Abstract
Fetal and neonatal alloimmune thrombocytopenia (AIT) is the most common cause of severe thrombocytopenia in fetuses and neonates [1]. Maternal IgG alloantibodies against paternally derived fetal platelet antigens cross the placenta early in pregnancy and commonly result in severe thrombocytopenia. While the reported incidence varies somewhat with the assigned threshold of thrombocytopenia (50, 100, or 150 × 109/L), in most unselected populations AIT affects 1 in 1,000 live births. Table 14.1 displays the studies of AIT in unselected populations, systematically screened. In its severe form, AIT has the potential for significant morbidity (including intracranial hemorrhage in utero) and mortality. In milder forms, there are either antibodies with no thrombocytopenia, or mild to moderate thrombocytopenia, which is identified only by a complete blood count obtained for another indication or in a screening study. While there have been extensive efforts made in the diagnosis and characterization of the disease, strategies for early detection and intervention remain controversial.
Fetal and neonatal alloimmune thrombocytopenia (AIT) is the most common cause of severe thrombocytopenia in fetuses and neonates [1]. Maternal IgG alloantibodies against paternally derived fetal platelet antigens cross the placenta early in pregnancy and commonly result in severe thrombocytopenia. While the reported incidence varies somewhat with the assigned threshold of thrombocytopenia (50, 100, or 150 × 109/L), in most unselected populations, AIT affects 1 in 1,000 live births. Table 14.1 displays the studies of AIT in unselected populations, systematically screened. In its severe form, AIT has the potential for significant morbidity (including intracranial hemorrhage in utero) and mortality. In milder forms, there are either antibodies with no thrombocytopenia, or mild to moderate thrombocytopenia, that are identified only by a complete blood count obtained for another indication or in a screening study. While there have been extensive efforts made in the diagnosis and characterization of the disease, strategies for early detection and intervention remain controversial.
Table 14.1 Incidence of neonatal thrombocytopenia
Population and number screened | Incidence of AIT | Reference |
---|---|---|
Pregnant women | ||
|
|
Neonates | ||
|
Notes: Unless otherwise specified, neonatal thrombocytopenia is defined as a cord blood platelet count
less than 150 x 109/L
* Platelet count <50 × 109/L
** Platelet count <100 × 109/L
Pathogenesis
There are three requisite components of the pathogenesis of AIT. First, there must be an incompatibility between maternal and fetal “platelet-specific” antigens, which are inherited from the father. The second requirement, alloimmunization, is a maternal humoral immune response specific to these “foreign” fetal platelet antigens. Finally, maternal antiplatelet IgG alloantibodies must cross the placenta, bind to fetal platelet antigens, and cause fetal platelet destruction with resultant thrombocytopenia. Recent work suggests that antibodies to fetal platelets may also react with fetal megakaryocytes making an important contribution to fetal thrombocytopenia. The term alloimmunization, therefore, implies that there is a parental antigen incompatibility, and that maternal antibodies are produced and are specific to the paternally inherited (and foreign to the mother) fetal platelet antigen. The predictability of this process, and its ability to cause fetal or neonatal thrombocytopenia, is well characterized serologically but perplexing in a number of areas, and warrants careful discussion.
Human Platelet-Specific Antigens
Platelets share several antigen systems with other cell types, including the HLA class I antigens, and the ABO blood group antigens [2, 3]. However, these two antigen systems have never been unequivocally implicated in AIT. The existence of AIT secondary to an HLA antigen mismatch remains controversial, although there are anecdotal reports purporting to illustrate such cases. Rather, AIT is caused by antibodies to platelet-specific antigens, which represent epitopes of the platelet surface glycoproteins. Currently there are at least 33 human platelet-specific antigens listed by the Working Party on Platelet Serology of the International Society of Blood Transfusion (ISBT), each with a different serologic frequency and ethnic distribution [2, 4–6]. In 1990, the ISBT Working Party on Platelet Serology formulated nomenclature for the human platelet antigen system, as an attempt to standardize an otherwise confusing system of antigen names and descriptions. Platelet-specific antigens are designated HPA for human platelet antigen. They are numbered in the order of their original description, and the alleles labeled alphabetically in the order of their serologic frequency [2, 4, 7]. DNA sequencing has permitted the genotyping of the specific amino acid changes associated with each allele. This genotyping system provides an additional nomenclature for describing human platelet-specific antigens. Each HPA type is biallelic, and autosomal co-dominant, differing in only one amino acid. It is important to note that there is overlap of certain alleles, as discussed by Newman [8], and that the naming of a certain allele implies an understanding of the molecular identity of that glycoprotein although knowledge of the full sequence is typically lacking. Table 14.2 contains the HPA type, the glycoprotein on which it is located, the previous antigen designations, the associated amino acid changes for each allele, and the frequency of each antigen in selected populations. This information is maintained and updated in an internet database available online at www.ebi.ac.uk/ipd/hpa/table1.html [9,10].
HPA name | Other names | Glycoprotein | DNA allele (amino acid change) | Gene frequency (Caucasian) | Serologic frequency | |
---|---|---|---|---|---|---|
White | Japanese | |||||
HPA-1a | Zwa, PLA1 | GPIIIa | Leu33 | 0.85 | 97.90% | 99.90% |
HPA-1b | Zwb, PLA2 | GPIIIa | Pro33 | 0.15 | 26.50% | 3.70% |
HPA-2a | Kob | GPIb | Thr145 | 0.93 | 99.30% | NT* |
HPA-2b | Koa, Siba | GPIb | Met145 | 0.07 | 14.60% | 35.40% |
HPA-3a | Baka, Luka | GPIIb | Ile843 | 0.61 | 87.70% | 78.90% |
HPA-3b | Bakb | GPIIb | Ser843 | 0.39 | 64.10% | NT |
HPA-4a | Pena, Yukb | GPIIIa | Arg143 | 1.00 | 99.90% | 99.90% |
HPA-4b | Penb, Yuka | GPIIIa | Gln143 | <0.01 | 0.20% | 1.70% |
HPA-5a | Brb, Zavb | GPIa | Glu505 | 0.89 | 99.20% | NT |
HPA-5b | Bra, Zava, Hca | GPIa | Lys505 | 0.11 | 20.60% | NT |
HPA-6bw** | Caa, Tua | GPIIIa | Arg489Gln | 0.85 | ||
HPA-7bw | Moa | GPIIIa | Pro407Ala | 0.85 | <1 | |
HPA-8bw | Sra | GPIIIa | Arg636Cys | 0.85 | ||
HPA-9bw | Maxa | GPIIb | Met837Val | |||
HPA-10bw | Laa | GPIIIb | Gln62Arg | |||
HPA-11bw | Groa | GPIIIa | His633Arg | |||
HPA-12bw | Iya | GPIb | Glu15Gly | |||
HPA-13bw | Sita | GpIa | Met799Thr | |||
HPA14bw | Oea | GPIIIa | Lys611del | |||
HPA-15a | Govb | CD109 | Ser682 | 0.5 | ||
HPA-15b | Gova | CD109 | Tyr682 | 0.5 | ||
HPA-16bw | Duva | GPIIIa | ThrT140Ile | |||
HPA-17bw | Vaa | GPIIb/IIIa | Thr195Met | |||
HPA-18bw | Caba | GPIa | Gln716His | |||
HPA-19bw | Sta | GPIIIa | Lys137Gln | |||
HPA-20bw | Kno | GPIIb | Thr619Met | |||
HPA-21bw | Nos | GPIIIa | Glu628Lys | |||
HPA-22bw | Sey | GPIIb | Lys164Thr | |||
HPA-23bw | Hug | GPIIIa | Arg622Trp | |||
HPA-24bw | Cab2a+ | GPIIb | Ser472Asn | |||
HPA-25bw | Swia | GPIa | Thr1087Met | |||
HPA-26bw | Seca | GPIIIa | Lys580Asn | |||
HPA-27bw | Cab3a+ | GPIIb | Leu841Met | |||
HPA-28bw | War | GPIIb | Val740Leu | |||
HPA-29bw | Khab | GPIIIa | Thr7Met |
Notes: *NT = Not Tested
** “w” indicates antigens for which an alloantibody against only one allele is identified
As discussed above, AIT has been reported after alloimmunization to numerous human platelet antigens (see Tables 14.3 and 14.4). In almost all large series, HPA1a incompatibility is not only the most common incompatibility resulting in AIT [11–19], but also an incompatibility that results in the most severe fetal and neonatal thrombocytopenia. Other severe incompatibilities result from HPA 3a and b incompatibility as well as that of HPA-9b. Studies suggest that HPA-5b incompatibility may be nearly as common as HPA-1a incompatibility, but severe thrombocytopenia after HPA-5b alloimmunization is rare [20–22]. HPA-4 incompatibility, as well as those of HPA-6b and HPA-21b, are more common in East and South Asian populations. Of note, the initial description of a new human platelet antigen is frequently prompted by a severely affected patient with significant morbidity. Therefore, most non-HPA-1a incompatibilities have severe index cases, but incidences appear to be very low. Despite numerous reports of new, and potentially severe or frequent incompatibilities, it is clear that HPA-1a incompatibility remains by far the most common cause of severe AIT in Caucasian populations. HPA-9b and HPA-3a and 3b are the next most common severe causes of neonatal alloimmune thrombocytopenia (NAIT).
Number of patients | Entry criteria | Bleeding symptoms | Antigen incompatibility | Reference |
---|---|---|---|---|
88 | Thrombocytopenic* infants with AIT |
| HPA-1a | [12] |
24 | Infants with AIT |
| HPA-1a | [15] |
46 | Thrombocytopenic infants with AIT |
|
| [14] |
Notes:
* Thrombocytopenia defined as a platelet count less than 100 x 109/L
** Died at 6 months of age from complications of the ICH
Table 14.4 Less common antigen incompatibilities in AIT
HPA type | Reference | Patients reported | Platelet nadir (× 109 cells/L) | Hemorrhagic sequelae | Maternal HLA type |
---|---|---|---|---|---|
HPA-1b | 43, 146–150 | 6 total |
|
| No consistent association |
HPA-2b | 47 | 3* | Mild | None | |
HPA-3a | 80, 151 |
| 13 |
| None reported |
HPA-3b | 47 | 4* | Mild <20 | None | |
HPA-4b | 77 | 2 (sibs) | 6–12 |
| No consistent association |
HPA-5b | 12, 13, 16, 20, 21, 45, 47, 58, 152 | 39 total |
|
|
|
HPA-6b | 45, 47, 153, 154 | 4 total | Mild |
|
Notes: * AIT suspected due to antigen incompatibility and thrombocytopenia, but there was no antibody detected.
**ICH = intracranial hemorrhage
Alloimmunization
Fetal platelet antigens may be expressed as early as 16 weeks’ gestation [23], and alloantibody formation with fetal platelet destruction may occur as early as 16 to 20 weeks of gestation [24, 25]. Alloimmunization is reported in up to 50% of severely thrombocytopenic newborns. This may be due in part to beta 3 integrin (GPIIIa) expression on syncytiotrophoblasts, although this has not been confirmed [26]. Antibodies have not been detected in primiparous women prior to 17 weeks’ gestation [17]. In 46 sensitized HPA-1a negative women, Williamson et.al. reported that alloantibodies were detected before the 20th week of gestation in 59%, between 21 and 34 weeks in 17%, between 33 weeks and term in 6.5%, and on postnatal testing only in 6.5% [17].
Van Loghem et al. initially reported that 97% of North American and European/white express HPA-1a [27]. The Hardy–Weinberg law predicts the allelic frequency to be 0.83 for HPA-1a and 0.17 for HPA-1b. Therefore, in populations of northern European descent, the genotypic distribution is calculated to be 68.9% homozygosity for HPA-1a, 2.9% homozygosity for HPA-1b, and 28.2% heterozygosity (HPA-1a/HPA-1b) [28, 29]. The frequency of the HPA-1b genotype has been confirmed in several large population studies [17, 18, 30, 31]. Both the predicted and actual frequency of mothers at risk for HPA-1a incompatibility, by virtue of being homozygous for HPA-1b, is 2%–3%. The HPA-1b homozygous mother will be susceptible to alloimmunization in all cases if her partner is homozygous for HPA-1a (68.9%), or in half the offspring of a heterozygous partner (14.1%). Based on these gene frequencies, alloimmune thrombocytopenia as a result of HPA-1a incompatibility may develop in 1 of 42 pregnancies[29]. The actual incidence, as stated above, is 1 in 1,000 to 1 in 2,000. In fact, several prospective studies have shown that only 4%–14% of mothers homozygous for HPA-1b will produce anti-HPA-1a antibodies [11, 17, 31, 32].
The discrepancy between the expected and actual incidence of AIT can be explained, at least in part, by the efficiency of antigen presentation by different MHC subtypes. In 1981, Reznikoff-Etievant et al. reported that the immune response to HPA-1a is associated with the maternal HLA type B8DR3 [33].a Subsequently, several authors confirmed a strong association between the production of anti-HPA-1a antibodies and the HLADR3 locus [32, 34–39]. de Waal et al. later linked it to the supertypic HLA DRB3*0101 allele [40].
The HLA DRB3*0101 protein has a binding affinity for a peptide containing the Leu33 substitution on the integrin β3 (HPA-1a), but not for the corresponding peptide containing Pro33 (HPA-1b), demonstrating the importance of recognition of fetal peptides expressed on the β3 integrin by the maternal class II MHC proteins for antigen presentation [41, 42]. This genetic maternal restriction on the immune response to foreign fetal antigens accounts, in large part, for the relatively low number of HPA-1a negative mothers who develop alloantibodies against their HPA-1a positive fetus. The other not-well- clarified factor is maternal exposure to fetal platelets.
Williamson et al. studied HPA type, antibody production, and maternal HLA type in more than 24,000 consecutive pregnancies in Cambridge and East Anglia, England [17]. Among the 2.5% of women homozygous for HPA-1b, anti-HPA-1a antibodies were detected in 12%. All but one of the antibody producers was HLA DRB3*0101 positive, while the overall frequency of the allele was 31.9%. The presence of the HLA DRB3*0101 phenotype did not predict alloimmunization (positive predictive value of 35%), but the absence of the HLA DRB3*0101 precluded alloimmunization with very few exceptions (negative predictive value 99.6%). Finally, only a minority of antibody-formers will have severely thrombocytopenic fetuses/newborns.
Although not as well studied, alloantibody formation in cases of HPA-5b incompatibility has been associated with maternal HLA DRw6 positivity [20, 21, 43]. Other HPA incompatibilities resulting in alloimmunization may have similar HLA associations [44], but the low incidence of AIT due to these antigens makes study difficult.
Alloantibody Mediated Thrombocytopenia
Maternal alloimmunization to fetal platelet antigens is most likely necessary and usually, but not always, sufficient to induce immune thrombocytopenia in the fetus or newborn. There are a number of reported cases of high titer maternal antiplatelet alloantibodies in infants with normal platelet counts [17, 21, 30, 31, 45]. Maternal alloimmunization without neonatal thrombocytopenia may be as high as 32% [45]. In addition to maternal HLA MHC class II modulation of alloimmunization, there appears to be another regulatory step in AIT. The process of antibody-mediated platelet destruction may be inhibited by unknown factors specific to certain maternal–fetal pairs. One possibility is a so-called “blocking” antibody that might be anti-HLA; this has not been confirmed. Such an antibody could be directed against FcγR and thus block platelet destruction.
Conversely, there are reports of maternal–fetal HPA incompatibility with neonatal thrombocytopenia (presumed AIT), with no detectable maternal alloantibody [12, 16, 19, 45–47]. Newer techniques of antibody measurement have substantially improved detection but not entirely resolved this issue[48].
Careful and extensive study of AIT is largely limited to anti-HPA-1a antibodies as discussed above. The role of alloantibodies to other fetal platelet antigens is less clear. There are reports of suspected AIT with anti-HLA antibodies [12, 49–51, 52–54], anti-blood group antibodies [12, 55, 56], and antiplatelet–glycoprotein-specific antibodies [47, 57]. Of these less common causes of AIT, anti-HLA antibodies are perhaps the most considered but the least certain. King et. al. found no significant relationship between the formation of anti-HLA antibodies and neonatal thrombocytopenia [51]. It is possible that the presence of anti-HLA antibodies in infants with thrombocytopenia is coincidental, as anti-HLA antibodies will be absorbed by HLA antigens in the placenta or on WBCs, thus minimizing their effect on fetal platelets. At best the role of anti-HLA alloantibodies is unclear but, when present, they appear to have little effect on neonatal platelet counts [50, 51]. It is, of course, impossible to exclude that they might have a thrombocytopenic effect in individual cases. Resolution could be achieved by collecting sufficient platelets from cord blood to identify specificity of the antibodies in the eluate, but this technically would require a tour de force.
Clearly, the details of the genetic and immunologic controls governing the process of alloimmunization and its outcome have yet to be completely described. Our ability to predict the occurrence of alloimmunization in cases of fetomaternal platelet antigen incompatibility is limited, as is our ability to predict the presence and severity of thrombocytopenia after alloimmunization occurs.
Clinical Presentation
For newborns with unsuspected AIT, evaluation will commonly be initiated by the pediatrician or neonatologist after perinatal identification of petechiae or purpura, or by the incidental finding of thrombocytopenia on a complete blood count obtained for a different indication, e.g., suspected infection. When defined as either a cord blood or neonatal platelet count of <100–150 × 109/L, neonatal thrombocytopenia is reported in 0.5%–0.9% of newborns, with severe thrombocytopenia (platelet count less than 50 × 109/L) in 0.14%–0.24% [1, 19, 31, 45, 47, 58] (See Table 14.1). Immune-mediated thrombocytopenia may account for as much as 30% of neonatal thrombocytopenias, occurring in 0.3% of all newborns [45]. A small additional subset of asymptomatic patients will be identified because of a sibling with AIT.
The frequency of hemorrhagic symptoms in AIT is likely overestimated in clinical studies not based on population screening, considering that a substantial proportion of patients with AIT identified by screening will not have severe thrombocytopenia and go undetected [17, 59]. For those patients with clinically significant disease, or with neonatal platelet counts less than 50 × 109/L, the incidence of minor hemorrhagic diatheses (petechiae, ecchymoses, or hematomas) is as high as 80% [12, 15, 19]. The most frequent bleeding symptoms for AIT with clinically significant disease are listed in Tables 14.3 and 14.4.
AIT identified through routine screening is more likely to follow a benign course. In two large maternal screening studies of AIT, 65% of infants with persistent in utero exposure to maternal anti-HPA-1a alloantibodies had mild or no thrombocytopenia [17, 59]. While petechiae and ecchymoses are important markers of clinically significant disease, they occur in a minority of screened patients with AIT. On the other hand, a family history of fetal and neonatal AIT (FNAIT) without perinatal hemorrhagic symptoms should not prevent the consideration of hemorrhagic AIT in subsequent pregnancies. Additionally, some thrombocytopenic infants with proven AIT will have other perinatal problems (e.g. infection, poor feeding, low birth weight, cardiac problems, or respiratory problems), which may confuse the etiology of the thrombocytopenia [16, 60].
Neonatal platelet counts can vary from normal to less than 10 × 109/L. When looking at all infants with AIT, as many as 35% may have platelet counts less than 50 × 109/L [17]. However, in series of clinically affected AIT cases identified because of bleeding symptoms, 90% will have platelet counts <50 × 109/L and 50% with have counts <20 × 109/L. While this represents a minority of all cases of AIT, when present and when identified clinically rather than by screening, thrombocytopenia in AIT is usually severe [12, 16, 17]. In fact, AIT is the most common cause of severe thrombocytopenia and intracranial hemorrhage in term newborns in all studies to date [1, 16, 19].
Intracranial Hemorrhage
The mortality rate with AIT has been reported as high as 15%, but may be less even in clinically recognized cases. Almost all deaths are associated with intracranial hemorrhage (ICH) [12, 30]. ICH occurs in 10%–20% of affected neonates [12, 30, 61]. In a series of almost 50,000 unselected term neonates, the incidence of ICH was 1 in 1,500 live births. Twenty-five percent occurred in infants with AIT, making it the most common cause of severe ICH in term newborns [62, 63]. Intraventricular hemorrhages are most common in neonates, especially premature ones, but unifocal, multifocal, and large parenchymal hemorrhages are more commonly reported in AIT [12, 64–67]. The vascular distribution of ICH in alloimmune thrombocytopenia may be expected from the fact that normal hemostasis is maintained, in part, through interaction between platelets and the vascular endothelium. It is possible that glycoprotein-bound antiplatelet antibodies interfere with the ability of platelets to support the vascular endothelium [68]. It is also possible that minimal trauma can lead to devastating vascular hemorrhage in the developing thrombocytopenic fetus or neonate. Platelets also support normal angiogenesis, and defective angiogenesis leading to intracranial hemorrhage has been demonstrated in an animal model of anti-HPA-1a antibodies in NAIT [69].
More than 50% of all reported intracranial hemorrhages in AIT will occur antenatally [12, 30, 70]. Severe thrombocytopenia (platelet count <20 × 109/L) was present at the time of initial fetal sampling by 24 weeks of gestation in 50% of affected fetuses; thrombocytopenia has been reported as early as 13 to 16 weeks’ gestation [13, 24, 25, 71]. In utero hemorrhages may present at any point after 18 weeks of gestation or in the newborn period, presenting with fetal distress or demise [72, 73], fetal or neonatal hydrocephalus [12, 73, 74], encephalomalacia, intracranial cysts [64, 65, 75], an abnormal neurological exam, or poor feeding [15, 62, 70, 75, 76]. ICH has been reported in association with almost all antigen incompatibilities [77, 78], but it is most commonly associated with HPA-1a incompatibility [12, 15, 70].
In vitro binding of autoantibodies to GPIIb/IIIa may rarely result in an acquired disorder in platelet function [68]. In patients homozygous for HPA-1a, alloantibodies to HPA-1a will completely eliminate platelet aggregation to all platelet agonists except ristocetin, but the effect is much less in heterozygous HPA-1a expression (as in all fetuses with AIT). The pathophysiology mediating this effect is not entirely clear. Glycoprotein IIb/IIIa is the most abundant glycoprotein on the platelet surface, and it is the site of fibrinogen binding. However, antibodies to HPA-1a do not appear to bind near enough to the fibrinogen-binding site to cause a steric hindrance [79]. The vascular distribution of some in utero hemorrhages has led to additional speculation about the possible role of antibodies to endothelial GPIIIa in the pathogenesis of intracranial hemorrhages [69]. However, if there is a seemingly equivalent rate of ICH in HPA-3b (GPIIb) incompatibility, this suggests that this may not be a prominent factor since GPIIb is not expressed on endothelial cells [80]. In summary, platelet counts less than 20 × 109/L in AIT are permissive but not sufficient for ICH. Anti HPA-1a antibodies do not likely cause substantial platelet dysfunction in the HPA-1a heterozygous fetus. However, anti-HPA-1a antibodies may negatively affect angiogenesis and contribute to ICH.
Predictors of Disease
A goal of recent screening protocols has been to define prognostic factors that may identify those patients at risk for ICH [17, 18, 81, 82]. As discussed earlier, the presence of maternal alloantibody does not predict thrombocytopenia. However, studies have suggested that high (greater than 1:32) third trimester maternal antibody titers [17, 18], and high titers of the IgG3 subclass [82], may predict severe thrombocytopenia. For HPA-5b incompatibilities, neither antibody titer nor subclass appears to predict disease [22].
More than 80% of subsequent siblings of AIT patients will be affected. Mildly affected or undiagnosed first-born children may have severely affected siblings. Initial fetal platelet counts of less than 20 × 109/L are reported in 50% of siblings of affected children. Forty-five percent of second siblings may have platelet counts of less than 20 × 109/L by 24 weeks’ gestation. However, the only significant predictor of severe disease in subsequent affected siblings is antenatal ICH in the previous sibling [63, 71]. Recent studies have focused on the predictive value of HLA, e.g., combinations of DRB30101 and specific DRB4 alleles, but this is still preliminary. Other approaches have been to study the association of anti-HPA-1a antibody effect on in vitro megakaryocytopoiesis and neonatal platelet counts, and the role of anti-HPA-1a antibody fucosylation in induction of ICH.
Diagnosis
The diagnosis of AIT in a thrombocytopenic infant or fetus requires several laboratory observations. There must be HPA incompatibility between mother and child (usually defined via paternal typing). Although not always identifiable, even with the most sensitive techniques, a firm diagnosis of AIT requires the identification of maternal antiplatelet alloantibodies. These antibodies should bind to paternal, but not maternal platelets, and they should be specific to the antigen incompatibility in question, including specificity for the glycoprotein on which the incompatible epitope is located.
Antigen Testing
Few facilities can do both DNA-based and serologic testing for the complete range of the most frequently encountered HPA antigens. With the development of oligonucleotide probes and the refinement of PCR techniques, it is possible to obtain platelet antigen typing on amniocytes and fetal leukocytes [83–85]. Techniques are also now available for platelet antigen genotyping from dried blood spots on cards to aide in rapid perinatal diagnosis [86]. Currently, free fetal DNA in the maternal circulation can be identified and HPA-1a/b genotype determined from maternal blood alone.
Several ELISA-based [87, 88] and fluorescence-based [89] techniques have been developed for rapid antigen typing on large numbers of samples. ELISA-based kits are also available to screen for common HPA antigens, and to allow accurate testing of the common antigens at smaller centers [88].
Alloantibody Testing
Early antibody testing was performed with the platelet suspension immunofluorescent test (PSIFT). The PSIFT technique detects antiplatelet antibodies bound to the surface of platelets, but is not specific for anti-HPA alloantibodies [90, 91]. In 1987, Kiefel et al. reported accurate alloantibody detection with an enzyme immunoassay, the monoclonal antibody immobilization of platelet glycoprotein assay (MAIPA) [92]. This technique, and subsequent refinements, now allows for the identification and quantification of glycoprotein-specific alloantibodies [92–94]. MAIPA can be both utilized during pregnancy to monitor antibody levels, and possibly adapted for large scale screening programs [95]. The MACE is a similar technique. Other techniques using surface plasmon resonance and expressing specific antigens on fibroblasts show promise for large scale screening programs [5, 96, 97].
Genotyping
DNA-based typing is now widely used to perform antigen typing [98]. One method uses polymerase chain reaction (PCR) to amplify genomic DNA encoding specific antigens using allele-specific primers, followed by electrophoresis and band visualization. Another method relies on fluorescent, allele-specific DNA probes that are quenched in solution but become fluorescent when hybridized to DNA from patient samples.
Who to Test
When evaluating the thrombocytopenic newborn, it is important to carefully consider the diagnosis of AIT; if nothing else because of the high rate of ICH with which it is associated and the high recurrence rate. AIT is the most common diagnosis resulting in severe neonatal thrombocytopenia, is likely to result in more severe thrombocytopenia in the mother’s next pregnancy, and can also affect her female siblings and cousins. We believe that all infants with platelet counts less than 50 × 109/L should be tested for AIT and managed accordingly. Even in patients with mild thrombocytopenia, it is important to identify those with AIT, because of the implication for subsequent offspring, who may be more severely affected [99]. In general, any thrombocytopenic infant, even with a platelet count greater than 50 × 109/L, without evidence of asphyxia or sepsis, is deserving of evaluation. A high index of suspicion must be maintained even in the setting of other medical problems, as up to 30%, infants with AIT will also have other medical problems that confuse the diagnosis of AIT. Therefore, the diagnosis of other newborn diseases, regardless of whether or not they are independent causes of thrombocytopenia, should not prevent an evaluation for AIT. This is especially true with severe thrombocytopenia or ICH, since both are strongly associated with AIT. In premature infants, where an ICH is likely due to an underdeveloped germinal matrix, AIT should be considered if the ICH is associated with thrombocytopenia, or if there is a parenchymal bleed. Finally, any familial or transient neonatal thrombocytopenia should also be evaluated [99, 100].
Screening Programs
There is no consensus on obtaining routine neonatal platelet counts, or whether to perform prenatal maternal platelet antigen typing. While numerous series have performed neonatal and maternal screening, only Norway currently routinely screens all pregnancies for AIT. Alloimmune thrombocytopenia is a significant cause of prenatal and perinatal morbidity and mortality, the pathogenesis is relatively well understood, and early intervention can prevent adverse outcomes. Unfortunately, we lack specific biological markers of severe disease to justify treating if no family history is available (previous affected fetus), either at all or more aggressively knowing that the fetus will be severely affected.
The financial and technical burden of screening all mothers for HPA-1a1b would be difficult to deal with unless prevention was possible. Testing would have to be based on the ethnic distribution of the most likely antigen incompatibility, and the severity of the associated thrombocytopenia. For European/white populations this would require testing for the HPA-1a antigen. Such a program would miss incompatibilities among the less common human platelet antigens, some of which can lead to severe disease, but would not likely miss many cases of neonatal thrombocytopenia.
If a screening program were to identify a woman with the HPA-1b antigen, what would be the next step to determine the need for treatment? Testing for both anti-HPA-1a antibody and HLA-typing for DRB*30101 has been suggested. High anti-HPA-1a antibody titers may predict significant thrombocytopenia and certain studies suggest that low level or absent antibody may be predictive of less severe thrombocytopenia. In most cases of AIT, alloimmunization is eventually detected but it may require multiple antibody assays throughout the pregnancy and postnatal period. Additionally, there are at least three reported cases (including those in unpublished communications) of alloimmunization to HPA-1a in mothers that do not have the HLA DRB*30101 subtype, although these are rare. A recent publication suggests that they may be more common than we thought, but that they are milder than those in HPA-1b1b mothers with the DRB3 haplotype.
Screening programs have the potential to prevent the devastating or fatal hemorrhagic complications of AIT. However, there is almost no circumstance in which fetal blood samplings would be justified from a screening program. If we assume that there is a human-platelet antigen incompatibility in 1 in 40 pregnancies, and that 2% of these will have detectable alloimmunization [17, 30–32], then 1 in 2,000 pregnancies will result in alloimmunization. Only 35% of infants exposed to antiplatelet alloantibodies will develop moderate to severe thrombocytopenia [17], and only 10%–20% of these neonates with suffer an intracranial haemorrhage [12, 15, 61].
Two large, multicentered studies completed in northern Europe have explored these issues [59, 101]. Both studies exclusively screened for anti-HPA-1a antibodies, and neither study used fetal blood sampling or antenatal treatment. In both studies the majority of maternal–fetal pairs with anti-HPA-1a antibodies did not result in AIT. The Norway study used preterm caesarian-section delivery to reduce the risk of ICH, while the United Kingdom study did not report any intervention. In the United Kingdom study it was estimated that the cost to diagnose one case of AIT would be US$ 99,000, while preventing one episode of ICH would cost US$ 1.9 million.
Management
The goal of diagnosing and treating AIT is to prevent ICH, either antenatal or perinatal, and prevent the severe morbidity or mortality associated with ICH in this period. For those patients diagnosed in the neonatal period, early diagnosis and intervention may prevent or at least stabilize ICH [70]. Some cases will be diagnosed antenatally due to diagnosis of AIT in a first degree relative, or due to an in-utero ICH diagnosed on screening ultrasound. Effective management of the affected fetus can dramatically reduce the risk of ICH in those with history of previously affected pregnancies. While the rate of ICH may be small, its consequences may be devastating and thus aggressive evaluation and treatment measures are warranted.
Antenatal Management
Several factors are important to consider when developing antenatal therapy plans for fetuses suspected of having AIT. The natural history of fetal NAIT must be considered, with the potential for severe thrombocytopenia, and the increasing risk of ICH with declining platelet counts. The efficacy, morbidity, and mortality associated with the available therapies and procedures must also be considered. First-born children are rarely diagnosed before birth, unless part of a maternal antigen typing program. Thus, the most experience (and data) on management of AIT is derived from treatment of siblings of previously affected fetuses.
Severity of Disease
There are no reliable predictors of severe fetal thrombocytopenia other than an antenatal ICH in an older sibling. However, subsequent affected infants are likely to have at least as severe thrombocytopenia as their older siblings. In one study, up to 40% of pre-treatment fetal platelet counts were lower than the nadir of the previously affected infant. When measured, fetal platelet counts were noted to decrease, sometimes rapidly, even if initially adequate. Conversely, there have been no reported cases of spontaneous fetal platelet count increases in AIT. If fetal thrombocytopenia in previous pregnancies was not severe, the outcome in subsequent pregnancies is impossible to predict.
ICH
Neonatal and fetal ICH is very rare at platelet counts greater than 20 × 109/L. However, the risk of ICH at a given platelet count after vaginal birth and after cesarean section has not been studied in randomized controlled trials in AIT. Several early reports of ICH in thrombocytopenic infants after vaginal delivery prompted authors to advocate for scheduled “elective” cesarean section in severely thrombocytopenic infants [102, 103]. However, it is not clear if vaginal delivery is an independent risk factor for ICH in thrombocytopenic neonates since most ICHs occur antepartum [104, 105]. The safety of cesarean section vs. vaginal delivery in AIT has also not been well studied. Although it may seem that cesarean section would be safer, antenatal therapy may increase the fetal platelet count to greater than 50 × 109/L, making vaginal birth an option.
Therapeutic Procedures
In the past, monitoring the efficacy of therapeutic interventions in fetuses with AIT required fetal blood sampling using cordocentesis. There is a reported fetal loss rate of 0.2% to 7.2%, depending on the technique (free-hand technique, fixed-needle technique, or combined technique), operator experience, and underlying fetal disease [82, 106–109]. In otherwise healthy but thrombocytopenic fetuses, the risk of fetal loss is approximately 1%–6%[110, 111]. The data is mixed on whether fetal loss is related to fetal platelet count, with some authors finding a correlation between low fetal platelet counts and fetal demise[111], and others not [82]; our data is in favor of a correlation. Therefore transfusion of maternal or other matched antigen negative platelets was the standard of care, to be given in conjunction with fetal blood sampling even prior to availability of the fetal platelet count. There is no longer an expectation that fetal sampling should be a routine part of care except possibly very late in pregnancy to decide when and how to deliver.
Efficacy of Selected Therapies
Intrauterine Platelet Transfusions
Shortly following the description of the cordocentesis procedure, Daffos et al. described the use of washed maternal platelets injected into the umbilical vein of an infant with AIT [112]. Subsequent publications reported the efficacy of prophylactic HPA-1a negative platelet transfusions in maintaining fetal platelet counts and avoiding hemorrhagic complications [25, 113–115]. Unfortunately, transfused platelets have a short half-life and repeat transfusions are required every 5 to 7 days to maintain an adequate platelet count (>20 × 109/μl) in a fetus with severe AIT. Repeated weekly fetal blood samplings for the purpose of platelet transfusions carry a greatly increased risk of fetal loss or distress and therefore this approach has almost entirely been abandoned.
Intravenous Immunoglobulin and Corticosteroids
While corticosteroids have shown limited benefit as single agents in AIT [116], with some efficacy as primary treatment in mildly affected cases, they are used primarily as adjunctive treatment with intravenous immunoglobulin (IVIG). The use of maternally administered intravenous immunoglobulin has had the greatest impact on restoration of adequate fetal platelet counts and prevention of ICH [117–121]. The mechanism of effect of IVIG is unclear, although IVIG is thought to most likely work by reducing the level of maternal anti-HPA-1a antibodies and especially by competitively inhibiting the binding of alloantibodies to the FcRn receptor [120, 122]. IVIG administered at sufficient doses will block FcRn receptors in the placenta, preventing endocytotic uptake of alloantibodies [123]; whether IVIG also affects the fetal mononuclear-phagocytic system, preventing alloantibody-mediated platelet destruction, is unknown [120, 122]. However, the same mechanism in mothers may lower the anti-HPA-1a titer in the maternal circulation. The former mechanism is indirectly supported by the observation that the levels of IgG in the maternal circulation after infusion of 1 gm/kg of IVIG are in the range that was shown to competitively inhibit the transport of 90% of anti-D in a placental perfusion model [121, 123]. Direct infusion of IVIG into the fetal circulation [124, 125] and peritoneum [25] has been reported without great success. Given the efficiency of maternal IVIG transfer across the placenta, and the theoretical benefit of FcRn receptor blockade in the placenta, it is most likely unnecessary to directly infuse IVIG into the fetus. However, data on this approach are limited. Recent data suggest that IVIG blockade of antibody transfer to the fetus may have its greatest impact in the protection of fetal megakaryocytes [126].
In 15 of the first 18 maternal–fetal pairs treated with maternally administered IVIG (1 gram/kg weekly), there was a substantial increase in fetal platelet counts. None of the fetuses or newborns suffered an ICH, even when an antenatal ICH was reported in an older sibling. Almost all of the infants had higher birth platelet counts than did their older siblings. However, in these first cases when IVIG was used in combination with 3 to 5 mg/kg per day of dexamethasone, 4 of 5 patients developed oligohydramnios [118, 120]. In 1992, Lynch et al. reported that the use of IVIG alone seemed inferior to that of IVIG and steroids [120]. Therefore, a treatment protocol with IVIG plus 1.5 mg/kg per day of dexamethasone was piloted. At this lower dose of dexamethasone, there was no reported oligohydramnios [100, 120]. However, a randomized trial failed to demonstrate benefit of combined IVIG and dexamethasone (1.5 mg/kg) over IVIG alone [100].
There are a number of reports of refractory fetal thrombocytopenia despite administration of maternal IVIG [25, 120, 127–129]. The addition of high dose prednisone (60 mg/day) to weekly IVIG tended to be effective in patients who do not respond to IVIG alone. This dose of prednisone in combination with IVIG did not cause oligohydramnios [100].
Weekly antenatal IVIG appears to be an effective therapy in preventing in utero and perinatal ICH. It is important that the intensity of therapy be matched to the perceived risk to the fetus so that there is no need to perform a fetal blood sampling since virtually all fetuses will be successfully treated. This means over-treating some fetuses to be sure to successfully treat almost all fetuses. Again, the risk of fetal blood sampling in potentially severe thrombocytopenia is too great to recommend its routine use.
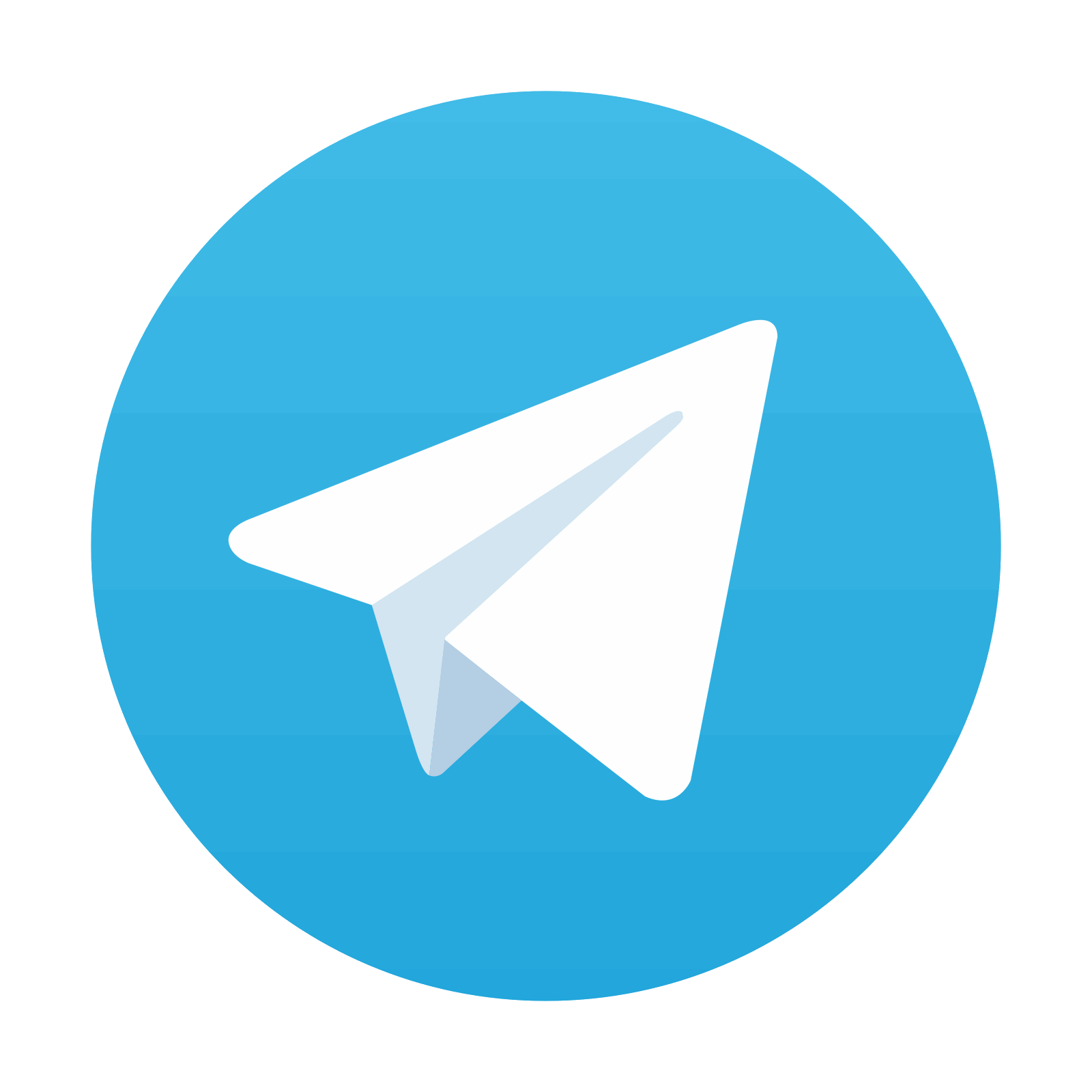
Stay updated, free articles. Join our Telegram channel

Full access? Get Clinical Tree
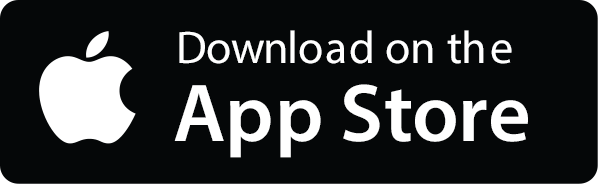
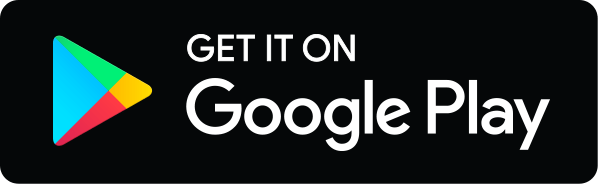
