mono-phosphate (cGMP) (group IIB) or calcium or phosphatidylinositide metabolites (or both) as the intracellular signal (group IIC). The intracellular messenger has been identified as a protein kinase/phosphatase cascade for the hormones listed in group D.
A few hormones fit in more than one category (i.e., some hormones act through cAMP and Ca2+), and assignments change with new information.
MECHANISM OF ACTION OF GROUP I HORMONES
A schematic representation of the mechanism of action of group I hormones (see Table 4-3) is shown in Figure 4-9. These lipophilic molecules probably diffuse through the plasma membrane of all cells but encounter their specific, high-affinity receptor only within target cells. The hormone-receptor complex then undergoes an “activation” reaction that causes size, conformation, and surface charge changes that render it able to bind to chromatin. In some cases—with the glucocorticoid receptor, for example—this process involves the disruption of a receptor–heat shock protein complex. Whether the association
and activation processes occur in the cytoplasm or nucleus appears to depend on the specific hormone. The hormone-receptor complex binds to specific regions of DNA and activates or inactivates specific genes.34,35 By selectively affecting gene transcription and the production of the respective messenger RNAs (mRNAs), the amounts of specific proteins are changed, and metabolic processes are influenced. The effect of each of these hormones is specific; generally, the hormone affects <1% of the proteins or mRNAs in a target cell.
and activation processes occur in the cytoplasm or nucleus appears to depend on the specific hormone. The hormone-receptor complex binds to specific regions of DNA and activates or inactivates specific genes.34,35 By selectively affecting gene transcription and the production of the respective messenger RNAs (mRNAs), the amounts of specific proteins are changed, and metabolic processes are influenced. The effect of each of these hormones is specific; generally, the hormone affects <1% of the proteins or mRNAs in a target cell.
The nuclear actions of steroid hormones predominate and are well defined, but direct actions of these hormones in the cytoplasm and on various organelles and membranes also have been described.36 An effect of estrogens, cAMP, and glucocorticoids on mRNA degradation rates has been demonstrated.37,38,39 and 40 Glucocorticoids also affect posttranslational processing of some proteins.41
Although the biochemistry of gene transcription in mammalian cells is not completely understood, a general model of the structural requirements of steroid regulation of gene transcription can be drawn (Fig. 4-10). Steroid-regulated genes must be in regions of “open” or transcriptionally active chromatin (depicted as the bubble in Fig. 4-9), as defined by their susceptibility to digestion by the enzyme DNase I.42 The open or closed conformation of chromatin may be regulated by the extent of acetylation of the histones that combine with DNA to form chromatin (discussed later this section). Genes have at least two separate regulatory regions in the DNA sequence immediately 5′ of the transcription initiation site. The first of these, the basal promoter element (BPE), is generic, because it is present in some form in all genes.43 This is depicted as containing the consensus sequence GTATA (A/T)A(A/T), called the TATA box (see Chap. 3), because this is the structure found most frequently. Another common component of the BPE is the CAAT box; this sequence or some equivalent structure usually is present. The BPE appears to specify the site of RNA polymerase II attachment to DNA and therefore the accuracy of transcript initiation.44
A second regulatory region is located slightly farther upstream than the BPE, and this may also consist of several discrete elements. This region modulates the frequency of transcript initiation and is less dependent on position and orientation. In these respects, it resembles the transcription enhancer elements found in other genes.45,46 The regulatory region consists of two types of DNA elements in genes that respond to hormones. Hormone response elements (HREs) are short segments of DNA that bind a specific hormone receptor/ligand complex.32,34,35 HREs are often capable of regulating transcription from test promoter/reporter gene constructs, but in most physiologic circumstances, other DNA element/protein complexes are required. The HRE usually is found within 250 nucleotides of the transcription initiation site, but the precise location of the HRE varies from gene to gene.
Identification of an HRE requires that it bind the hormone-receptor complex more avidly than does surrounding DNA or DNA from another source. The HRE also must confer hormone responsiveness. Putative regulatory sequence DNA can be ligated to reporter genes to assess this point. Usually, these fusion genes contain reporter genes not ordinarily influenced by the hormone, and these genes often are not expressed in the tissue being tested. Commonly used reporter genes are firefly luciferase or bacterial chloramphenicol acetyltransferase. The fusion gene is transfected into a target cell, and if the hormone
now regulates the transcription of the reporter gene, an HRE is functionally defined. Position, orientation, and base-substitution effects can be precisely described using this technique.
now regulates the transcription of the reporter gene, an HRE is functionally defined. Position, orientation, and base-substitution effects can be precisely described using this technique.
Although HREs do transmit a hormone response in simple promoter test conditions, the situation is much more complex in most naturally occurring genes. The HRE must interact with other elements (and associated binding proteins) to function optimally. Such assemblies of cis-acting DNA elements and trans acting factors are called hormone response units (HRUs).45 An HRU, therefore, consists of one or more HREs and one or more DNA elements with associated accessory factors (Fig. 4-11). In complex promoters—regulated by a variety of hormones—certain accessory factor components of one HRU (glucocorticoid) may be part of that for another (retinoic acid). This arrangement may provide for the hormonal integration of complex metabolic responses.
The communication between an HRU and the basal transcription apparatus is accomplished by one or more of a class of coregulator molecules (see Fig. 4-11). The first of these described was the cAMP response element binding (CREB) protein, so-called CBP. CBP, through an amino terminal domain, binds to phosphorylated serine 137 of CREB and facilitates transactivation in response to cAMP. It thus is described as a coactivator. CBP and its close relative, p300, interact with a number of signaling molecules, including activator protein-1, signal transducers and activators of transcription, nuclear receptors, and CREB.46
CBP/p300 also binds to the p160 family of coactivators—described in the next paragraph—and to a number of other proteins. It is important to note that CBP/p300 also has intrinsic histone acetyltransferase (HAT) activity. The importance of this is described later in this section. Some of the many actions of CBP/p300 appear to depend on intrinsic enzyme activities and the ability of this protein to serve as a scaffold for the binding of other proteins.
Three other families of coactivator molecules, all of ˜160 kDa, have been described. These members of the p160 family of coactivators include (a) SRC-1 and NCoA-1; (b) GRIP 1, TIF2, and NCoA-2; and (c) p/CIP, ACTR, AIB1, RAC3, and TRAM-1.47 The different names for members within a subfamily often represent species variations or minor splice variants. There is ˜35% amino acid identity between members of the different subfamilies.
The role of these many coactivators is still evolving. It appears that certain combinations are responsible for specific ligand-induced actions through various receptors. The role of HAT is particularly interesting. Mutations of the HAT domain disable many of these transcription factors. Current thinking holds that these HAT activities acetylate histones and result in the remodeling of chromatin into a transcription-efficient environment.48 In keeping with this hypothesis, histone deacetylation is associated with the inactivation of transcription.
In certain instances, the removal of a corepressor complex through a ligand-receptor interaction results in the activation of transcription. For example, in the absence of hormone, the thyroid or retinoic acid receptors are bound to a corepressor complex containing N-CoR or SMRT and associated proteins, some of which have histone deacetylase activity.47 The target gene is repressed until the binding of hormone to the thyroid receptor results in the dissociation of this complex, and gene activation then ensues.
MECHANISM OF ACTION OF GROUP II HORMONES
Most group II hormones are water soluble, have a short plasma half-life and no transport proteins, and initiate a response by binding to a receptor located in the plasma membrane (see Table 4-3 and Table 4-4).
CYCLIC ADENOSINE MONOPHOSPHATE AS THE SECOND MESSENGER
Cyclic AMP (3′,5′ adenylic acid), a ubiquitous nucleotide derived from adenosine triphosphate (ATP) through the action of the enzyme adenylate cyclase (Fig. 4-12), plays a crucial role in the action of several hormones. The intracellular level of cAMP is increased or decreased by various hormones (see Table 4-3). This effect varies from tissue to tissue and sometimes within a given tissue, depending on which specific hormone-receptor interactions occur. For example, epinephrine causes large increases of cAMP in muscle and relatively small changes in liver; the opposite is true of glucagon.
Tissues that respond to several hormones of this group do so through unique receptors converging on a single class of adenylate cyclase molecules. The best example of this is the adipose cell in which epinephrine, ACTH, TSH, glucagon, LH, melanocyte-stimulating
hormone, and vasopressin stimulate adenylate cyclase and increase cAMP.15 Combinations of maximally effective concentrations are not additive, and treatments that destroy one receptor response have no effect on the response of other hormones. Some actions of cAMP occur outside the nucleus, presumably by mediating changes in the degree of phosphorylation of critical enzymes involved in metabolic processes, such as glycogenolysis, gluconeogenesis, and lipolysis.49 Effects of cAMP on the transcription of many genes have been described, and this clearly is a major action of this molecule.50
hormone, and vasopressin stimulate adenylate cyclase and increase cAMP.15 Combinations of maximally effective concentrations are not additive, and treatments that destroy one receptor response have no effect on the response of other hormones. Some actions of cAMP occur outside the nucleus, presumably by mediating changes in the degree of phosphorylation of critical enzymes involved in metabolic processes, such as glycogenolysis, gluconeogenesis, and lipolysis.49 Effects of cAMP on the transcription of many genes have been described, and this clearly is a major action of this molecule.50
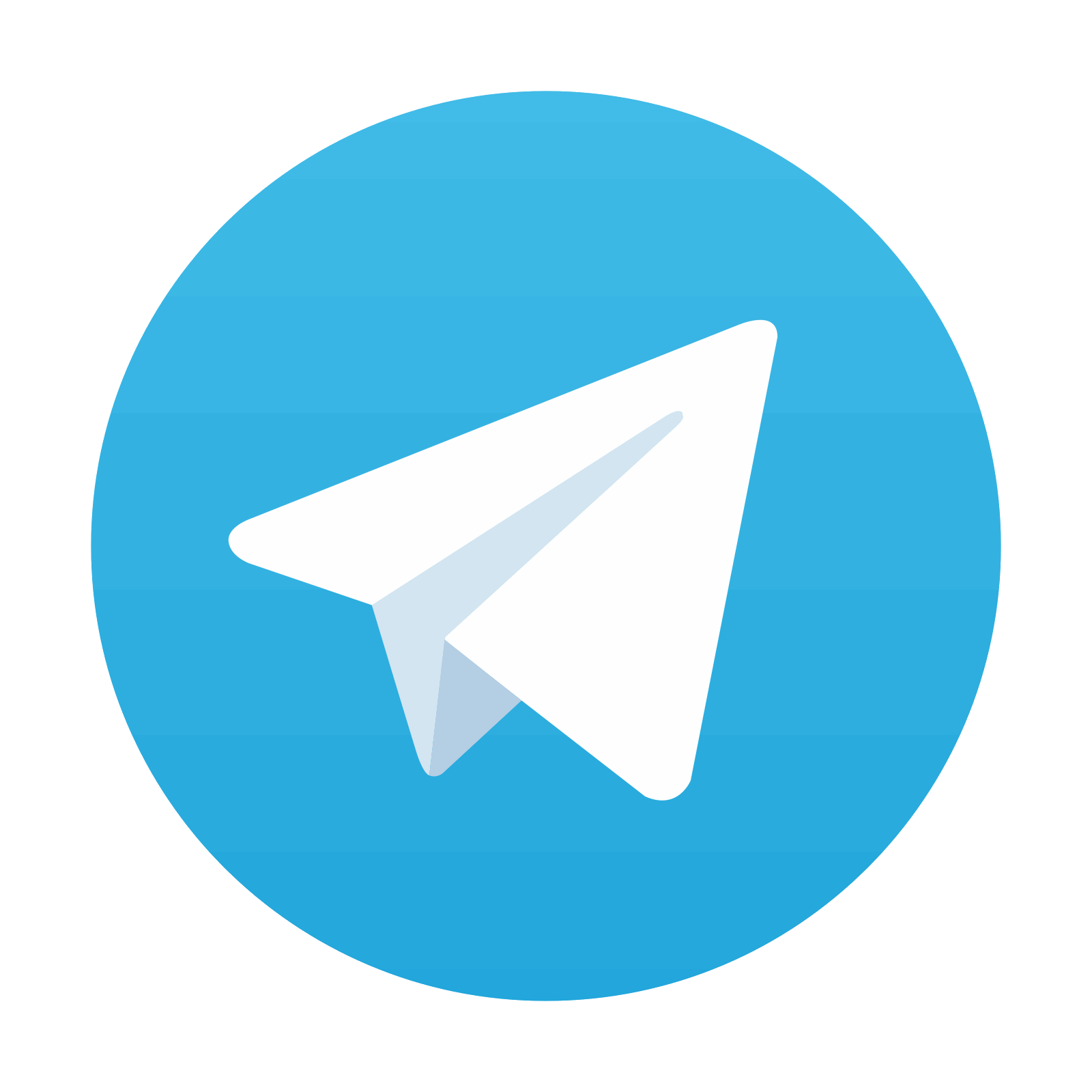
Stay updated, free articles. Join our Telegram channel

Full access? Get Clinical Tree
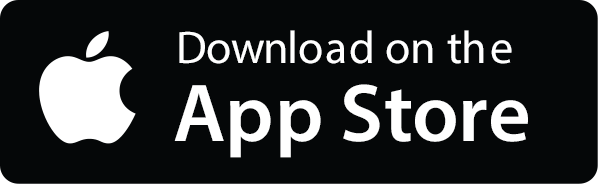
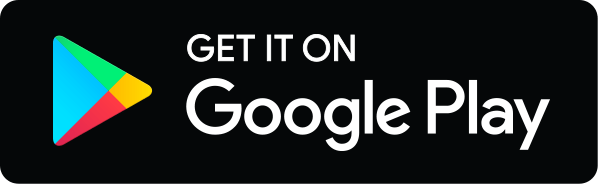
