Abstract
Hemolytic disease of the fetus and newborn (HDFN) is the immune mediated destruction of fetal and neonatal red blood cells by maternal antibody. HDFN occurs when the fetal red blood cells express a paternally inherited antigen not present on maternal red blood cells. The spectrum of illness ranges from clinically insignificant to that of a critically ill, anemic, hydropic, and jaundiced infant at risk for bilirubin-induced brain damage (kernicterus).
Introduction
Hemolytic disease of the fetus and newborn (HDFN) is the immune mediated destruction of fetal and neonatal red blood cells by maternal antibody. HDFN occurs when the fetal red blood cells express a paternally inherited antigen not present on maternal red blood cells. The spectrum of illness ranges from clinically insignificant to that of a critically ill, anemic, hydropic, and jaundiced infant at risk for bilirubin-induced brain damage (kernicterus).
It was the pivotal paper by Dr. Louis K. Diamond in 1932 that clearly identified the development of the maternal antibody in response to the incompatibility of the fetal red cells with maternal red cells even though the Rhesus red cell antigen had not yet been identified [1]. Parallel to the understanding of HDFN, Landsteiner began the process of identification of the red cell antigens at the start of the twentieth century, first with the identification of the ABO system and then with the identification of the Rh system [2]. He described the development of antibodies against rhesus monkeys red cells in rabbits. He suggested that this was a new antigen distinct from the ABO system and called it the Rhesus antigen. This name is now well established in spite of the early controversy, since the antigen identified was a simian antigen which is related but is not the same as the human antigen. Current International Society of Blood Transfusion terminology for this blood group is Rh and the symbol is RH.
The term HDFN replaced the original term, erythroblastosis fetalis, when the immunological mechanisms responsible for fetal anemia and jaundice were confirmed [3]. The D antigen of the Rh blood group, designated RHD, continues to be the most commonly identified antigenic stimulus for HDFN. RHD negative mothers give birth to RHD positive fetuses in about 9% of European-ancestry pregnancies. In a first pregnancy, without prophylaxis, 15%–17% of these at-risk mothers would become immunized against RHD and more than 20% of the babies had HDFN requiring treatment [4]. Subsequent pregnancies with the same maternal fetal antigen mismatch have a higher frequency of affected infants. Overall, among women with established anti-D antibodies, 50% of RHD babies with HDFN will have minimal disease requiring no intervention, 25% will have disease requiring intervention after birth to prevent brain injury by bilirubin and the effects of significant anemia, and 25% will require in utero intervention to prevent severe morbidity or mortality [4]. The case fatality rate for RHD HDFN in 1986 in the USA was 2.6% [5]. In 1998 the death rate attributed to HDFN in USA was reported to be nine infants per million live newborns [6]. During the period from 1970 through the late 1990s the use of prophylactic anti-D antibody increased, and the frequency of both maternal alloimmunization and RHD HDFN decreased dramatically [7]. At the same time there has been a gradual increase in the frequency in HDFN due to other antigens [8]. A review of antibodies recognizing red cell antigens that have been detected either in maternal serum during pregnancy or described to cause HDFN are listed in Table 9.1. This chapter will review maternal anti-erythrocyte antibody development, the red cell antigens involved, the diagnosis of HDFN in neonates, and management of the affected neonate and fetus.
Table 9.1 Maternal antibodies recognizing blood group antigens. Blood group antigens involved in HDFN
System namei | Symbolii | ISBT numberiii | Antigensiv | Frequency HDFNv | Severityvi | References (population)vii |
---|---|---|---|---|---|---|
ABO | ABO | 001 | ABO | Common | Mild–moderate | [23, 158–159] |
Rh | RH | 004 | D, c, C, E, e | Common | Severe | [53–54, 57–58, 60–61, 63, 160–161] |
Cw, Cx | Described | Severe | [162–163] (Pakistan) | |||
Ew, G, Rh17 | Described | [164–166] | ||||
Kell | KEL | 006 | K, k, Ku | Common | Severe | [65–66, 69–70, 167–168, 169–170, 171] |
Jsb | Moderate–severe | [74–75] | ||||
Kpa, Kpb | Mild–moderate | [73] | ||||
Jsa, Ula | Mild–moderate | |||||
Duffy | FY | 008 | Fya | Described | Severe | [87–89, 172] |
Fyb | Uncommon | [86] | ||||
Fy3 | Described | Mild–moderate | [91] | |||
Kidd | JK | 009 | Jka, Jkb, Jk3 | Mild–moderate | [95–96, 98, 97, 172, 173] | |
MNS | MNS | 002 | M | Described | Mild–severe delayed | [103–105, 172, 174–175] (Japan) |
N | Described | [176] | ||||
S, U, Vw | Moderate | Severe | [106, 172, 107–108, 177] | |||
s | ||||||
Mta | Described | Mild–moderate | [178–179] | |||
Hil, Mur | [175, 180] (Taiwan) | |||||
Langereis | LAN | 033 | Lan | Described | Severe | [181] |
Landsteiner–Wiener | LW | 016 | LW | Described | [182] | |
P | P1PK | 003 | P1 | Not described | Maternal antibody | [172] |
Lutheran | LU | 005 | Lua | Described | Mild | [183–184] |
Lub | Not described | Maternal antibody | [185] | |||
Lewis | LE | 007 | Lea | Described | Mild | [186, 187] |
Leb | Not described | Possible | [188–189] | |||
Diego | DI | 010 | Di | Described | Mild to severe | [64] |
Colton | CO | 015 | Coa, Co3 | Described | Mild | [190] |
Gerbich | GE | 020 | Ge2, Ge3 | Described | Mild–severe | [191, 149, 192 ] (Hispanic) |
Scianna | SC | 013 | Sc2, Rd | Described | Mild–severe or delayed | [193–194] |
JR | JR | 032 | Jra | Described | Mild–severe | [195–198] (Japan) |
Dombrock | DO | 014 | Not described | Maternal antibody | [199] | |
Yt | YT | 011 | Not described | Maternal antibody | [200] | |
Chido/ Rodgers | CH/RG | 017 | Not described | Maternal antibody | [201] | |
JMH | JMH | 026 | Not described | Maternal antibody | [201] | |
Knops | KN | 022 | Not described | Maternal antibody | [201] | |
Cromer | CROM | 021 | Not described | Maternal antibody | [61–64] | |
Notes:
i Common blood group system by common name.
ii International Society of Blood Transfusion (ISBT) blood group symbol.
iii ISBT blood group system number.
iv Specific antigens within the blood group system.
v Indicates whether HDFN has been described. In the situation of maternal antibody detected without apparent HDFN, the designation “Not described” is used.
vi This gives the reader a brief overall sense of severity of HDFN described in the literature for the particular red blood cell group system.
Development of Maternal Anti-Erythrocyte Antibodies
There are essentially three mechanisms for the development of maternal anti-erythrocyte antibodies: (1) innate production, (2) sensitization via fetomaternal hemorrhage, and (3) iatrogenic exposure in the form of prior RBC transfusion. The major blood group system, ABO, is characterized by innate antibody production. Individuals with blood type O innately produce anti-A and anti-B antibodies. No prior maternal blood exposure is necessary for formation of these antibodies.
The second mechanism, fetomaternal hemorrhage (FMH) is infrequent prior to the start of the third trimester [9–11]. Less than 15% of all new anti-RHD antibodies identified prior to term in primigravida women are detected before 28 weeks’ gestation [9]. FMH occurs most frequently around the time of delivery. The association between the week of gestation and increasing frequency of FMH is likely a direct effect of the growing fetus exerting greater force on placental tissue with normal maternal movement. Additionally, the fetal blood volume increases with increasing fetal age. Events during pregnancy associated with an increased risk of FMH are detailed in Table 9.2 [12]. Knowledge of the association of FMH with these events drives the recommendation for additional doses of anti-RHD to prevent maternal isoimmunization.
Known previous transfusions |
Medical history with potential for unappreciated history of transfusions |
|
Risk for unappreciated fetal maternal hemorrhage |
|
Prior pregnancy history |
|
The third mechanism is iatrogenic exposure through maternal transfusion which may be required for obstetric complications. Additionally, with the increasing medical success, children with complex medical problems are living into young adulthood. Some of these young adults will have received blood transfusions as part of their medical care. Although transfused mothers represent only a small percentage of patients at risk for HDFN, they represent 50% of the pregnancies affected by Kell HDFN [13]. Now that RHD HDFN is largely preventable, preventing iatrogenic cases of HDFN should be a priority [14–15].
The expanded utilization of reproductive technology has led to unexpected risk for development of HDFN. Frequent changes in health-care providers creates the opportunity for both intentional and unintentional omission of details of medical history. The use of donor oocytes, donor sperm, or adopted embryos may go unshared with a subsequent obstetrics provider or pediatrician. Reproductive technologies can lead to a fetus or neonate with HDFN presenting with fetomaternal red cell antigen mismatches that appear biologically impossible based on known maternal and paternal red cell phenotypes [16–17]. For example, the AB+ adopted embryo born to an O− mother and O− father.
Red Blood Cell Antigen Systems
The International Society of Blood Transfusion (ISBT) currently recognizes 36 red blood cell systems, each consisting of one or more red cell surface antigens controlled at a single gene locus or two very closely linked homologous genes that have little or no observable recombination. Each system has a name, a 2–3 letter symbol, and a 3-digit number (mainly used in computer logging). Within each system are a variable number of antigens. Tables linking system names, gene designations, chromosomal locations, cluster of differentiation (CD) numbers, and the numeric designations are available on the ISBT website page “Red Cell Immunogenetics and Blood Group Terminology” (available at http://www.isbtweb.org/working-parties/red-cell-immunogenetics-and-blood-group-terminology ). We have included the current ISBT terminology in Table 9.1 which outlines the blood groups antigens that have been described to result in a spectrum of symptoms from simply detectable maternal antibody to red cell antigen up to severe HDFN requiring a high level intervention.
Immunoglobulin is transported across the placenta by an energy dependent process that uses an Fc receptor, FcRn [18]. IgA and IgM are not transported since they are not bound by FcRn, and there is no other transfer mechanism. Maternal IgG transfer appears to begin in the 15th week, but it is minimal up to the 22nd week of gestation in normal pregnancies. After the 22nd week IgG transport rapidly accelerates, such that the IgG concentration in term neonates equals or exceeds the maternal level [19]. In addition to the amount and avidity of antibody produced, the IgG subclass that is produced can also affect transport across the placenta and hemolysis [20, 21].
ABO
ABO was the first red blood cell antigen system identified. There are two antigens (A, B) resulting in four blood types (A, B, AB, O). The A and B antigens are glycotransferases that differ by four amino acids. The O allele contains a single nucleotide deletion resulting in a frame-shift mutation and coding for a protein without enzymatic activity [22]. Every immune competent person who does not express the A or B protein, will innately produce antibody to that antigen. Symptomatic HDFN due to ABO mismatch occurs almost exclusively in infants whose mothers are blood type O [23]. Some A or B infants born to blood type O mothers will develop more severe hemolytic disease than others. HDFN from ABO mismatch is characterized predominantly by hyperbilirubinemia and less anemia compare to HDFN as a result of anti-RHD. A blood type B infant of a blood type O mother is associated with greater hyperbilirubinemia risk than a blood type A infant of an O mother [24–25]. The greater risk for hyperbilirubinemia appears to be particularly in neonates with mothers of African origin [26–29]. One recent report highlights several such neonates who experienced progressive hyperbilirubinemia despite phototherapy and required an exchange transfusion [30].
Routine cord blood screening of infants born to type O mothers has been recommended in the past and remains common practice in many nurseries. The literature, however, suggests that such screening is not warranted given the cost and low yield [23, 24, 31]. Recommendations by the American Association of Blood Banks, and the American Academy of Pediatrics state cord blood testing for infant’s blood type and direct antiglobin test (direct Coombs test, DAT) is not required provided there is appropriate birth hospitalization bilirubin surveillance and risk assessment before discharge [32–33]. A blood type, DAT, and measurement of total serum bilirubin is indicated in the evaluation of any newborn with jaundice developing in the first 24 hours of life and/or clinically significant hyperbilirubinemia including those treated with phototherapy.
Rh System
The RH antigens are coded for by two closely related genes on chromosome 1, RHD and RHCE. The genes are codominant which results in protein product of the gene inherited from each parent. The RHCE gene codes for C, c, E, and e polypeptides [34, 35]. The RH proteins are only expressed in erythroid cells [35–36]. In fetal development the RHD antigen can be detected on red blood cells (RBC) as early as the 7th week of gestation. The highest density of red cell antigens is on mature red blood cells.
The RHD gene codes for the D protein. Unlike small c and e, small d only indicates the absence of D protein expression (in this chapter designated as RHD negative). In Caucasian populations the lack of RHD expression is due to gene deletion, and occurs in 11%–35% of Caucasian ethnic groups [37]. Among the 0.5%–7% eastern Asian and southern Africans who are serologically RHD negative, as many as 66% have a grossly intact RHD gene [38–42]. RHD negative status in Africans can be due to a 37 bp insertion which encodes a premature stop codon [35].
RHD is the most immunogenic of the RBC antigens [43]. The sensitivity of the immune system to RHD is illustrated by the fact that multiple 0.01 ml doses of whole blood can be sufficient for immunization [44]. Untreated, 1%–2% of RHD negative mothers of RHD positive, ABO compatible fetuses will have anti-RHD detectable at the end of a first pregnancy [45–46]. At 3 to 6 months postpartum a total of 9% of this group of mothers will have a positive IAT, with the increase attributable to immunization by FMH at delivery. During a second RHD positive, ABO compatible pregnancy an additional 8% of RHD negative mothers will have anti-RHD detected, consistent with secondary immunization following undetected primary immunization during the first pregnancy. Thus, 15%–17% of mothers will be immunized following a first ABO compatible, RHD incompatible pregnancy without immunoprophylaxis.
Type O RHD negative mothers carrying type A or B RHD fetuses have a lower frequency of anti-RHD immunization [47–50]. This is due to the removal of fetal red blood cells from maternal circulation by the agglutinating IgM anti-A or anti-B antibody preventing sensitization to the RHD antigen [15].
Anti-E antibodies are the most frequently found anti-Rh system antibodies found in pregnancy but are less immunogenic than anti-D antibodies [51, 52, 53]. However, significant HDFN may occur in up to 20% of infants of sensitized mothers and severe disease including hydrops may occur. Anti-e is less frequent and significant HDFN is less frequent but may occur [54].
The rare RH null phenotype results from deletion of RHAG which must be present for RH antigens to be present on the surface of the red blood cell. Rh null appears serologically the same as RHcde but is characterized hematologically by normochromic, hemolytic anemia, and complete lack of the RH antigens in the RBC membrane [55].
Anti-RHc, “little c” occurs less frequently than anti-RHE, at an incidence of about 0.7 per 1,000 pregnancies, but 20%–30% of affected neonates will require postnatal intervention [56–58]. Therefore anti-RHc is second only to RHD as a cause of HDFN. Importantly, one report described a frequency of 13% of anti-c positive pregnancies resulting in severe anemia or hydrops [59]. Others have reported the same with anti-c, but at a lower rate [60–61]. RHC, “big C” is reported to be the target of maternal hemolytic antibodies in 0.1 to 0.2 per 1,000 pregnancies. Of those with anti-C antibodies, one-third of affected babies showed some sign of being affected, but less than 10% required any treatment.
RHCw, an antigen associated with HDFN, is a mutated form of RHC and differs from RHC by a single amino acid [62]. Each of the RH antigens (D, C, c, E, e), the RH variants Cw, Cx, Ew, Ces, and G, have been implicated as causes of significant HDFN (see Table 9.1). Anti-e antibody, as the least immunogenic of the Rh group antigens, is uncommon and has been reported to cause by comparison more mild HDFN [43, 63].
Kell
The Kell antigen system (KEL) was named for the woman in whom the antibody was first identified [64]. Many of the KEL variants differ only by a single amino acid. Although the majority of these antigens are without clinical relevance to newborns, antibody to K, k, Ku, and Jsb are significant causes of moderate to severe HDFN [65–66].
The KEL glycoprotein is the first erythroid specific antigen known to be expressed during erythroid development [67]. Thus, antibody targeted against this antigen can profoundly affect red blood cell production. This observation helps to explain the manifestations of HDFN caused by antibodies to KEL in which anemia and reticulocytopenia may be more severe, and hyperbilirubinemia less severe, than when other antigens are involved [68–70].
Anti-K antibody is found in about 0.1% of pregnant women [71]. However, most of these women have developed antibodies due to transfusion exposure [13]. Since only 9% of people of European ancestry and 2% of people of African ancestry are antigen positive for K, and almost all are heterozygous, the likelihood that a fetus will express K is small [72]. Antibody to KEL has been associated with a lower critical titer, 8, than for RH antigens [65 ]. When an antibody to K is detected in initial prenatal screens, there is great importance in determination of paternal antigen status. With paternal heterozygosity and a titer of 1:8 or greater, fetal antigen determination is indicated to guide future monitoring. Of those infants born to mothers with anti-K and known to express the K antigen, 40% in one series were severely affected, 24% required phototherapy, and the remainder received no intervention [13]. In another series 73% of antigen positive infants were severely affected [68]. The other KEL associated antigens: k, Kpb [73], and Jsb [67, 74–75] have been associated with HDFN that in the case of Jsb was severe.
Duffy
The Duffy (FY) antigen is a red cell membrane glycoprotein which functions as an attachment site for the malaria agent, Plasmodium vivax, and as a receptor for multiple chemokines [76–79]. There are two principal forms, Fya and Fyb, which are codominant and differ by a single amino acid [80]. The null variant genotype FY/FY and phenotype Fy(a−b−) is a single base pair change in the promoter region at the binding site for the hematopoietic transcription factor GATA-1 [81–82]. The null variant Fy(a−b−) is found in 95% of West Africans and 68% of African Americans [77]. The lack of erythroid expression of Fya and Fyb in the FY/FY individuals is considered to be among the many RBC adaptations to the environmental pressure of malaria [83]. The molecular basis for the null variant is an example of tissue-specific gene regulation, as the Duffy antigen is expressed in nonerythroid tissues, including those with the FY/FY genotype, apparently under control of other transcription factors [84].
Although Western African ancestry individuals have a high frequency of lacking the Fya and Fyb antigens on red blood cells, a population based study in the United States did not find an increased frequency of antibody to Fya among individuals self-classified as black compared to those self-classified as white [85]. In this series in which 45 patients had developed antibody to Fya, none had developed antibody to Fyb. Fyb is calculated to have an immunogenic potency one-eighth that of Fya [85]. It has not been implicated as a cause of HDFN requiring treatment except in a single case [8, 86]. In two case series that included pregnant women with anti-Fya, two-thirds of mothers had a history of blood transfusion [56, 87]. Antibody to Fya has been implicated in significant HDFN [88–89]. The anti-Fya titer has been a poor predictor of severity of neonatal disease [89]. Two case series described 40 Fya positive infants born to mothers with anti-Fya. Of these 40, 2 received intrauterine transfusions, 3 received exchange transfusions, and 3 were treated with phototherapy alone [57, 89]. Fetal genotype determination of Fya is described [89–90].
Another distinct antigenic site, Fy3, is absent on those who are Fy(a−b−), but detectable on those who are Fy(a+b−) and those who are Fy (a−b+). Anti-Fy3 has been associated with alloimmunization and very rarely with moderate HDFN [91].
Kidd
Kidd (JK) antigen is a glycoprotein, which functions as a constitutive urea transporter in red blood cells and kidney [92]. Jka and Jkb, differ by a single base pair and amino acid [93]. The Kidd glycoprotein carries an additional antigen, Jk3, which appears to generate an antibody response only in Kidd null Jk(a−b−) subjects. Anti-Jk3 will react with all Jka or Jkb positive cells. Jk(a−b−) is uncommon except among people of Polynesian ancestry [94].
Each of these three epitopes has been associated with HDFN requiring intervention in a small number of cases [95–98]. Antibody to the Jka and Jkb antigens has been associated with positive direct antiglobulin test and hyperbilirubinemia requiring phototherapy [95–96].
MNS
Antigens of the MN reside on GYPA and Ss resides on GYPB. GYPA and GYPB are heavily glycosylated and are considered sialomucins. Cells lacking GYPA have altered ion transport capabilities. GYPA may interact with the red cell protein band 3, an anion exchange protein altering membrane flexibility [99, 100]. Glycophorin A (GYPA) is one of the most abundant red cell proteins. During erythroid maturation GYPA is detectable shortly after the KEL antigenic group [67]. Anti-M is usually an IgM antibody. In one series, antibodies to MNS accounted for less than 5% of the HDFN [101]. While more recent series report 10% HDFN due to anti-M/N [102–103]. Intervention required ranged from no intervention to exchange transfusion [104–105]. In a review of Japanese cases, severe HDFN occurred despite low maternal titers. Additionally, some of these infants developed late onset anemia suggesting anti-M may be able to suppress hematopoiesis analogous to Kell [103]. The frequency of detected anti-S is about 5% that of anti-RHD in the post immunoprophylaxis era. A strong association with transfusion history is reported, but the antibody is too weak to titrate in two-thirds of the cases. Moderately severe HDFN has occurred and there is one case report of IUT for HDFN due to anti-S [106]. The glycophorin B molecule carries the U antigen as well as S/s. Rarely anti-U has been implicated in HDFN, but among the 15 reported cases all affected infants had an DAT titer of greater than 1:256 [107]. Another infant had low titers and required only phototherapy for mild HDFN [108].
Clinical Management
With the advent of prevention strategies, prenatal screening, management of affected pregnancies, and effective in-utero treatment, HDFN has become a relatively rare and, usually, manageable disease. It is important, however, to not underestimate its ability to cause significant harm in the form of perinatal loss, prematurity, and neonatal morbidity when it does occur.
Prenatal Prevention
Women who are RH negative lack the RHD antigen on their red cells. If the fetus inherits the RHD gene from the father, RH positive fetal cells can, through the course of normal pregnancy, access the maternal intravascular compartment and elicit a maternal immune response in the form of IgG antibodies against the RHD antigen. The antibody can cross the placenta and attack fetal red cells, resulting in HDFN.
In the absence of prevention strategies, 14% of affected pregnancies result in stillbirth and half result in neonatal death or severe morbidity [109]. In the United States, the strategy of postnatal injection of anti-RHD immune globulin resulted in a steady decline in the frequency of anti-D in pregnant women and a concomitant decrease in the frequency of perinatal death due to anti-D HDFN [5, 110]. During the period from its introduction in 1968 to its widespread use by 1983, the reduction in maternal immunization and perinatal death were each about 90%. After 10 to 15 years of routine administration of postnatal IM anti-D immune globulin to RHD negative, and anti-RHD antibody negative mothers, the immunization rate of at-risk mothers had decreased from 16% to 2%, and deaths due to HDFN were decreased from 22–45 per 100,000 live births to 3.9–7.5 per 100,000 live births [46, 110–111]. This decrease was primarily attained by the application of postnatal anti-RHD prophylaxis, but it was aided during that time by the birth pattern of a smaller number of second and subsequent pregnancies with the accompanying risk for repeat antigen exposure [112].
The addition of a dose of anti-RHD immune globulin at 28 weeks further decreased the frequency of immunization ten-fold from approximately 2% with postnatal prophylaxis alone to 0.2% with pre- and postnatal anti-RHD immune globulin [45–46, 113]. In addition, specific antepartum events including spontaneous or induced abortion [114–115], amninocentesis [116–117], cordocentesis [118–119], chorionic villus sampling [120–121], antepartum hemorrhage, blunt abdominal trauma [122], external cephalic version [123], and others were identified as increasing the risk of immunization to RHD. (See Table 9.2.) Subsequent studies showed a decrease in immunization when anti-D immune globulin was given following amniocentesis and pregnancy termination [124–125]. Policies were developed for additional immune prophylaxis to prevent immunization following the above specific high-risk events for FMH [126].
The American College of Obstetricians and Gynecologists has published evidence-based clinical practice guidelines for the prevention of RHD alloimmunization that simplify and standardize prenatal care [127]. These recommendations include maternal ABO blood type and antibody screen at the first prenatal visit. RHD negative, antibody negative women are rescreened at 24–28 weeks and, if still antibody screen negative, are given a 300 microgram intramuscular injection of anti-D immune globulin. After delivery of an RHD positive infant, RH negative women who remain antibody negative are given a repeat 300 microgram dose of anti-D immune globulin within 72 hours of delivery.
This antepartum strategy is predicated on the fetal antigen status being an unknown. Paternal blood typing may be helpful if the father is RH negative. If paternity is certain, then the fetus is also negative, and no further intervention is warranted. Alternatively, cell-free fetal DNA in the maternal circulation, even in the first trimester, is highly sensitive (99%) and specific (95%) for fetal RHD genotype [128–130]. Knowing the fetal genotype can eliminate the need for further intervention. Although this strategy may not be cost-effective or pragmatic currently, it is likely to become so as the price and availability of the technology improve and could eventually change the management algorithm.
According to the American Association of Blood Banks, pregnant patients who are blood grouped as weak D (formerly Du) have historically been categorized as D antigen negative with recommendations to utilize RHD immune globulin. The College of American Pathologists now recommends RHD genotype. This would allow weak D phenotypes 1, 2, and 3 to be treated as RH positive. Such a shift could potentially save 24,700 injections of RHD IgG [131].
If the maternal antibody screen is positive at pregnancy registration or becomes positive for anti-D or other red cell antigen antibodies known to cause fetal immune-mediated hemolysis, the fetus is at risk for HDFN, and additional surveillance is warranted. Available diagnostic and surveillance options for determining the presence and severity of HDFN include serial testing of maternal antibody titer by indirect antiglobin test (IAT). Other techniques include: determination of fetal genotype by DNA-based techniques on maternal blood or amniotic fluid, middle cerebral artery Doppler velocity studies by ultrasound, amniocentesis using optical density change of the amniotic fluid as an indicator of hemolytic activity in the fetus, and direct determination of fetal hemoglobin level and red cell antigen type by percutaneous umbilical cord blood sampling. The best option to employ is determined by the antigen involved, the mother’s obstetric history, the father’s genotype, and the gestational age at which the antibody is first detected. An algorithm for the monitoring of pregnancies at risk for RHD alloimmunization is demonstrated in Table 9.3. Much of the algorithm applies to pregnancies at risk for HDFN from alloimmunization against any red cell antigen.
Maternal antibody status at beginning of pregnancy | Maternal antibody status (prior to Rhogam) 24–28 weeks | Rhogam given? | Maternal antibody status at delivery | Maternal antibody | Diagnosis | Infant risk for HDFN |
---|---|---|---|---|---|---|
Negative | Negative | Yes | Positive | Anti-D | Passive anti-D; | Unlikely* |
Rhogam effect | ||||||
Negative | Negative | No | Positive | Anti-D | Late RHD sensitization | Yes |
Negative | Positive | No | Positive | Anti-D | Early RHD sensitization | Yes |
Positive | Positive | No | Positive | Anti-D | Sensitized pregnancy to RHD | Yes |
Negative | Negative | Yes | Positive | Non-D antibody | Late sensitization to non-D antigen | Yes |
A notable exception to this surveillance strategy is Kell alloimmunization. The Kell antibody has the ability to not only attack mature red cells in the fetal circulation, but it can also attack progenitor cells in the fetal bone marrow resulting in decreased red cell production. This two-pronged attack of decreased red cell production and increased destruction can result in rapid and severe anemia irrespective of maternal antibody concentration. MCA Doppler surveillance is initiated at 18 weeks [65].
For a pregnant woman who is newly identified to be at risk for HDFN based on an antibody screen result, the IAT titer continues to be the measure that is most widely used to determine whether invasive testing should be done. Serial antibody titers are performed at regular intervals, usually every 2 to 4 weeks in the second trimester and every 1 to 2 weeks in the last trimester. Each lab that performs serial measurement of maternal anti-blood group antibodies determines a critical titer defined as the lowest titer that has been associated with fetal compromise at their institution. The critical titer is specific for a given antigen since it varies with the antigen. An additional criterion that may be employed is the sequential increase in titer greater than 2 dilutions, for example, 2 increasing to 16. Given the limited frequency of HDFN, many labs do not have the volume of patients to determine their own critical titers. Therefore, any titer between 16 and 32 is generally used.
Historically, an IAT anti-D titer of 32 or greater triggered the need for close follow up and the use of more invasive procedures. Amniocentesis could then begin as early as 14 weeks in antigen-sensitized mothers. The fluid was evaluated for bilirubin pigment concentration, by measuring the optical density of the amniotic fluid specimen at 450 nm, the spectral peak for bilirubin, and subtracting the value for the control fluid at 450 nm producing the OD450. The results were then plotted on Liley’s original graph that used data from samples taken from 27 weeks’ gestation to term and was divided into three regions by two curves [132]. Later, Queenan et al. collected data for fetuses from 14 weeks to term and constructed a graph with four regions [133].
Noninvasive ultrasound monitoring using the middle cerebral artery (MCA) peak systolic velocity (PSV) has almost entirely replaced invasive monitoring. Using current MCA velocity criteria to identify significant anemia the false negative rate is 0%, and the false positive rate is 12%. Importantly, the ability to identify fetuses with significant anemia is independent of ultrasound changes associated with hydrops [134]. This technology has now become the standard for monitoring pregnancies at risk of HDFN, avoiding invasive procedures such as amniocentesis and cordocentesis that carry an inherent risk and can accelerate the disease via additional FMH.
In women with an obstetric history of a prior affected fetus/neonate who carry an RH-positive fetus in the current pregnancy, HDFN can be expected to be more severe. MCA PSV monitoring should begin earlier and applied more frequently in this instance.
In Utero Therapy
The mainstay of treatment for severe HDFN is intrauterine transfusion (IUT). IUT corrects fetal anemia and decreases the volume of circulating fetal RBCs carrying the antigen responsible for HDFN. After several IUTs, the percent of fetal RBCs is negligible. The pregnancy can, therefore, continue to term with periodic transfusions. In experienced hands, the fetal risk of IUT is remarkably low [135]. Long-term outcome studies suggest low levels of neurodevelopment impairment of survivors [136].
Intrauterine transfusion can be accomplished by two approaches: intraperitoneal and intravascular transfusion. Historically, intraperitoneal transfusion preceded intravascular for technical reasons. With the advent of high-resolution, real-time ultrasound, which allowed for fetal intravascular access, intravascular transfusion replaced intraperitoneal except in unusual circumstances. Intraperitoneal transfusion relies on packed red blood cells (PRBCs) to be gradually absorbed from the intraperitoneal compartment. It is still employed when intravascular access is technically impossible, such as in maternal obesity and early gestation, or as an adjunct to intravascular transfusion to potentially extend the interval between transfusions. Intraperitoneal IUT is less effective, however, in the presence of ascites. Importantly, it does not provide direct information about the effectiveness of the treatment. Intravascular access, however, holds multiple advantages over intraperitoneal. Not only does it quickly correct the anemia, but it also allows for accurate and direct assessment of anemia and monitoring of the treatment’s success. It allows for the sampling of fetal blood, which can be used for a myriad of informative laboratory tests such as blood type, hemoglobin level, platelet count, reticulocyte count, and Kleihauer–Betke. These laboratory values allow for an accurate assessment of the degree of anemia as well as the fetal response to treatment and can be used to guide management.
Donor blood must be negative for the RBC antigen(s) involved in HDFN and cross-matched with the mother to avoid sensitization to a new antigen. They should be as fresh as possible, spun to a high concentration (hematocrit of 75%), CMV negative, irradiated, and leukodepleted (see Chapter 20, Table 20.4). Considerable time may be required for the blood bank to match and prepare donor blood, especially if multiple antibodies are involved. Close communication with the blood bank and transfusion medicine is helpful in procedure planning. Maternal blood may be the best source of PRBCs in cases of multiple maternal alloantibodies, but pregnant women are often excluded from blood donation for various reasons [137]. See Chapter 20 on Transfusion Practices for more information.
The procedure consists of two distinct components: percutaneous umbilical blood sampling (PUBS) and IUT. Sampling involves achieving intravascular access and collecting a sample of fetal blood. If fetal anemia (2 standard deviations below the gestational age mean) is confirmed, transfusion is initiated immediately [138]. Given the challenge and inherent risks of obtaining intravascular access, all equipment, supplies, and personnel must be in place and prepared to perform an IUT. The major risk of IUT is fetal bradycardia, most often secondary to a large bolus of viscous blood. Bradycardia typically, but not always, resolves with temporary interruption of IUT. Contingency planning must include the ability to perform an emergency cesarean section after a gestational age when neonatal survival would be anticipated. This planning must include the informed consent of the patient and advanced notification of anesthesia, nursing, and neonatology teams. Antenatal corticosteroids should be administered in proximity to the next planned IUT after viability is achieved.
Intrauterine transfusion is initiated when convincing evidence for severe fetal anemia exists, usually by middle cerebral artery Doppler assessment 1.5 multiples of the median [134]. Once transfusion is initiated, it is generally repeated at progressively elongated intervals of 2, 3, and then 4 weeks. This strategy takes into account the volume of fetal cells within the intravascular compartment. Repeated IUTs increase the volume of donor RBCs and decreases the percent of fetal RBCs, which can be monitored by the fetal reticulocyte count and Kleihauer–Betke on subsequent samplings. Donor blood cells have a longer half-life than fetal cells, allowing for extension of the interval between IUTs. An alternative strategy is to follow MCA Doppler studies after the first IUT to determine the timing of the next. The efficiency of this strategy diminishes after the second IUT as donor RBCs have different flow properties than fetal cells [139]. Typically, the last IUT is performed at 35 weeks with delivery at 38 weeks. Cesarean section may be reserved for routine indications.
In the rare circumstance of severe disease at very early gestation, both plasmapheresis and intravenous immunoglobulin (IVIg) have been used to mitigate the disease until intrauterine transfusion is technically achievable. Both interventions are associated with maternal side effects, high cost, and short duration of effect [139–140].
Pregnancies complicated by HDFN managed by IUT evidence an overall survival of approximately 90%. Long-term neurologic outcomes are generally favorable, matching the baseline incidence for neurologic impairment in the general population, except in the case of severe hydrops fetalis [136]. The presence of hydrops fetalis decreases survival, highlighting the importance of efforts to prevent its occurrence [141–142].
Perinatal Communication: A Critical Handoff
To assure timely and effective neonatal care, all medical providers attending the mother and newborn must know the status of the maternal anti-erythrocyte antibody screen and if positive recognize its potential to herald significant hyperbilirubinemia in the neonate [143]. If known prenatally, obstetric providers should communicate the finding of the positive maternal anti-erythrocyte antibody screen to labor and delivery, the newborn nursery and neonatal care takers well prior to delivery. Prenatal neonatology consultation for pregnancies complicated by maternal alloimmunization should be solicited to provide parental counsel on the postnatal evaluation and management of the newborn and to coordinate neonatal care.
Similarly, if first identified during the delivery hospitalization, newborn caretakers should be notified of a positive maternal anti-erythrocyte antibody screen in a timely fashion, prior to and no later than the time of delivery. This is necessary to secure cord blood typing and direct antiglobulin testing to determine whether the maternal anti-erythrocyte antibody or antibodies pose a risk for neonatal hemolysis and ensure there are no delays in identifying significant early hyperbilirubinemia or in initiating treatment.
Immune mediated hemolysis is often quite robust and resultant early hyperbilirubinemia can be rapidly progressive. It is therefore essential that the critical information of a positive maternal anti-erythrocyte antibody screen be clearly communicated across the perinatal continuum between obstetric and newborn caretakers to ensure newborn safety.
Additionally, post-natal diagnosis of HDFN should be transmitted back to the obstetrics provider in preparation for future pregnancies.
Interpreting a Positive Maternal Antibody Screen at the Time of Delivery
Accurate interpretation of a positive maternal anti-erythrocyte antibody screen at the time of delivery is necessary to ensure proper newborn care. This will include the determination of what red blood cell antigen the maternal antibody is directed toward and when the mother became antibody positive. This may require a call to the blood bank to clarify or confirm the antibody screen results. Then it is necessary to determine if the infant carries the antigen to which the antibody is directed. This requires a blood type and DAT on the infant, ideally from cord blood to ensure a timely evaluation.
If the mother is RHD positive and has a positive anti-erythrocyte antibody screen, then she is sensitized, and the blood bank will tell you which non-RHD antigen the antibody is directed toward. Alternatively, if the mother is RHD negative and has a positive anti-erythrocyte antibody screen then it is necessary to determine whether she was antibody negative at the beginning of the pregnancy, antibody negative at 24–28 weeks’ gestation prior to RH immunoglobulin administration, determine whether she received RH immunoglobulin, and whether the antibody is anti-D. Table 9.3 shows how to interpret the maternal antibody status in RHD negative woman at the time of delivery [30]. Figure 9.1 is an algorithm to help newborn care takers determine how best to evaluate and manage the neonate in the context of a positive maternal anti-erythrocyte antibody screen [143].
It is also important to recognize that a RHD positive infant delivered to a RHD-negative woman during the first isoimmunized pregnancy (antibody screen negative at the beginning of the pregnancy but then turns positive) is at risk for HDFN. In fact, at least 20% of such infants are affected and require treatment in terms of either phototherapy or exchange transfusion [144]. The same holds true for RHD positive women during the first alloimmunized pregnancy.
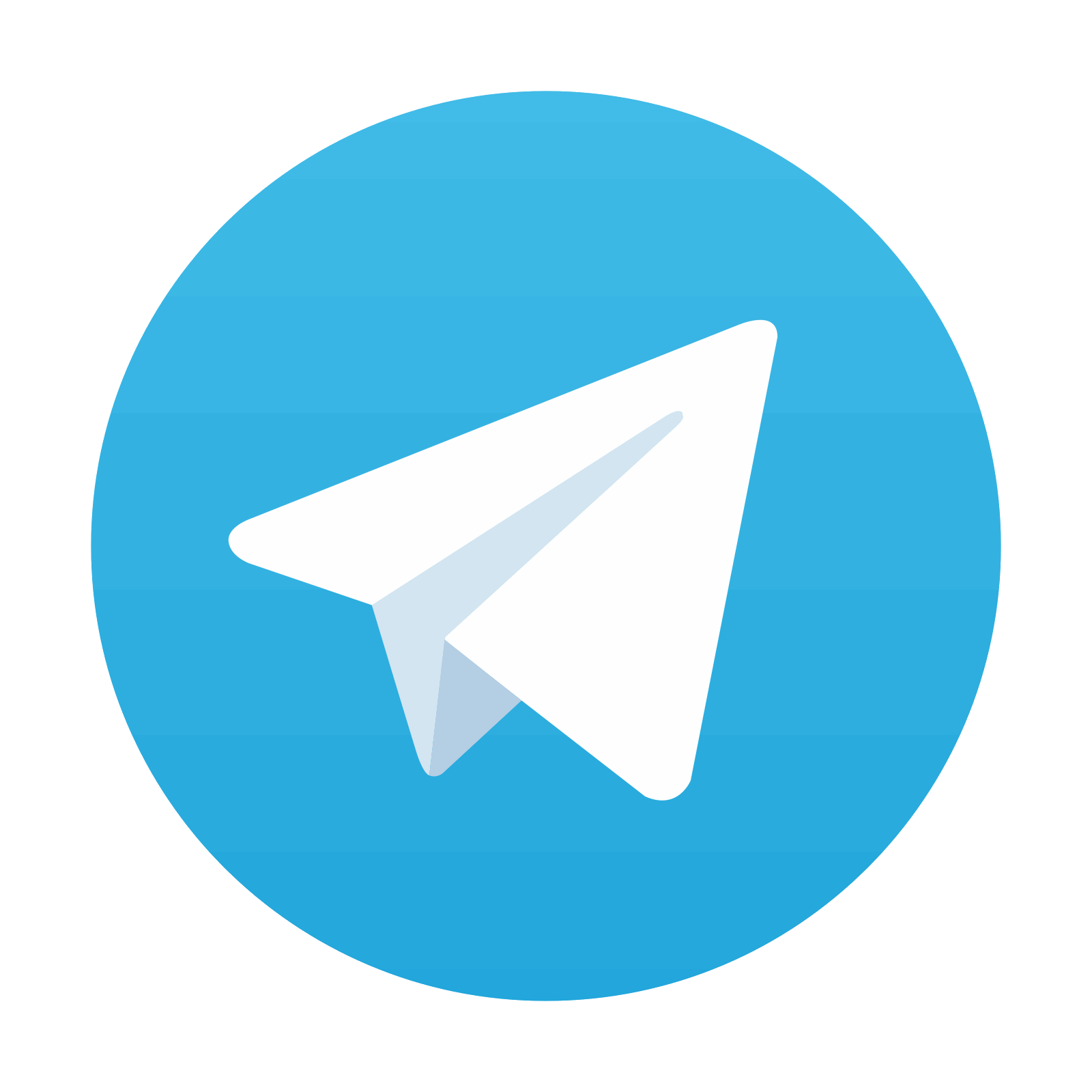
Stay updated, free articles. Join our Telegram channel

Full access? Get Clinical Tree
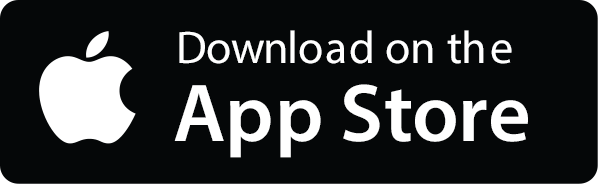
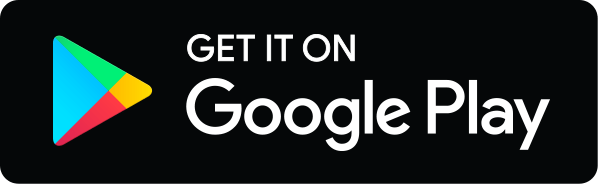
