Abstract
Anemia of prematurity is a multifactorial anemia, characterized by relatively low plasma erythropoietin (EPO) levels, iatrogenic blood loss, low circulating blood volumes and insufficient erythropoiesis. This anemia has been long characterized as nutritionally insensitive, but nutrition may influence its clinical course. Anemia of prematurity is treated with erythrocyte transfusions. However, delaying umbilical cord clamping may increase initial hematocrit percentages, improve infant iron status, prevent erythrocyte transfusions, decrease necrotizing enterocolitis (NEC), and decrease intraventricular hemorrhage (IVH). Many published studies have examined the potential of therapy with recombinant human EPO or other erythropoietic stimulating agent (ESA). Although EPO therapy is associated with statistically lower number of total erythrocyte transfusions, most early transfusions are not eliminated. However, early erythropoietic EPO dosing may also decrease NEC and IVH as well as improve neurocognitive outcomes. A recent systematic review refuted previous concerns that early administration of EPO was associated with increased retinopathy of prematurity (ROP). Early high dose EPO regimens are currently being studied for neuroprotection in premature infants. Iron deficiency in EPO treatment is also of potential concern, but long-term iron status of EPO treated premature infants is similar to controls.
Introduction
Anemia of prematurity is a multifactorial anemia, characterized by relatively low plasma erythropoietin (EPO) levels, iatrogenic blood loss, low circulating blood volumes, and insufficient erythropoiesis. This anemia has been long characterized as nutritionally insensitive, but nutrition may influence its clinical course. Anemia of prematurity is treated with erythrocyte transfusions. However, delaying umbilical cord clamping may increase initial hematocrit percentages, improve infant iron status, prevent erythrocyte transfusions, decrease necrotizing enterocolitis (NEC), and decrease intraventricular hemorrhage (IVH). Many published studies have examined the potential of therapy with recombinant human EPO or other erythropoietic stimulating agent (ESA). Although EPO therapy is associated with statistically lower number of total erythrocyte transfusions, most early transfusions are not eliminated. However, early erythropoietic EPO dosing may also decrease NEC and IVH as well as improve neurocognitive outcomes. A recent systematic review refuted previous concerns that early administration of EPO was associated with increased retinopathy of prematurity (ROP). Early high dose EPO regimens are currently being studied for neuroprotection in premature infants. Iron deficiency in EPO treatment is also of potential concern, but long-term iron status of EPO treated premature infants is similar to controls.
Physiology of Anemia of Prematurity
Erythropoietin (EPO), the primary hormone regulating erythropoiesis, is measurable throughout fetal gestation [1]. In the fetus and newborn, EPO is produced primarily by the liver, which may be relatively insensitive to hypoxia, compared to kidney [1, 2]. After term birth, erythropoiesis is suppressed by markedly improved postnatal oxygen delivery, a relatively depressed plasma EPO, and a fall in hemoglobin (Hb) occurs that reaches “physiologic nadir” in the first months postpartum [3]. This response is exaggerated in premature infants [4]. The anemia of prematurity reflects not only insufficient EPO production [4], but also small circulating blood volume, hemorrhage, hemolysis, shortened red blood cell (RBC) survival, and perhaps most important is the iatrogenic blood loss, coined the “anemia of phlebotomy” (summarized by Ohls) [5]. Anemia of prematurity is traditionally described as nutritionally “insensitive” in that it does not improve with addition of iron, although iron contributes to the recovery of Hb [6, 7]. Iron status is critical, in that insufficient iron supplementation may inhibit the efficacy of EPO in prematurity [7, 8].
Therapy for Anemia of Prematurity
Minimizing Blood Loss
Blood loss impacts the clinical course of the anemia of prematurity. In a typical 800 gram birth weight premature infant, with red cell mass at birth of 27 mL, avoidance of erythrocyte transfusions depends on avoidance of blood loss [9]. Lower initial Hb and acuity are major factors in determining erythrocyte transfusion [10, 11]. In the absence or presence of EPO therapy, phlebotomy clearly correlates to erythrocyte volumes transfused [9, 10, 12–19]. This is most evident when precise transfusion criteria are employed [14]. Some centers report relatively lower phlebotomy loss on even the smallest infants [20, 21]. Inline and microsampling point of care testing minimizes blood loss in unstable infants [17, 22, 23]. Advances in perinatal care that decrease patient acuity would logically decrease blood administration [13], but erythrocyte transfusion practices vary widely [14–16]. In the neonatal intensive care unit (NICU), blood volume drawn is related to volume transfused, with phlebotomist overdraw in the blood tubes becoming significant [9, 24, 25].
Delayed Umbilical Cord Clamping
At premature delivery, administering an autologous placental transfusion by delaying umbilical cord clamping decreases number of erythrocyte transfusions [26–30]. Delayed clamping may sufficiently augment the initial red blood cell volume by 10%–15% [31, 32]. In a Cochrane Systematic Review, delayed clamping for up to 180 seconds was associated with higher hematocrit values after birth, fewer erythrocyte transfusions for anemia, fewer cases of intraventricular hemorrhage (IVH; all grades), and fewer cases of necrotizing enterocolitis (NEC) [29]. Recent review of delayed clamping in extremely preterm infants confirmed fewer transfusions and less IVH, with both blood pressure and survival higher [33]. It is not known whether delayed clamping either works as well as EPO in preventing erythrocyte transfusions or whether delayed clamping augments the efficacy of EPO in premature infants. Additionally, iron [34] contained in the red blood cell mass may improve long-term iron status and decrease iron deficiency anemia [35], but red cell breakdown results in higher peak bilirubin levels after delayed clamping [29]. Nonetheless, the American College of Obstetricians and Gynecologists and American Academy of Pediatrics recommend delaying cord clamping for premature infants when feasible [30].
Erythrocyte Transfusions
Although covered in another chapter in more detail (see Chapter 20), any review of EPO therapy should cover erythrocyte transfusions and transfusion triggers [25, 36]. Conservative erythrocyte transfusion criteria were developed as part of the clinical trials of EPO in prematurity, and these were recognized as safe and effective tools in limiting transfusions [12, 14, 37]. The desire of clinicians to limit blood donor exposure, with or without EPO therapy, has led to lower numbers of erythrocyte transfusions per infant [10, 13, 38, 39]. Recently, randomized studies examined the safety of lower Hb or hematocrit triggers [17, 40], showing no consistent adverse sequelae at hospital discharge of restrictive triggers for erythrocyte transfusion [41, 42], with conflicting findings in the two large studies [17, 40]. In follow up, one study found lower white matter volumes in toddlers with liberal transfusions [43] that adversely impacted function [43], and white matter correlated with function in females at school age [44], while the larger study found no differences in death, cerebral palsy, cognitive delay, or hearing loss in toddlers with restrictive or liberal transfusion criteria, but higher mental development index (MDI) with in post hoc analysis in liberal transfusions [41, 45].
EPO Therapy for Anemia of Prematurity
Since 1990, over 65 studies have been published reporting use of EPO or erythropoietic stimulating agents (ESAs) to prevent transfusions in prematurity. ESAs are new drugs either structurally altering EPO in order to increase half-life or are EPO mimetic by binding to EPO receptor, with only limited number of newborns studied [46]. See Table 8.1 for a summary of EPO trials in prematurity. These EPO studies have undergone meta-analyses and Cochrane Systematic Reviews evaluating early or late EPO, or comparing early vs. late EPO [47–50]. Early EPO was defined as beginning therapy at <8 days and late EPO beginning at ≥ 8 days [48–50]. Primary or secondary endpoints to these studies include avoidance of transfusion, number of transfusions, transfusion volume, rise in reticulocytes, or rise in hematocrit. The studies are diverse with respect to degree of stability and birth weight of infants. These studies vary according to timing of initiation, EPO dose, dosing interval, duration of therapy, and route of administration. Initial data supported that EPO might be more efficacious if initiated earlier (before 4 days postnatal age) [36, 51, 52]. However, the Cochrane review did not support this finding [50]. Compared to older individuals, premature infants need a greater per kilogram dosage of subcutaneous (SQ) or intravenous (IV) EPO, secondary to greater plasma clearance, greater distribution volume and shorter mean residence time in premature neonates (see Table 8.1) [53, 54]. Various subcutaneous dosing intervals and continuous intravenous infusion have been examined, with comparable dosing showing no difference in plasma EPO levels, clearance and reticulocyte counts as daily [55]. However, more frequent subcutaneous dosing may be more efficacious [56].
Table 8.1 EPO trials in prematurity
Timing of initiation of therapy: | Day of life (DOL) 2 to DOL 28 (≥ DOL 8) |
rhEPO dose: | 150 to 1,500 U/kg per wk (750 U/kg per wk) |
Dosing interval: | Twice weekly to daily (3 times/wk) |
Duration of therapy: | DOL 10 until 6 weeks, or until hospital D/C (stop before 34 weeks’ gestation) |
Route of administration: | IV bolus, IV continuous drip, SQ |
Nutritional supplementation: | |
Oral iron | 2 mg/kg daily to 36 mg/kg daily (≥4 mg/kg per day optimal, but monitor iron status) |
Parenteral iron | None to 3 mg/kg daily or 20 mg/kg weekly |
Folate | 40 µg daily to 1,000 µg daily |
Vitamin E | 5–25 IU daily (25 IU) |
B12 | None to 21 µg/kg/wk SQ |
Data also support that optimal nutritional supplementation impacts EPO efficacy, Table 8.1. Optimal caloric and protein intake may optimize the effect of EPO [57–59]. Optimal vitamin B12, folate, and iron supplementation may also increase EPO efficacy [60, 61]. In published studies, oral iron supplementation during EPO therapy ranges from 2 mg/kg daily to 36 mg/kg daily; and parenteral iron administration ranges from 1–3 mg/kg daily or 6–20 mg/kg as a weekly injection [36, 52, 55, 62–65]. Optimal nutritional supplementation during EPO therapy remains under investigation.
Meta-analyses from controlled and randomized EPO studies have been performed. Meta-analysis conclusions are limited due to heterogeneity of the studies, including differences in erythrocyte transfusion criteria [47–50]. In Vamvakas and Strauss [47], only 20% met optimal design criteria [12, 36, 66–68]. Table 8.2 summarizes the studies as analyzed by the Cochrane review, early (<8 postnatal days) vs. late (≥8 postnatal days) dosing [48–50]. The meta-analyses of early and late EPO dosing concluded that EPO decreases erythrocyte transfusion number, with the average number of children needed to treat in order to prevent an erythrocyte transfusion being 6–7 [48–50]. Early dosing is not more effective than late and high dosing (>500 IU/kg per week) is not more effective than low (≤500 IU/kg per week) [48–50]. Eliminating early erythrocyte transfusions should be the focus of additional work.
Table 8.2 EPO findings from Cochrane Systematic Reviews
# Infants (EPO/control) | # Studies | Prevent all Tx | Decreased % Tx (% Tx) | Decreased Tx# (#) | Mortality | Morbidity | |
---|---|---|---|---|---|---|---|
Early | 907/843 | 19 | No | Yes (52 vs. 69%) | Yes (2.0 vs. 3.0) | Same (10.0 vs. 9.2%) | None |
Late | 605/537 | 20 | No | Yes (42 vs 60%) | Yes (1.5 vs 2.2) | Same (5.0 vs. 6.3%) | None |
# Infants (early/late) | # Studies | Prevent all Tx | Decreased % Tx (% Tx) | Decreased Tx# (#) | Mortality | Morbidity | |
---|---|---|---|---|---|---|---|
Early/ Late | 131/131 | 2 | No/No | Same (68 vs. 75%) | Yes (2.1 vs. 2.4) | Same (10 vs. 13%) | Early ROP ≥ late |
Notes: Early <8 days of age, Late >8 days of age. All dosages are included.
Transfusion (Tx). Percent of infants transfused (% Tx). Mean number of transfusions/infant (#).
Retinopathy of prematurity (ROP).
Other morbidities examined: IVH, PVL, Sepsis, BPD, NEC, SIDS, hospital stay, neurodevelopment.
Typical side effects of EPO therapy are relatively uncommon in premature neonates. Those seen in adults, including hypertension, thrombosis, bone pain, rash, and seizures are generally not seen. Pure red cell aplasia, which has been reported in adults on chronic therapy, has not been reported in infants [69]. Some studies show decreased absolute neutrophil counts, but no increase in infections [67, 70–72]. Some studies show increased platelet numbers, but no thromboses [71–73]. Some studies cite poor weight gain [69], but this is not a consistent finding. The most concerning potential risk was first seen in retrospective analyses and unique to premature infants, that is, an association between EPO and retinopathy of prematurity (ROP) [74]. Risk factors included >20 cumulative doses of EPO, or later introduction of EPO dosing [74]. A previous Cochrane Systematic Review teased out a greater incidence of ROP in early ESA/EPO group, but a recent update did not find this outcome [48–50]. The physiologic basis for this concern is that EPO serves as a vascular growth factor [75]. However, early ESA/EPO administration occurs during phase I vaso-obliterative stage, characterized by cessation of retinal vascular growth due to hyperoxia, while later EPO dosing coincides with phase II vaso-proliferative stage, which theoretically would be responsive to EPO. Another theoretical EPO complication is iron deficiency, a situation more worrisome in premature infants than other populations due to irreversible learning deficits found in infants with severe iron deficiency [76]. Monitoring iron status is somewhat cumbersome in premature infants and is discussed in more detail later in the chapter.
Potential protective effects of erythropoietic ESA/EPO treatment were decreased incidences of NEC [77] and decreased patent ductus arteriosis [78]. Early erythropoietic EPO dosing may also decrease NEC (number needed to benefit of 33) and IVH (number needed to benefit of 25), [48, 49]. In the Cochrane Systematic Reviews, neurodevelopmental outcome was improved in early ESA/EPO vs. control (number needed to benefit of 13) [48], but was not found in later EPO treatment, although late EPO treatment is due for an update [49]. Exogenous EPO exerts neuroprotective effects in animals. Premature infants given lower, erythropoietic dosages of ESA/EPO were reported to have better neurocognitive outcome and improved as toddlers or preschoolers [79, 80], with improved outcome is potentially related to higher peak EPO levels [79]. One group extended findings to teenage years, finding overall development and IQ scores improved in EPO vs. not, finding that the differences were attributed to those without vs. with intraventricular hemorrhage [81].
EPO for Neuroprotection
The impact of very high dose EPO given immediately after birth for neuroprotection is currently under study in two populations. High dose EPO is under study in term newborns to improve neurocognitive and motor outcomes in those with neonatal encephalopathy [82–84]. High dose neurocognitive prophylaxis using EPO is also under study in premature infants [85–88]. Although optimal dosing and timing of high dose neuroprotective EPO in premature infants still unclear, a recent meta-analysis supported that therapy improved the mental developmental index at 18–24 months, with the number needed to treat of 14 [85]. Long-term outcome assessments from multicenter trials are underway, including the Swiss study [87, 88] and the PENUT study [86]. The PENUT study underway combines high neuroprotective EPO dosing, followed by erythropoietic EPO dosing, a regimen that also may prevent some early erythrocyte transfusions.
Cost
Several studies show that EPO therapy to prevent erythrocyte transfusions in neonates is probably not cost effective [78, 89–91], but many centers dose multiple infants with aliquots from the same drug vial, decreasing cost [92]. In light of worldwide blood shortage and safety issues, cost effectiveness may be less important, but studies in countries with limited resources found that EPO treatment did not decrease erythrocyte transfusions [93, 94].
Summary of rhEpo in Prematurity 2018
In premature infants, advances to improve stability of, minimize phlebotomy, and delaying umbilical cord clamping may decrease the early erythrocyte transfusions in premature infants not normally prevented by erythropoietic dosing of EPO alone. It is also feasible that EPO may be more effective when combined with delayed umbilical cord clamping. EPO is indicated in some premature infants, especially in those born to families of Jehovah’s Witnesses or to parents who object to blood transfusions [47]. EPO at both erythropoietic and high doses may be neuroprotective, making EPO, although moderately effective in prevent erythrocyte transfusions, a better option than multiple transfusions in treating anemia of prematurity.
Iron Status of Premature Infants
Perinatal Iron Acquisition
Iron stores in premature infants increase in proportion to gestational age and birth weight. The healthy term infant’s total body iron content is 75 mg/kg body weight [95, 96]. Normally, fetal iron is accrued at 1.6–2.0 mg/kg daily, even with poor maternal iron status [97, 98]. As much as 80% of body iron is contained in Hb [96]. Thus, premature infants, before their late preterm growth spurt, are born with relatively poor iron status [99, 100]. However, certain groups are endowed with poorer iron at birth, up to 17% of premature infants have biochemical iron deficiency, and thus are at risk for developing iron deficiency anemia [99–104]. These risk factors include cesarean delivery, immediate cord clamping, maternal obesity, maternal diabetes, maternal smoking, being small for gestation, and/or placental insufficiency [99–104]. Although utilized in all body tissues, the greatest demand for iron in the premature infant is erythropoiesis. As neonatal blood volume expands with rapid growth, premature infants produce massive amounts of Hb. For each gram of Hb synthesized, 3.47 mg of elemental iron is required. Erythrocyte expansion needs are more rapid for premature than for term infants, secondary to the relatively faster growth rate. Postnatal iron status for infants (and older individuals) is sensed by iron regulators and controlled through enteral iron transporters. Stable isotope studies show that infants are very efficient at intestinal iron absorption [105–108]. The implications of maintaining adequate iron status during early postnatal life include better neurocognitive outcomes [109].
Measuring Iron Status in Premature Infants
In early postnatal life, interpreting values for the battery of tests used to measure iron status in older individuals is challenging. These tests poorly reflect iron stores in early development, but investigators examining rhEPO in prematurity must try to measure iron status. Although an imprecise index of iron stores in the first months of life [6, 105, 110], plasma ferritin levels are reported in many EPO in prematurity trials [12, 63, 90, 111–115]. Plasma ferritin levels generally fall with increasing postnatal age in all infants, with prematurity no exception [6]. The lower cutoff value for normal plasma ferritin in premature infants is unknown, but is likely much higher than textbook normals of 12 µg/L [96]. In children and adults with EPO-stimulated erythropoiesis, clinicians use cutoff values of plasma ferritin as high as 60–100 µg/L [116, 117]. As with older patients, Maier, et al. recommend <100 µg/L as cutoff [90], while Meyer, et al. recommend <65 µg/L as cutoff to increase oral iron supplementation [115]. In support of these recommendations, an exclusively breastfed cohort of late preterm infants without any supplemental iron, a ferritin value of 70 µg/L at 6 weeks of age predicted developing iron deficiency anemia at 6 months of age [118].
Erythroid maturation and proliferation rely on the transferrin receptor (TfR) pathway for iron delivery [119]. Soluble TfR (sTfR) levels are reported in premature infants receiving rhEPO [64, 120, 121]. In adults, the sTFR is shed from reticulocytes entering the circulation and measures either erythropoiesis or iron status [122, 123]. An early rise in sTfR has been shown to predict erythropoietic response in adults treated with rhEPO [124]. Although sTfR levels have been reported to reflect iron status in newborns [125] and premature infants [120], we found that sTfR levels also correlated to stimulation of erythropoiesis in both term and premature infants [126, 127]. The ferritin index, the sTfR level divided by log plasma ferritin, may overcome this limitation. As in older individuals, the ferritin index could be used during EPO therapy in prematurity [128]. The newer, automated hematology counters report reticulocyte hemoglobin content (CHr), a more sensitive measure of iron-deficient erythropoiesis [117]. Combining the ferritin index with CHr may be of utility [129].
Other studies utilized different markers of iron status during rhEPO treatment in prematurity. Bechensteen, et al. recommend a cutoff value of serum iron <90 µg/100 mL to indicate iron deficiency [57]. In adults receiving rhEPO, hypochromic erythrocytes >6% are abnormal [130], and levels >8% may also be abnormal in neonates, triggering an increase in iron therapy [63, 65, 115, 121].
Zinc protoporphyrin/heme (ZnPP/H) ratios measure incomplete erythrocyte iron incorporation accompanying iron deficiency [131]. ZnPP/H ratios greater than 100 µM/M in rhEPO-treated adults measure incomplete iron incorporation into erythrocytes [132, 133]. ZnPP/H ratio correlates inversely to gestational age, suggesting that rate of erythropoiesis may impact the ratio [134]. However, ZnPP/H ratios also decrease in response to iron therapy or erythrocyte transfusion, supporting the importance of iron supply to the erythrocyte in determining the ratio [135, 136]. As with reticulocyte CHr, examining reticulocyte-enriched ZnPP/H may also be useful for monitoring iron therapy [137].
Postnatal Iron Sources
The anemia of prematurity was traditionally treated with frequent erythrocyte transfusions, but the effects of modern conservative transfusion practices on the long-term iron status of premature infants is poorly studied. Because of the high hematocrit of packed erythrocytes, up to 1 mg of iron is given per mL transfused. This iron “administration” may be clinically significant, as iron stores and plasma ferritin levels rise with erythrocyte transfusions in prematurity [138–140]. EPO treatment does not alter this the association between erythrocyte transfusions and plasma ferritin [8, 21, 59, 66, 67, 73, 111, 112, 115, 128, 141]. As clinical practice increasingly limits number of erythrocyte transfusions given premature infants, the iron allotment from transfusions will decrease. If choosing to treat premature infants with EPO, the potential for tissue iron deficiency is great and requires meticulous monitoring for iron deficiency.
Enteral Iron Supplementation
Data in adults support that iron supplementation is necessary for EPO efficacy. EPO studies in prematurity administered between 2 mg/kg to 6 mg/kg daily oral iron. Several studies found at least one indication for iron deficient erythropoiesis in EPO-treated, oral iron-supplemented infants, compared to oral iron-supplemented controls [55, 63, 64]. High dose oral iron fumarate (18–36 mg/kg daily) is used routinely in Norway and may be associated with less severe anemia of prematurity overall [57, 59].
Parenteral Iron Supplementation
Erythropoietin studies in adults show that parenteral iron increases effectiveness and decreases functional iron deficiency [117]. In prematurity, several neonatal studies examined EPO, combined with parenteral iron [36, 52, 55, 62]. Three studies compared EPO/parenteral with EPO/enteral iron [63–65]. All found similar hematocrit in EPO/parenteral vs. EPO/enteral iron [63–65], but Pollak, et al. found higher reticulocytes and higher plasma ferritin in the EPO/parenteral vs. EPO/enteral iron [65]. Meyer, et al. observed higher hypochromic reticulocytes with EPO/enteral iron [63], while Pollak, et al. found higher hypochromic erythrocytes in both iron groups, compared to controls [65].
Parenteral iron therapy may have toxicity. Deficient antioxidant status of prematurity may contribute to iron-induced free radical diseases of prematurity, such as bronchopulmonary dysplasia, ROP and necrotizing enterocolitis [142–144]. No clinical evidence of iron toxicity was seen in the EPO/parenteral iron studies above, but only one study investigated measures of lipid peroxidation. Immediately after a 2-hour IV infusion of 2 mg iron sucrose, Pollak, et al. found a transient rise in plasma malondialdehyde levels [65]. Because significant hemolysis is observed after erythrocyte transfusion [145], we studied and found elevated plasma malondialdehyde levels in premature infants after erythrocyte transfusion [146]. Caution should be exerted when administering parenteral iron in conjunction with erythrocyte transfusions, at least until further evidence supports that EPO, parenteral iron, and simultaneous erythrocyte transfusions are safe.
Long-Term Iron Status of Premature Infants
Long-term iron status is of major concern in rapidly growing premature infants. Only three studies followed iron status of EPO/enteral iron-treated infants after hospital discharge. Although lower plasma ferritin levels are seen in EPO/enteral iron vs. control infants, it is reassuring that both EPO and control infants have similar plasma ferritin levels at 4–12 months of age [111, 128, 141]. Less reassuring are the extremely low ferritin levels seen (mean levels 21–40 µg/L), with several individual plasma ferritin levels below the 12 µg/L cutoff for iron deficiency in adults [111, 128, 141]. It is possible that avoiding erythrocyte transfusions could endanger the long-term iron stores of rapidly growing premature infants. One recent study determined that human milk is an inadequate iron source for the growing number of late preterm infants, a population unlikely to be transfused and thus to benefit from the iron within erythrocyte transfusions, so must be supplemented early [118].
Conclusion
The anemia of prematurity is multifactorial, but recovers more quickly if blood loss is minimized. EPO is effective, but does not completely eliminate early erythrocyte transfusions in most premature infants. However, delayed cord clamping or enacting restrictive erythrocyte transfusion criteria are also effective as well. Many unanswered, but testable questions include: Which group of premature infants would benefit the most from EPO? How does nutritional supplementation optimize EPO efficacy? Does EPO impair long-term iron status? How are EPO and ROP related? Clinicians should be judicious with its implementation and duration, as it is not yet standard of care. For now, efforts should be focused on measures to prevent early erythrocyte transfusions in premature infants.
References
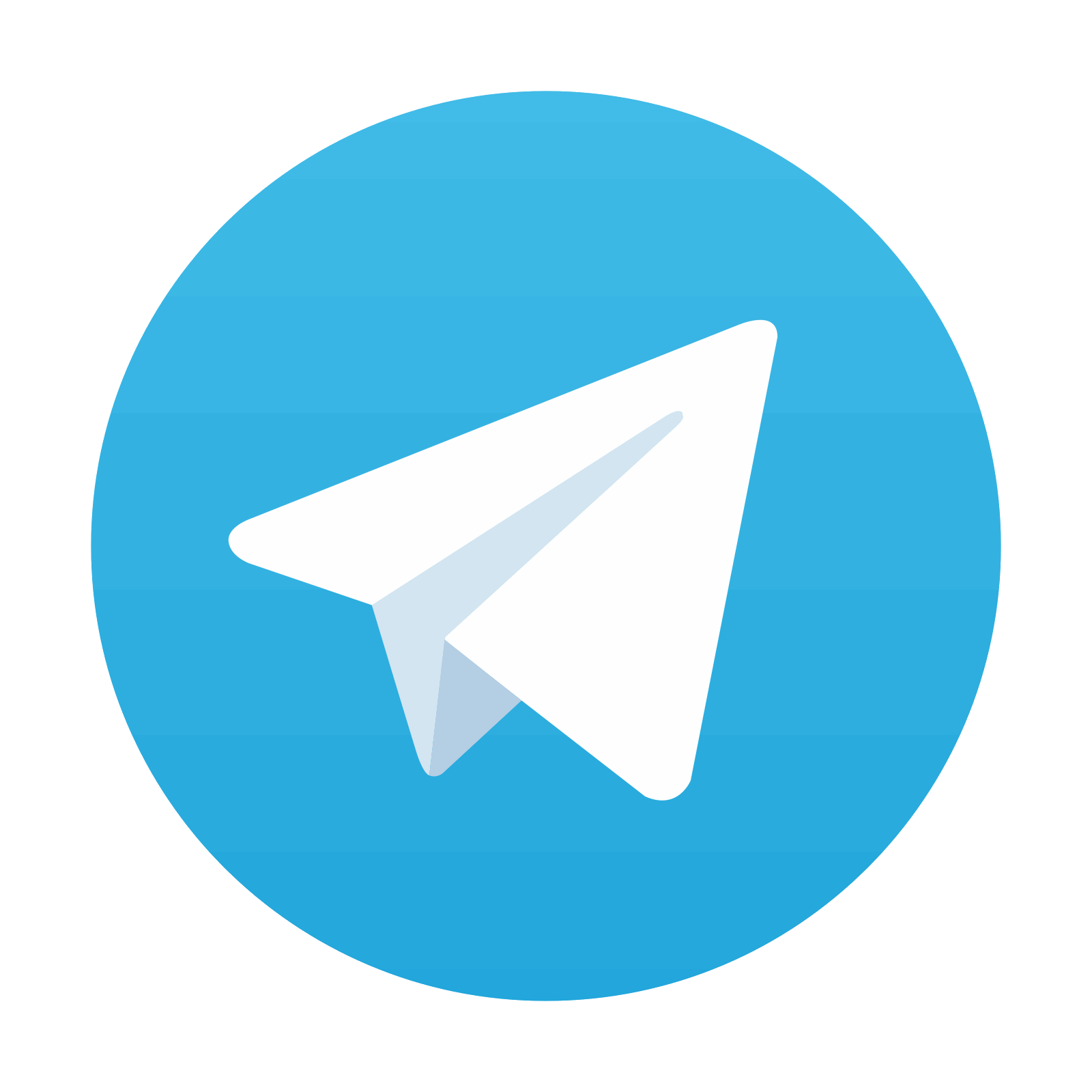
Stay updated, free articles. Join our Telegram channel

Full access? Get Clinical Tree
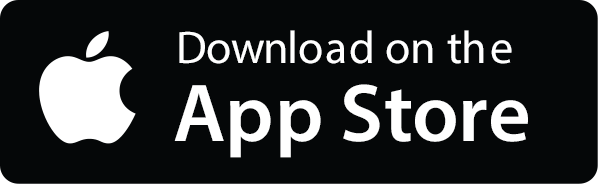
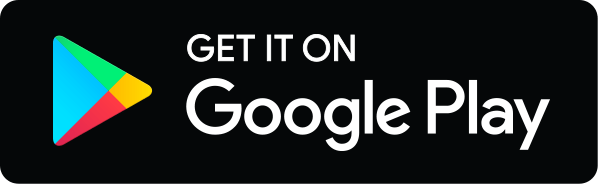
