Chemical Modifiers of Radiation Response
Chemical agents have been administered in conjunction with radiotherapy (RT) for both the enhancement of antitumor therapeutic efficacy and the amelioration of treatment-induced toxicity. Two concepts are fundamental to understanding the rationale for chemical modification of radiation response and to interpreting the studies that have addressed this issue. The first is the therapeutic ratio (TR), which is defined as the TCP/NTCP where TCP is the tumor control probability and NTCP is the normal tissue complication probability. Both of these parameters have sigmoid dose–response curves (Fig. 29.1). The horizontal separation between these two curves for any given treatment will often determine its overall utility. As the separation between these curves increases, the likelihood increases the odds that the treatment will be effective without causing an unacceptable level of morbidity. Conversely, when the two curves are closer together, the treatment may be less effective while causing an unacceptable level of morbidity.
The second concept is the efficacy/toxicity profile of the putative chemical modifier, which can directly affect the TR. A radiosensitizing agent that exacerbates toxicity to the same extent that it improves efficacy (shifting both NTCP and TCP curves to the left) may leave the TR unchanged or worsened and not be clinically practical. Conversely, a radioprotective agent that also reduces RT efficacy against the tumor (shifting both NTCP and TCP curves to the right) also may not affect or even reduce the TR. The intrinsic toxicity of a radioprotector must also be considered when reduction of NTCP is the primary goal of a given chemical modification strategy. A compound that causes significant side effects of its own may render it unsuitable even if it can reduce the treatment-induced toxicity in question. This chapter will explore chemical radiosensitization and radioprotective strategies. The primary focus will be on treatments that have been clinically tested in head and neck cancer in order to amplify these concepts.
FIGURE 29.1. A graphic representation of the therapeutic index (TI). The tumor control probability (TCP) is to the left of the normal tissue complication probability (NTCP) and both are displayed as sigmoid dose–response curves. Larger separations are indicative of higher TIs. Ideally, normal tissue protection strategies would move the NTCP curve to the right without compromising TCP (moving the TCP curve to the right). Ideal therapeutic intensification strategies would move the TCP curve to the left without worsening NTCP (moving the NTCP curve to the left).
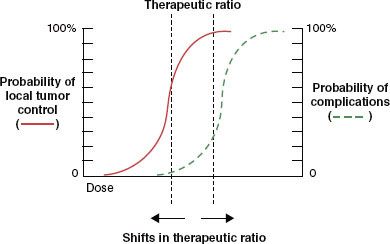
CHEMICAL RADIOSENSITIZATION
The Oxygen Effect
Tumor cell killing is produced by direct ionizations within critical cellular targets as well as by the indirect effect of energy deposited in other cellular molecules including water. Ionizing radiation generates free radicals, which can lead to cellular death via the creation of single strand and double strand breaks in DNA. This damage can be fixed or repaired by the chemical processes of oxidation and reduction, respectively.1 The addition of molecular oxygen to target free radicals produces altered chemical structures that are potentially lethal. Tumor hypoxia reduces radiosensitivity in vitro and in vivo.2,3 Well-oxygenated cells (partial pressure of oxygen or PO2 >10 mm Hg) are approximately 2.5 times more sensitive to a given dose of ionizing radiation than their hypoxic counterparts.
Clinical data clearly demonstrate the existence of tumor hypoxia in head and neck cancer4,5 and extremely strong correlations between hypoxia and both in-field treatment failure and overall survival (Figs. 29.2 and 29.3).6 This effect is independent of presenting stage of disease.7 Tumor hypoxia has also been correlated with local and distant recurrence in carcinoma of the cervix treated with surgery8,9 or RT10 and with distant failure in soft tissue sarcomas treated with surgery and adjuvant RT.11
FIGURE 29.2. The correlation between pretreatment head and neck tumor oxygenation and local-regional disease control after radiotherapy with or without concurrent chemotherapy. Dashed line represents tumor median partial pressure of oxygen (Po2) >10 mm Hg. Solid line represents tumor median Po2 <10 mm Hg.
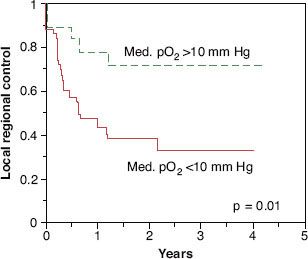
FIGURE 29.3. The correlation between pretreatment head and neck tumor oxygenation and local-regional disease control after radiotherapy with or without concurrent chemotherapy. Dashed line represents tumor median partial pressure of oxygen >10 mm Hg.
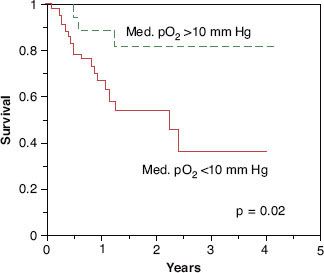
Augmentation of Tumor Oxygenation
Therapeutic attempts to overcome the deleterious effect of tumor hypoxia have followed three general lines of investigation: increased delivery of oxygen to tumor, preferential sensitization of hypoxic cells with oxygen mimetic agents, or cytotoxic agents that selectively target hypoxic tumor cells. Hemoglobin concentration is the major determinant of the oxygen delivery capability of blood to tissue. Hemoglobin oxygen saturation exceeds 90% when the arterial PO2 is >70 mm Hg. Still, oxygen is relatively insoluble in plasma under normobaric conditions. Under hyperbaric conditions, considerable quantities of oxygen can be dissolved into plasma and thus be potentially available for delivery to hypoxic tissues.
Clinical trials of hyperbaric oxygen (HBO) and RT were conducted from the 1950s to the 1970s. Trials conducted in patients with cancers of the central nervous system,12 lung,13 bladder,14 and skin15 showed no benefit from the addition of HBO. Randomized trials conducted in carcinoma of the cervix16 and head and neck17,18 did, however, show improvements in locoregional control and overall survival. The cumbersome logistics associated with HBO delivery in conjunction with RT necessitated the utilization of nonconventional hypofractionated treatment regimens. This reality has prevented HBO from being incorporated into routine clinical use.
Carbogen (95% oxygen [O2]/5% carbon dioxide [CO2]) breathing, with or without concurrent nicotinamide administration, has also been utilized in attempts to improve tumor oxygenation and enhance RT response. The rationale for CO2 addition in the gas breathing mixture is the generation of a mild acidosis that right shifts the oxyhemoglobin association curve, facilitating more unloading of oxygen into the most hypoxic tissues. The rationale for adding nicotinamide, a vitamin B derivative, is based on preclinical studies showing enhancement of tumor blood flow.19,20 Polarographic electrode assessments in cervix and head and neck cancer have demonstrated that carbogen breathing and nicotinamide administration improve tumor oxygenation in some patients.21–23
A randomized trial of hyperfractionated RT with or without carbogen was conducted at the University of Florida from 1996 to 2002.24 The study included patients with T2 to T4 squamous cell carcinoma of the oropharynx, larynx, and hypopharynx and was designed to detect a 20% improvement in 2-year local control in the carbogen arm relative to the RT alone arm, with a 15% improvement in 4-year cause-specific survival. Virtually all enrolled patients in both arms completed their prescribed courses of treatment. The addition of carbogen to RT did not appear to improve any of the planned end points of this trial. Most important, however, was the fact that while the design of this trial called for the enrollment of 675 patients, only 101 were entered over a 5-year period. The trial was therefore significantly underpowered in terms of its ability to detect the desired treatment effects. The inability to accrue patients, however, also called into question the overall viability of strategies utilizing carbogen.
The use of accelerated RT with carbogen and nicotinamide was tested in a phase II trial of 215 head and neck cancer patients.25 Ninety-seven percent had stage III or IV disease, and the primary tumor site was laryngeal in 46%, hypopharyngeal in 23%, and oropharyngeal in 23%. Full compliance with carbogen breathing during RT was obtained in 88% of patients. Nicotinamide was administered 1 to 1.5 hours prior to RT at 60 to 80 mg/kg. Nicotinamide-induced nausea and vomiting necessitated discontinuation of the drug in 10% of patients receiving the lower dose and 31% of patients receiving the higher dose. Five-year locoregional control rates were 48% for hypopharynx primaries, 77% for larynx and 72% for oropharynx primaries.26
Allosteric modifiers of hemoglobin structure have been identified that can shift the oxyhemoglobin dissociation curve to the right and increase O2 delivery to hypoxic tissues.27 One such compound, RSR-13 (efaproxiral) has been tested in animal models and shown to improve tumor oxygenation28 and enhance the effectiveness of RT.29 A phase III open label trial of whole-brain RT and oxygen breathing with or without daily infusion of efaproxiral was conducted in 538 patients with brain metastases.30 Fifty-four percent of the patients had metastatic non–small cell lung cancer and 20% had metastatic breast cancer. Overall, no improvement in survival was detected. A planned subset analysis suggested significant improvement in median survival time in breast cancer patients with sufficient levels of efaproxiral in their erythrocytes.31 However, the phase III ENRICH trial, which examined efaproxiral and supplemental oxygen with whole-brain radiotherapy in breast cancer patients with brain metastases, showed no significant difference in overall survival.32
Anemia is a very powerful adverse prognostic factor in various malignancies, including carcinomas of the lung,33 cervix,34 and head and neck.35–37 Polarographic electrode oxygen measurements in head and neck cancer have demonstrated that anemic patients are significantly more likely to have poorly oxygenated tumors than nonanemic patients, but significant tumor hypoxia has also been detected in patients who are not anemic.6,38 Whether correction or prevention of anemia with blood transfusions or erythropoiesis-stimulating agents can improve treatment outcomes has been investigated in multiple studies.
The use of blood transfusions in cervical cancer patients gained traction after an initial publication from Princess Margaret Hospital showing an improvement in pelvic control and cure rates associated with correction of anemia.39 However, subsequent publications from the same group showed no survival benefit to transfusion when the data were critically re-examined and analyzed on an intent-to-treat basis.40 In head and neck cancer patients, studies suggest that blood transfusions may have a negative effect on survival.41,42 Correction of anemia via erythropoietin (EPO) administration was evaluated in a double-blind, placebo-controlled randomized trial in 351 head and neck patients treated with RT.43 The primary end point was local-regional progression-free survival. Eighty-two percent of patients (54) who received EPO maintained >14 g/dL (women) or 15 g/dL (men), while only 15% of the patients in the placebo arm attained this benchmark. The relative risk of locoregional progression, however, was 1.62 in the EPO arm, compared to placebo (P = .0008) with a similar, detriment seen for survival in those patients who received EPO (relative risk 1.39; P = .02). A systematic review pooling data from five randomized studies with a total of 1,397 patients showed significantly worse overall survival in head and neck cancer patients with the addition of EPO to radiotherapy (odds ratio 0.73; P = .005).44 These poorer outcomes may have been the result of overcorrection of hemoglobin levels with increased thromboembolic events.45 Tumor cells have also been found to express EPO receptors, with stimulation of downstream signaling pathways that may promote a more invasive phenotype.46,47
Sensitization of Hypoxic Cells
Electron-affinic compounds can oxidize radiation-induced free radical damage in the cell to produce increased kill.48 The use of these agents would be particularly attractive in the hypoxic tumor microenvironment, where low oxygen concentrations impair the effectiveness of RT. The 2-nitroimidazoles are one such class of compounds that are metabolized into their active form under hypoxic conditions. Misonidazole, the prototype 2-nitroimidazole, was tested in two randomized trials. The Danish Head and Neck Cancer Study-2 (DAHANCA-2) performed a double-blind randomized trial evaluating the effect of misonidazole given in two drug schedules with split-course irradiation in the treatment of carcinoma of the larynx and pharynx.49 Patients were stratified according to tumor site (larynx vs. pharynx), nodal status, and institution. The total misonidazole dose was 11 g/m2. The study assessed 626 patients. Overall, the misonidazole group did not have significantly better local tumor control than the placebo group. Serious peripheral neuropathy, the dose-limiting toxicity of all nitroimidazole compounds, occurred in 26% of misonidazole-treated patients. The European Organisation for Research and Treatment of Cancer conducted a randomized study of conventional fractionation RT versus modified fractionation RT (three fractions per day) with or without misonidazole in 523 advanced head and neck cancer patients. No differences were seen in treatment outcome.50
Etanidazole (SR2508) is an analog of misonidazole with lower lipid solubility and less neurotoxicity in phase II studies in head and neck cancer.51 A Radiation Therapy Oncology Group (RTOG) phase III study with etanidazole in head and neck tumors entered 521 patients who received conventionally fractionated irradiation with or without etanidazole 2 mg/m2 3 times per week.52 Of those on the etanidazole arm, 77% received at least 14 doses of the drug. No grade III or IV central nervous system or peripheral neuropathy was observed. The 2-year actuarial local tumor control was 40% in each arm, and the survival was 41% and 43%, respectively, in the irradiation alone and the irradiation plus etanidazole arms. A similar study of 374 patients performed in Europe did not show any overall benefit to treatment with etanidazole but did demonstrate increased neurotoxicity in the patients who received the drug.53
Nimorazole is a 5-nitroimidazole of the same structural class as metronidazole.54 Its dose-limiting toxicity is nausea and vomiting; however, the drug can be administered with each radiation treatment. DAHANCA conducted a phase III trial of nimorazole (1.2 g/m2 vs. placebo) for squamous cell cancer of the supraglottic larynx and pharynx.55 There was a statistically significant improvement in locoregional tumor control (49% vs. 33% at 5 years; P = .002) but not for survival, which is consistent with the DAHANCA misonidazole trial. The use of nimorazole has become the standard of care in Denmark but has not been adopted in other countries.
Pharmacologic Targeting of Hypoxic Cells
Mitomycin C (MMC) is an alkylating agent metabolized in regions of low oxygen concentration and preferentially cytotoxic to hypoxic cells. MMC plays an integral role in conjunction with RT and 5-FU (fluorouracil) in the definitive nonsurgical management of squamous cell carcinomas of the anus.56 Yale University investigators examined the concurrent use of MMC in 195 head and neck cancer patients treated on two randomized trials.57 Their treatment program consisted of 68 Gy with or without MMC on days 1 and 43 of RT. Local regional recurrence-free survival was improved with the addition of MMC from 54% to 76% (P = .003). Overall survival improved from 42% to 48%, but this was not statistically significant. The majority of patients in these trials received adjuvant postoperative or preoperative irradiation. Only 74 (38%) received definitive primary RT, and the benefit from the addition of MMC in this subset is unclear.
A three-armed randomized trial conducted by the University of Vienna compared conventionally fractionated (CF) RT (2 Gy daily to 70 Gy) against variation of continuous hyperfractionated accelerated RT with or without MMC (V-CHART + MMC and V-CHART, respectively).58 RT was given as an initial 2.5-Gy fraction followed by 1.65 Gy twice a day to a total dose of 55.3 Gy in 17 days. MMC was given as a 20 mg/m2 bolus on day 5 of RT. Of the 239 patients enrolled, 85% had T3 or T4 primaries and 79% had nodal involvement. Three-year actuarial locoregional control was 48% for V-CHART plus MMC versus 32% for V-CHART and 31% for CF (P = .05 and .03, respectively). Survival including death from all causes was also improved to 41% in the V-CHART plus MMC arm as compared with 31% for V-CHART and 24% for CF (P = .03). The incidence of confluent mucositis was 90% in both experimental arms as compared with 33% in the CF arm. The median time to complete resolution of mucositis was 6 to 7 weeks in all three arms. Grade 3 or 4 hematologic toxicity, primarily thrombocytopenia, developed in 18% of the V-CHART plus MMC patients.
Porfiromycin, a derivative of MMC, provides greater differential cytotoxicity between hypoxic and oxygenated cells in vitro.59 The Yale investigators also conducted a phase III study that compared patients treated with conventionally fractionated radiation plus MMC versus radiation plus porfiromycin.60 Hematologic and nonhematologic toxicity was equivalent in the two treatment arms. With a median follow-up >6 years, MMC was superior to porfiromycin with respect to 5-year local relapse-free survival (91.6% vs. 72.7%; P = .01), local-regional relapse-free survival (82% vs. 65.3%; P = .05), and disease-free survival (72.8% vs. 52.9%; P = .03). There were no significant differences between the two arms with respect to overall survival (49% vs. 54%) or distant metastasis-free rate (80% vs. 76%). Their data supported the continued use of MMC as an adjunct to radiation therapy in advanced head and neck cancer and will become the control arm for future studies.
Tirapazamine (also known as SR-4233; WIN 59075; 3-amino-1,2,4-benzotriazine 1,4-dioxide) is a bioreductive agent preferentially cytotoxic to hypoxic cells in vitro. Twenty-five to 200 times more drug is required to produce the same level of cell killing in aerobic compared to anaerobic conditions.61,62 Under hypoxic conditions, a free radical one-electron reduction product rapidly forms and is believed to be the toxic species, causing oxidative damage to pyrimidines and inducing DNA strand breaks.63 Analysis of DNA and chromosomal breaks following hypoxic exposure to tirapazamine suggests that DNA double-strand breaks are the primary lesions involved in cell death.
This bioreductive agent differs from oxygen-mimetic sensitizers, such as the nitroimidazoles, in that it is itself cytotoxic to hypoxic tissues. Therefore, unlike the oxygen-mimetic sensitizers, tirapazamine-mediated therapeutic enhancement occurs whether the drug is given before or after irradiation.64,65 In fractionated radiation therapy of murine tumors, tirapazamine is as effective as, if not superior to, etanidazole.66 The efficacy of this radiation modifier depends on the number of “effective doses” that can be administered during a course of radiation therapy and the presence of hypoxic tumor cells.67 Tirapazamine can also enhance the cytotoxicity of cisplatin.68
Rischin et al.69 investigated the use of concurrent tirapazamine, cisplatin, and RT in advanced head and neck cancer in a series of trials. A phase I trial established the dosing schedule for tirapazamine given with RT and cisplatin. A randomized phase II study compared RT with cisplatin/tirapazamine versus RT with cisplatin/5-FU and suggested a benefit in the tirapazamine treatment arm (3-year local regional failure-free survival 84% vs. 66%; P = .07).70 Tumor hypoxia imaging was performed with 18-fluorodeoxyglucose-misonidazole positron emission tomography (PET) scanning in 45 of the patients on these studies.71 Hypoxia was identified in primary or nodal sites in 71% of the patients. Eight of 13 (62%) patients with hypoxic tumors who received cisplatin/5-FU experienced subsequent local-regional failure compared to only 1 of 19 (5%) patients with hypoxic tumors who received tirapazamine (hazard ratio [HR] = 15; P = .001). Only 1 of 10 patients with nonhypoxic tumors who received cisplatin/5-FU had a local-regional failure. These findings strongly suggested that the benefit of tirapazamine resulted from improved treatment efficacy against tumor hypoxia.
Two phase III trials were initiated to validate the use of tirapazamine in head and neck cancer. The HeadSTART study enrolled 861 patients and compared standard fractionation RT (70 Gy) with concurrent cisplatin/tirapazamine versus concurrent cisplatin alone.72 The primary end point was overall survival, with 2-year rates of 65.7% in the cisplatin alone arm and 66.2% in the cisplatin/tirapazamine cohort. No differences were seen in failure-free survival, time to locoregional failure, or quality of life. Of note, the patients in this study were not selected based on the presence of tumor hypoxia. Moreover, 12% had major RT planning deficiencies, with those patients having significantly worse locoregional control and overall survival compared to those in protocol compliance.73 A second trial with a planned enrollment of 550 patients was closed early due to excess number of deaths in the cisplatin/tirapazamine arm.74
Biologic Modifiers of Radiation Response
Overexpression of the epidermal growth factor receptor-1 (EGFR-1) is associated with an adverse outcome in squamous head and neck cancer.75 Cetuximab (C225) is a chimeric monoclonal antibody to EGFR. Preclinical studies have demonstrated that cetuximab sensitizes cells to the cytotoxic effects of ionizing irradiation.76,77 Preliminary studies demonstrated that this drug could be safely administered in conjunction with a course of RT for head and neck cancer.78 An open-label phase III trial tested the impact of weekly injections of cetuximab added to a course of RT alone.79 Most patients received accelerated fractionation with concomitant boost, although hyperfractionation and standard fractionation schemes were also permitted. Oral cavity primary tumors were ineligible for enrollment. Two-year local regional increased from 48% with RT to 56% with RT and cetuximab (P = .02). The initial survival advantage seen with the addition of cetuximab to RT has persisted, with updated 5-year overall survival rates of 45.6% versus 36.4% (P = .018).80
This trial provided an important proof of principle that adding a biologically targeted agent to a physically targeted modality improved therapeutic outcome. One-third of the patients enrolled had stage III disease, however, and thus had less advanced disease with more favorable prognoses than a significant proportion of patients who typically undergo chemoradiotherapy (CRT). A more favorable prognosis and improved treatment response has also been seen in patients with oropharyngeal squamous cell cancers associated with the human papillomavirus (HPV).81 Whether RT with cetuximab is as effective or less toxic than RT with cisplatin in this select population is being examined by the phase III RTOG-1016 study.
A separate phase III study, RTOG-0522, randomized patients with locally advanced head and neck cancer to receive RT and concurrent cisplatin with or without cetuximab. Results of the study were presented at the American Society of Clinical Oncology annual meeting in 2011.82 Treatment intensification with the addition of cetuximab to CRT did not improve 2-year progression-free or overall survival. Subset analyses of HPV-positive and HPV-negative patients are currently being performed. Still, EGFR inhibition remains a very active area of investigation in head and neck cancer. Agents currently in clinical trial include fully humanized monoclonal antibodies and orally administered small molecule inhibitors of the tyrosine kinase domains of the EGFR family of receptors.
CHEMICAL RADIOPROTECTION
The protection of normal tissues from the deleterious effects of radiation is a critical component in the development of a comprehensive treatment plan. Strategies for the accomplishment of this aim include the physical manipulation of the beam, modification of the fractionation schedule, and pharmacologic manipulation of the radiation response. Physical radiation protection rests on the principle of exclusion of normal tissue from the high-dose region and may be accomplished by contouring the shape of the radiation beam, the use of multiple treatment fields, the use of different beam energies, and modulation of the dose delivery from each beam (intensity-modulated radiation therapy [IMRT]). Modified fractionation typically uses multiple fractions of treatment per day as opposed to the conventional once daily paradigm in order to exploit the differing radiation repair capabilities of normal tissues as opposed to tumors. Physical modification of the treatment beam and altered fractionation are discussed elsewhere.
Protection
Pharmacologic radioprotection itself can be classified into three categories: protection, mitigation, and treatment. The direct cytotoxicity of ionizing irradiation results from the generation of free radicals that cause DNA strand breaks and lead to mitotic cell death. Amifostine (WR2721; Ethyol, Medimmune Inc, Gaithersburg, MD) is the prototype pharmacologic radioprotector that functions via free radical scavenging. Amifostine is a thiol containing pro drug that preferentially accumulates in the kidneys and salivary glands where it is metabolized to its active moiety, WR1065.83
An open-label phase III randomized trial was conducted from 1995 to 1997 to assess the ability of this drug to reduce the incidence of grade 2 or higher acute and late xerostomia and grade 3 or higher acute mucositis.84 Patients enrolled in this trial received curative intent or adjuvant postoperative irradiation without concurrent chemotherapy. All treatment was delivered with conventional once daily fractionation of 1.8 to 2.0 Gy. Curative intent delivery consisted of 66 to 70 Gy total dose, and postoperative irradiation was delivered at 50 to 60 Gy total dose depending on the patient’s assessed risk for recurrence. IMRT was not utilized, and inclusion of >75% of both parotid glands was required for inclusion in the study. Those patients who were randomized to receive amifostine were given a daily dose of 200 mg/m2 intravenously for 15 to 30 minutes every day prior to each fraction of radiotherapy.
Three hundred three patients were enrolled in this trial, and minimum follow-up was 2 years. Amifostine did not reduce the incidence of grade 3 mucositis but did significantly reduce the incidence of acute and long-term grade >2 xerostomia. One-year post-RT, the incidence was 34% versus 56% for patients who had received amifostine versus those who had not (P = .002). Unstimulated saliva production >0.1 g was also more common in patients who had received amifostine (72% vs. 49%; P = .003). Two years post-RT, amifostine use was still associated with a significantly lower incidence of xerostomia, although the magnitude of benefit was lower (19% vs. 36%; P = .05). The lower incidences in both groups of patients also suggest some late recovery of salivary function. Reinforcing this idea of late recovery of salivary function is the fact that the percentage of patients who did not receive amifostine but who could exceed the >0.1 g of unstimulated saliva threshold had increased to 57%.85
Severe toxicity (CTC grade >3) attributable to amifostine occurred in <10% of patients in this trial and consisted of nausea and vomiting and transient hypotension. Nearly two-thirds of the patients had less severe grades of these side effects. Drug-related toxicity did cause approximately 20% of patients to discontinue amifostine prior to completing radiotherapy. Subcutaneous administration of the drug causes less nausea, vomiting, and hypotension than intravenous dosing but is associated with an increased risk of cutaneous toxicity, which again causes 15% to 20% of patients to not complete a full course of amifostine in conjunction with their radiation.86 The incidence of severe cutaneous toxicity, including erythema multiforme, Stevens-Johnson syndrome, and toxic epidermal necrolysis, is 6 to 9 in 100,000.87
Some have argued that the size of this trial made it underpowered to detect a very small compromise in survival caused by amifostine (tumor protection).88 This argument is technically correct but overlooks the reality that absolute refutation of a small compromise of antitumor efficacy attributable to amifostine would have required an equivalence trial. Demonstration that amifostine reduced survival from a hypothetical 45% to 40% (P = .05; 80% power) would have necessitated >1,200 patients per study arm.89 Such a large study cannot be performed in head and neck cancer, because patient resources are too scarce. The largest randomized head and neck trial ever conducted, RTOG-9003, required 8 years to enroll 1,113 patients into four treatment arms.90 A meta-analysis with individual patient data from 12 trials and 1,119 patients examined the impact of amifostine on survival in patients treated with RT or CRT. The majority of patients (65%) had head and neck cancers, with 33% lung cancers and 2% pelvic carcinomas. The hazard ratio of death was 0.98 (95% confidence interval, 0.84 to 1.14; P = .78).91
The potential of amifostine as a protector against radiation-induced esophagitis during the treatment of non–small cell lung cancer was studied in a randomized trial conducted by the RTOG.92,93 No reduction in the incidence of grade 3 esophagitis was observed, although less swallowing dysfunction was observed in the patients who received amifostine. Part of the explanation for this absence may be attributable to the study design, which utilized a hyperfractionated radiation schedule 5 days per week (69.6 Gy total dose) and concurrent carboplatin/paclitaxel. Amifostine 500 mg intravenous was delivered 4 days per week prior to the afternoon fraction only. Moreover, 28% of the patients did not complete the full course of the drug either because of toxicity or refusal. Consequently, approximately 50% of the RT was delivered in the absence of the radioprotective drug in those patients who were randomized to receive it. Preclinical study of amifostine delivered daily in conjunction with fractionated lung and esophageal irradiation has demonstrated morphologic and immunohistochemical evidence of radioprotection.94–96
Amifostine is approved by the U.S. Food and Drug Administration for xerostomia in the setting of RT alone. The majority of both curative intent and adjuvant postoperative RT for head and neck cancer, with large target volumes that put the parotid glands at risk, is now delivered in conjunction with concurrent chemotherapy. Small phase II and III trials suggest that amifostine has a cytoprotective benefit in the chemoradiation setting, but level 1 evidence is lacking.97,98 Moreover, the widespread adoption of IMRT with its ability to spare one or both parotid glands and reduce the incidence of xerostomia compared to conventional, non-IMRT techniques has further reduced the role for this drug.99 The utility of amifostine in conjunction with IMRT has been investigated in small settings with inconclusive results.100
Mitigation
Administration of compounds that mitigate damage caused by previous radiation exposure constitutes a different approach to the management of radiation-induced toxicity. This strategy contrasts to the classical free radical scavenging radioprotective mechanism of drugs such as amifostine. The leading drug under development in this category is palifermin. Palifermin is a recombinant human keratinocyte growth factor that belongs to the fibroblast growth factor (FGF-7) family of cytokines. It stimulates cellular proliferation and differentiation in a variety of epithelial tissues including mucosa throughout the alimentary tract, salivary glands, and type II pneumocytes. Palifermin also regulates intrinsic glutathione-mediated cytoprotective mechanisms. Administration of palifermin in preclinical rodent models leads to a significant thickening of oral tongue mucosa.101 Preclinical studies of fractionated RT have revealed that the administration of palifermin leads to increases in the dose of RT necessary to induce ulcerative mucositis and to reductions in the duration of this ulceration when it does occur.102,103 Parotid gland production of saliva is also preserved when palifermin is administered in the setting of RT in preclinical systems. Preclinical evaluation of palifermin in a rodent model has also demonstrated that administration of a single dose of this drug after completion of a course of fractionated thoracic irradiation significantly reduces the severity and duration of pneumonitis and the severity of pulmonary fibrosis (Fig. 29.4).104
The ability of palifermin to reduce mucositis in a clinical setting has been tested in a pivotal phase III double-blind placebo-controlled trial of patients with non-Hodgkin lymphoma undergoing bone marrow transplantation.105 The bone marrow ablative regimen consisted of 12 Gy of total-body irradiation (TBI) given at 1.5 Gy twice a day. Thereafter, etoposide (VP-16) and cyclophosphamide were administered. Palifermin was delivered prior to the initiation of TBI and again after the completion of chemotherapy, which also corresponded to 5 days after the completion of TBI. The dose schedule of palifermin was 60 mcg/kg/d 3 times for both administrations. This trial enrolled 212 patients who were equally divided between the placebo and palifermin arms. The World Health Organization (WHO) scoring system was used. The incidence of grade 3 or 4 mucositis approached 90% in the placebo arm as opposed to approximately 60% in the palifermin arm. For those patients who developed this level of toxicity, the duration was significantly reduced from 10.4 days in the placebo arm to 3.7 days in the palifermin arm (P <.001). Grade IV mucositis developed in 62% of the placebo arm patients and only 20% of the palifermin arm patients (P >.001). Mean duration of grade IV mucositis was reduced from 6.2 days to 3.3 days with the use of this drug (P <.001).
A phase II study examined the safety and efficacy of palifermin in locally advanced head and neck cancer patients.106 Patients were randomized 2 to 1 between palifermin and placebo. Palifermin was delivered at a dose of 60 mcg/kg. Institutions had the discretion to deliver RT via conventional once-daily 2-Gy fractions or with an accelerated hyperfractionated regimen of 1.25 Gy twice daily. One hundred patients were enrolled, of whom 34 received accelerated hyperfractionation and the remainder received standard fractionation. The first dose was delivered prior to the initiation of CRT and then every Friday afternoon after the last fraction of radiation. Two additional doses of palifermin were given 1 and 2 weeks after the completion of RT for a total of 10 doses of the drug. Palifermin did not reduce the incidence or duration of mucosal or salivary gland toxicity. The subset of patients receiving hyperfractionated radiation, however, showed significant improvements in the duration and severity of mucositis (Fig. 29.5). They also had improved swallowing function and less salivary gland toxicity relative to patients who received placebo.
A subsequent randomized phase III study examined a higher dose of palifermin at 180 mcg/kg to reduce oral mucositis in 188 patients with locally advanced head and neck cancer treated with CRT.107 Palifermin was administered prior to starting CRT and once weekly for 7 weeks. The incidence of severe oral mucositis, the primary end point, was significantly lower in the palifermin arm compared to placebo (54% vs. 69%; P = .041). Both overall survival and progression-free survival were similar as well. However, no statistically significant differences emerged in secondary efficacy end points such as narcotic doses and duration of treatment breaks. A similar randomized phase III study examined palifermin at 120 mcg/kg in 186 head and neck cancer patients treated with postoperative CRT.108 Palifermin again reduced the time to development and duration of WHO grade 3 or 4 oral mucositis without differences in patient-reported pain scores, treatment breaks, or efficacy. The precise role for palifermin in the management of head and neck cancer remains to be established.
Treatment
Radioprotectors and radiation mitigators are both designed to minimize the risk of clonogenic death of normal cells and subsequent disruption of the protective mucosal barrier. Head and neck RT also initiates a local cytokine cascade, which includes interleukin-1 and -6 and tumor necrosis factor-α (TNF-α). An inflammatory response results, which contributes to the ultimate anatomic disruption of the mucosa. Secondary bacterial and fungal overgrowth are thought to exacerbate the local pathophysiology.
Sucralfate, a basic aluminum salt of sucrose, is used in the treatment of peptic ulcer disease. It provides a protective coating to ulcerated tissue by means of binding to exposed proteins in damaged cells.109 It also stimulates mucus production, mitosis, and surface migration of cells. Sucralfate has been tested in several double-blind placebo-controlled randomized trials. Despite the attractive conceptual nature of using it to ameliorate mucositis, the clinical data do not show any benefit from sucralfate.1,110–112
FIGURE 29.4. Mitigation of radiation-induced fibrosis attributable to single dose of recombinant human keratinocyte growth factor (KGF) administered after a course of fractionated hemithorax irradiation. The hematoxylin and eosin slides show the morphologic changes in the alveoli induced by irradiation including the inflammatory infiltrate and alveolar wall thickening. The Masson’s trichrome panels show the collagen deposition that is characteristic of fibrosis. KGF was given either at 5 mg/kg or 15 mg/kg. Less injury is seen with the higher dose of KGF, suggesting that a dose–response effect exists.
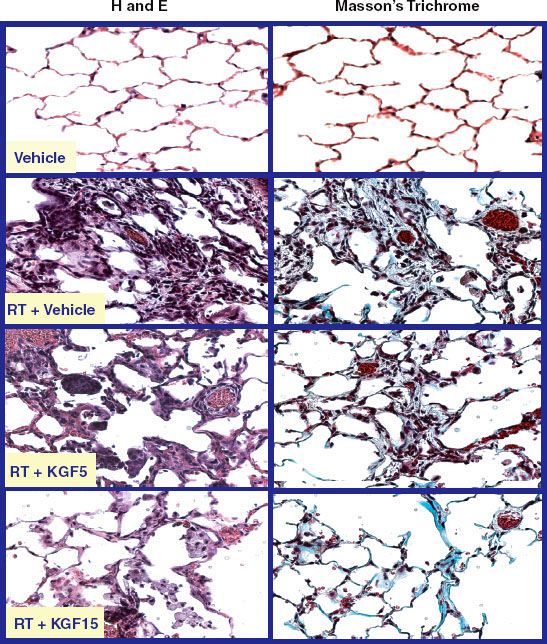
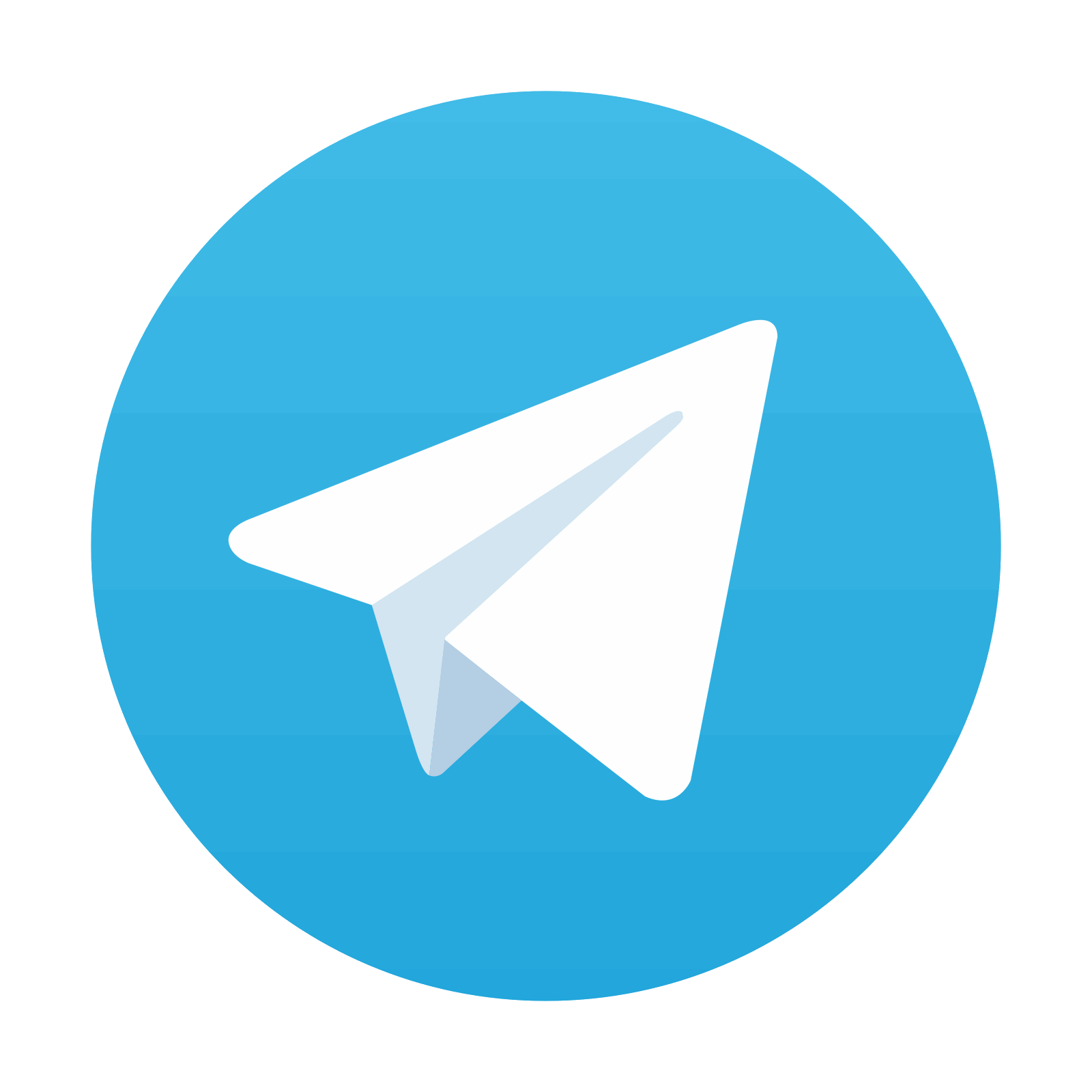