Abstract
Leukemia in the neonatal period is very rare and can present as early as the day of birth [1, 2]. Acute leukemia arises from clonal changes in hematopoietic precursor cells. In neonatal leukemia, defined as leukemia presenting in the first month after birth, these clonal abnormalities initiate during fetal development [3]. A backtracking molecular study of infants and young children who developed leukemia beyond the neonatal period demonstrated that the same clonal mutations found in the leukemia were also present in neonatal blood spots [4]. Though some epidemiologic studies have suggested that maternal intake of certain foods may contribute, the genetic and environmental risk factors for infant leukemia are not well understood [5–7]. One exception is the observation that an identical twin of an infant with acute lymphoblastic leukemia has a nearly 100% chance of developing the same type of leukemia [8, 9].
Introduction
Leukemia in the neonatal period is very rare and can present as early as the day of birth [1, 2]. Acute leukemia arises from clonal changes in hematopoietic precursor cells. In neonatal leukemia, defined as leukemia presenting in the first month after birth, these clonal abnormalities initiate during fetal development [3]. A backtracking molecular study of infants and young children who developed leukemia beyond the neonatal period demonstrated that the same clonal mutations found in the leukemia were also present in neonatal blood spots [4]. Though some epidemiologic studies have suggested that maternal intake of certain foods may contribute, the genetic and environmental risk factors for infant leukemia are not well understood [5–7]. One exception is the observation that an identical twin of an infant with acute lymphoblastic leukemia has a nearly 100% chance of developing the same type of leukemia [8, 9]. In contrast, the genetic risk factors associated with myeloproliferative neoplasms among neonates are better defined [10]. Neonates with Down syndrome are at risk of transient myeloproliferative disorder (TMD) [11) and neonates with Noonan syndrome or related Ras pathway disorders may present with juvenile myelomonocytic leukemia (JMML) [10]. Both TMD and JMML have the potential to be serious and life-threatening. Recognition of the presenting features of neonatal leukemia is important, as early initiation of therapy may prevent rapid progression of disease.
Acute Lymphoblastic Leukemia
The incidence of acute lymphoblastic leukemia (ALL) in infants under 1 year of age is 2.1 cases per 100,000 age-adjusted US population, or about 100 cases per year in the US [12]. ALL is slightly more common among female infants with a female to male ratio of 1.6:1 [12]. Age less than 3 months at diagnosis, rearrangement of the KMT2A gene (KMT2A-r) on chromosome 11q23, hyperleukocytosis at presentation, and poor response to prednisone therapy are adverse prognostic factors [13–16]. Congenital ALL diagnosed in the first month of life accounts for a minority of cases and is associated with a poor prognosis, with less than 20% overall survival [17].
A hallmark feature of infant ALL is extreme hyperleukocytosis. The white blood cell (WBC) count is typically above 100,000/µL and may be greater than 1,000,000/µL, with lymphoblasts representing the majority of nucleated cells. Alternatively, the leukemia may be of ambiguous lineage, with features of both lymphoblasts and myeloblasts, and therefore is thought to be derived from a very early multipotent hematopoietic precursor cell. Anemia and thrombocytopenia result from poor bone marrow production of other cell lineages. Hepatomegaly and splenomegaly, secondary to infiltration by lymphoblasts, may be associated with liver dysfunction, massive abdominal enlargement, and respiratory insufficiency. Infants with ALL may be lethargic and hemodynamically unstable at presentation, secondary to poor cerebral vascular flow, electrolyte abnormalities, hypoglycemia, or other metabolic derangements. Tumor lysis syndrome, acute renal failure, and disseminated intravascular coagulation (DIC) are oncologic emergencies associated with new onset neonatal ALL. Central nervous system involvement with leukemia, in the form of bulging fontanelle, papilledema, seizures, cranial nerve palsies, or lymphoblasts detected in the cerebrospinal fluid, is common in infants with ALL. Skin infiltration, known as leukemia cutis, is also common and appears as blue to purple, non-tender nodules scattered over the scalp, face, trunk, and extremities. It may be mistaken for excessive bruising and sometimes is the chief complaint that prompts initial evaluation.
The evaluation of a neonate with suspected ALL should include assessment for acute problems requiring immediate intervention (Table 21.1). The diagnosis of ALL is generally apparent by clinical presentation and peripheral blood analysis, but a bone marrow aspirate is helpful to obtain adequate material for cytogenetic studies. If the patient is too unstable to undergo a bone marrow procedure, then peripheral blood may be substituted for all of the necessary diagnostic studies. A lumbar puncture for cerebrospinal fluid testing and administration of intrathecal chemotherapy should be completed prior to the initiation of systemic chemotherapy. Leukemic infiltrates may be seen on a biopsy of a skin nodule, but skin biopsy is not necessary if the diagnosis is established by other means and the rash is typical of leukemia cutis.
Evaluation |
---|
History and physical with particular attention to neurological status, cranial nerve deficits, and leukemia cutis |
Check for dysmorphism suggestive of Down syndrome or Noonan syndrome |
Complete blood count, differential, morphology by peripheral blood smear |
Peripheral blood cytogenetics or FISH for trisomy 21 if Down syndrome suspected |
Basic metabolic profile, serum phosphorus |
Hepatic function panel |
Lactate dehydrogenase |
Uric acid level |
Prothrombin time, partial thromboplastin time, fibrinogen |
Blood group/type and alloantibody screen |
Blood culture if fever or other signs of sepsis |
Bone marrow aspirate for morphologic, flow cytometric, cytogenetic, and molecular testing* |
Cerebrospinal fluid assessment for leukemic blasts** |
Consider CXR, abdominal USS and/or echocardiogram to assess for effusions/ascites |
Genetic testing: GATA1 mutation analysis if TMD suspected; RAS pathway gene mutation analysis if Noonan syndrome, NF1 and/or JMML suspected |
*May substitute peripheral blood if patient is too unstable to undergo a bone marrow procedure
**Instill intrathecal chemotherapy during diagnostic lumbar puncture
Initial stabilization measures may include intravenous hydration, respiratory support with supplemental oxygen or mechanical ventilation, and pressor support. If the neonate is hemodynamically or neurologically unstable and has hyperleukocytosis with WBC count >100,000/µL then corticosteroid therapy should be initiated promptly and a cytoreductive procedure such as manual whole blood exchange or leukapheresis should be considered on a case-by-case basis [18, 19]. The goal of early intervention is to reduce the hyperviscosity associated with hyperleukocytosis and minimize end organ damage. Corticosteroid therapy is the mainstay of anti-leukemia induction therapy. Methylprednisolone or prednisone is given as single agent therapy for up to 7 days prior to cytotoxic chemotherapy [20]. Blood product transfusions should be given to support the hemodynamic status and correct DIC. Caution should be used because cellular blood products may further increase blood viscosity and risk cerebrovascular events. Platelet transfusions may accelerate DIC. Allopurinol should be started to prevent excess uric acid formation. If the uric acid is elevated and/or if the patient has acute renal dysfunction then administration of rasburicase, a recombinant urate-oxidase enzyme, is necessary to prevent irreversible renal damage. Even in the absence of elevated uric acid or serum creatinine, rasburicase should be considered to prevent tumor lysis syndrome with the initiation of corticosteroid therapy. It is important to note that rasburicase is contraindicated in patients with known glucose-6-phosphate dehydrogenase (G6PD) deficiency as it can precipitate severe acute hemolysis. Once the patient is stable, large bore central lines such as pheresis catheters should be removed as soon as possible to avoid catheter-associated thrombosis.
Treatment of neonatal ALL involves corticosteroid therapy and multi-agent cytotoxic chemotherapy. The total duration of therapy is typically 2 years for both boys and girls. Induction therapy lasts for 5 weeks and is a high risk time period for complications, including toxic mortality [21–23]. Invasive infections are particularly problematic among infants undergoing myelosuppressive therapy. Unfortunately, clinical trials have consistently demonstrated that adding more chemotherapy leads to increased toxicities without improving survival outcomes [24, 25]. The majority of infants enter into a temporary remission, followed by early relapse within 1–2 years. Relapsed infant ALL is quite chemoresistant and carries a very poor prognosis [26]. Hematopoietic stem cell transplant (HSCT) can be curative in first remission or following relapse, but is associated with a high mortality rate and many infants still experience relapse post HSCT [27–30].
Rearrangement of KMT2A, formerly known as MLL, is the primary clonal abnormality present in nearly all cases of congenital ALL and is the driver of leukemogenesis [31]. It is associated with lack of CD10 expression on the surface of the lymphoblasts. The KMT2A gene encodes for MLL protein, a histone methyltransferase involved with regulation of gene expression. The most common translocation partner of KMT2A in neonatal ALL is AFF1 (AF4), denoted as t(4;11)(q21;q23), though many other partners of KMT2A have been described [32]. As yet, no clinical drug is available to specifically target KMT2A-r. Overexpression of FLT3, which encodes for a receptor tyrosine kinase, is characteristic of ALL with KMT2A-r and was the target of a novel therapeutic in the Children’s Oncology Group trial, AALL0631 (NCT00557193) [33]. The Children’s Oncology Group trial, AALL15P1 (NCT02828358), is investigating the safety and efficacy of azacitidine, a DNA demethylating agent, in conjunction with chemotherapy for infants with ALL. Immunotherapy approaches are also being trialed and may prove to be of benefit to infants if results show increased cure rates and/or decreased need for chemotherapy.
The rate of event-free survival (EFS) of neonatal ALL with KMT2A-r is less than 20% [17]. Among all infants less than age 1 year with KMT2A-r ALL, the EFS is approximately 40% [20, 34, 35]. In contrast, infant ALL that lacks KMT2A-r is associated with an EFS of >70% [20, 35–37]. As noted previously, relapsed infant ALL is characterized by a very poor second remission rate and the majority of patients succumb to progressive leukemia [38]. Neonates with ALL tend to have lower first remission rates than older infants. Refractory disease, as evidenced by poor response to the first week of prednisone or persistent positive residual disease in the bone marrow during treatment, is indicative of a high risk of leukemia progression [15, 39]. In contrast, neonates who enter into a quick remission and have no detectable residual disease at the end of induction have the best chance of long-term cure.
Neonates with ALL who survive to the age of 3 years without relapse may be presumed to be cured of the ALL. Late relapses are uncommon. Survivors, particularly those treated with cranial radiation therapy and/or HSCT, are at risk of complications of therapy, including growth delay, diminished cognitive ability, cardiomyopathy, secondary acute myeloid leukemia, infertility, and other long-term health problems [40, 41]. Despite these risks, survivors may experience normal growth and development with relatively minimal late effects and normal fertility. As treatments improve with time, more children and adults will survive neonatal ALL and the late effects will become better defined for this cohort.
Acute Myeloid Leukemia
Acute myeloid leukemia (AML) has been reported to be slightly more common than ALL in the infant age range, although it is also extremely rare [42, 43]. The male to female ratio is nearly equal, at 1.1:1 [12]. Excluding AML related to Down syndrome, there are no consistent predisposing genetic or environmental risk factors for neonatal presentation of AML [43]. Similar to ALL in infants, AML in infants frequently involves KMT2A-r as the driving molecular abnormality. The most common translocation in infant AML is t(9;11)(p21;q23) involving the partner gene MLLT3 (AF9) [44]. The prognosis of infant AML with KMT2A-r may be related to the partner gene as well as the breakpoint of KMT2A [45]. Acute megakaryoblastic leukemia with t(1;22)(p13;q13) which leads to fusion of RBM15-MKL1, is also seen with increased frequency in congenital AML and is associated with an intermediate prognosis [46].
Neonates with AML may present with extensive extramedullary involvement [47]. Leukemia cutis is seen in at least 25%–30% of cases of neonatal AML and its appearance is similar to leukemia cutis of neonatal ALL [48]. Hyperleukocytosis, anemia, thrombocytopenia, hepato(spleno)megaly, and central nervous system involvement are also common features between neonatal AML and ALL [1]. In the case of AML, the circulating clonal population consists of aberrant myeloblasts, typically with monoblastic or megakaryoblastic morphologic features [2, 42, 49]. Neonates with AML are at risk of rapid progression to respiratory failure, neurologic deterioration, hemodynamic instability, DIC, and multisystem organ failure. Tumor lysis syndrome is also a risk, but somewhat less prominent in AML than ALL. A rare phenomenon of spontaneous regression of AML has been described in very young infants, particularly those with t(8;16)(p11.2;p13.3), which results in KAT6A–CREBBP fusion [50, 51]. As a result, some clinical trials require infants to be at least 1 month of age or to have evidence of symptomatic or progressive AML for enrolment and initiation of therapy.
The initial workup of a neonate with suspected AML is the same as that of ALL (see Table 21.1). A bone marrow aspirate should be performed unless the baby is too unstable to undergo the procedure. Early interventions include intravenous hydration, cardiopulmonary support, and transfusion of blood products as necessary to promote hemodynamic stability, prevent bleeding and correct DIC. Rapid initiation of broad-spectrum antibiotics should be a priority if fever or other signs of sepsis are present. Leukapheresis should be strongly considered for infants with hyperleukocytosis, defined as WBC count above 100,000/µL, and/or signs of life-threatening organ dysfunction due to involvement of AML [52]. Cerebrovascular events and bleeding complications of DIC are oncologic emergencies associated with hyperleukocytosis in patients with newly diagnosed AML.
Infants with AML are typically treated on the same chemotherapy protocols as older children [53]. The protocols consist of blocks of highly intensive, myelosuppressive chemotherapy. The current treatment in the US is based upon the outcomes of infants treated on the Children’s Oncology Group trial AAML0531 (NCT00372593), and includes five courses of cytarabine-based chemotherapy and gemtuzumab ozogamicin in the first course [54]. The total duration of treatment is approximately 6 months. Among infants in AAML03P1 (NCT00070174) and AAML0531, treatment with gemtuzumab ozogamicin, an anti-CD33 directed antibody-drug conjugate, was safe and associated with favorable disease outcomes [55]. HSCT is incorporated into the treatment of infants and children with AML and certain high-risk features of cytogenetics or response, such as detection of residual disease following the first course of therapy.
The event-free survival of neonates with symptomatic or progressive AML requiring treatment has been reported in the range of 40%–50% [42, 53]. Infants are at high risk of death due to toxicities such as pulmonary or sepsis complications during the induction phases of chemotherapy [23]. Therefore, it is important to keep infants with AML hospitalized until evidence of count recovery following each cycle of chemotherapy. Among survivors of neonatal AML, there is potential for significant late effects of therapy, including secondary malignancies, although the risks are not well defined due to the rarity of neonatal leukemia [40, 53].
Juvenile Myelomonocytic Leukemia
Juvenile myelomonocytic leukemia (JMML) is a rare clonal myeloproliferative disorder, driven by Ras pathway overactivation, with the potential to be rapidly progressive and fatal in young children [56]. When JMML presents in the neonatal period, it is often associated with Noonan syndrome, and is sometimes referred to as JMML-like myeloproliferative disorder of Noonan syndrome (NS/MPD) [10]. The diagnosis of JMML is based on specific clinical and genetic criteria [57, 58].
JMML can be difficult to recognize initially because the clinical findings may overlap with other conditions, such as sepsis [59]. Splenomegaly is a key diagnostic feature. Hepatomegaly, lymphadenopathy, rash, fever, and bleeding may also result from organ infiltration, anemia, and thrombocytopenia. In cases with very early presentation of severe anemia, hydrops may be present. In the peripheral blood, the total absolute monocyte count must be greater than 1000/µL and it may be much higher, and the total WBC count is typically also elevated while the blast percentage is less than 20%. A peripheral blood smear demonstrates increased myeloid precursor cells, including blast cells and abnormal monocytes. A bone marrow aspirate should be obtained, if possible, to rule out acute leukemia. Cytogenetic testing must be performed to show absence of the BCR/ABL rearrangement in the clonal population. Monosomy 7 or other chromosomal abnormalities may be present. In older infants and children, the hemoglobin F percentage may be increased for age but this test may be less informative in neonates with physiologic high hemoglobin F.
With recent advances in genomic understanding of JMML, the diagnostic criteria now incorporate genetic findings. Somatic mutation in PTPN11, KRAS, or NRAS, clinical diagnosis of neurofibromatosis type 1 (NF1) or germ line NF1 mutation, or germ line CBL mutation or loss of heterozygosity of CBL are each diagnostic oncogenetic findings [10]. Individuals with Noonan syndrome and PTPN11, KRAS, or NRAS mutation and patients with CBL mutation harbor a germ line mutation in the Ras pathway [60, 61]. Interestingly, the JMML-like polyclonal myeloproliferation of NS/MPD or of germ line CBL mutation is similar to transient myeloproliferative disorder of Down syndrome, in that it usually resolves spontaneously [62, 63]. In contrast infants and children with NF1-associated JMML always require hematopoietic stem cell transplant (HSCT) and the disease is associated with high relapse rates as well as high disease-related mortality [64]. For this reason, it is critical to determine if a neonate with JMML or a JMML-like picture has a germ line oncogenetic driver mutation. Of note, even some germ line mutations of Noonan syndrome or of CBL may lead to a more rapidly progressive disease in the neonate [65, 66]. As part of the diagnostic workup, molecular testing mutations in the aforementioned Ras pathway genes must be completed on both somatic (peripheral blood or bone marrow) and germ line (e.g., buccal swab) samples. Genetic counseling and molecular testing should be offered for parents and siblings of patients with germ line mutations.
The prognosis for infants and children with JMML is dependent upon age at diagnosis, with older children at higher risk, and cytogenetic factors [64, 67]. The majority of neonates with NS/MPD survive, with spontaneous resolution of the disorder. Among all infants less than 1 year of age with JMML, the rate of disease free survival is approximately 50% and the primary reason for treatment failure is relapse following HSCT [64]. Chemotherapy alone is not curative of JMML but it may be used for control of symptoms related to organ infiltration while awaiting definitive therapy with HSCT [58]. A “watch and wait” strategy is recommended for patients with certain oncogenetic variants [58]. The risk of myeloid malignancies does not appear to be increased for patients with Noonan syndrome who recover from NS/MPD [56, 66].
Down Syndrome Associated Leukemia and Transient Myeloproliferative Disorder
Background
Neonates with Down syndrome (trisomy 21) are especially susceptible to a leukemic disorder variously named transient myeloproliferative disorder (TMD), transient abnormal myelopoiesis (TAM), and transient leukemia of Down syndrome (TL-DS) [68–71]. These terms all refer to the same disorder and for simplicity, the term TMD is used throughout this chapter. TMD is often referred to as a preleukemic condition since retrospective and prospective studies show that the majority of cases spontaneously resolve without chemotherapy and without progressing to full blown leukemia [71, 72]. TMD always has a myeloid phenotype. Indeed, acute lymphoblastic leukemia has never been reported in a child with Down syndrome under the age of 12 months [73]. Importantly, despite the label “transient,” some affected neonates with TMD will develop fulminant, progressive leukemic blast cell infiltration of major organs, which is sometimes fatal. Up to 20% of survivors will ultimately develop a full blown acute myeloid leukemia of Down syndrome (ML-DS) [71, 72, 74]. It is therefore extremely important to be able to recognize TMD in neonates with Down syndrome, to diagnose it accurately and promptly and to monitor it effectively. Fortunately, recent advances in our understanding of the pathogenesis and natural history have allowed the development of consensus guidelines to help achieve these goals [11].
Pathogenesis and Epidemiology
Key to our understanding of TMD has been the discovery of the link to mutations in a single gene (GATA1). We now know that the blood cells in all neonates with TMD carry mutations in the GATA1 gene [69, 71, 75–78]. From a scientific point of view, the association between GATA1 mutations and trisomy 21 provides a unique model in which to study the development of leukemia in children with Down syndrome. From a clinical perspective, the discovery of GATA1 mutations has provided a molecular test to confirm the diagnosis of TMD (see later) and it helps us understand the natural history of TMD and its evolution to ML-DS. The mutations in the GATA1 gene cause the production of a GATA1 protein that is shorter (Gata1s) than the normal GATA1 protein. GATA1s is responsible for TMD although we still do not fully understand the mechanism [69, 75, 77, 79].
Clinical, pathologic and genetic studies show that GATA1 mutations are not inherited; instead they are acquired at some time during fetal life [69, 76]. They can be detected in cord blood, neonatal peripheral blood or on neonatal blood spots [69]. GATA1 mutations only cause TMD in neonates with trisomy 21, i.e., those who have typical features of Down syndrome or in neonates with mosaic Down syndrome [72, 77]. Furthermore, if very sensitive detection methods are used, 25%–30% of all neonates with DS have GATA1 mutations at birth, although only ~1 in 3 with GATA1 mutation (i.e., 10% overall) will have clinical features of TMD [71]. GATA1 mutations are never acquired after birth and they disappear in the first 3–12 months of life except in children with Down syndrome who go on to develop ML-DS [69, 76]. Therefore, GATA1 mutations contribute to the pathogenesis of both TMD and ML-DS. The reason(s) for the high frequency of GATA1 mutations in Down syndrome and how the combination with trisomy 21 causes neonatal TMD and ML-DS is unknown but remains an area of very active research.
Retrospective studies of the prevalence of TMD, carried out before GATA1 mutational analysis was available, are based on a clinical or hematologic diagnosis of TMD. These studies found that TMD affects ~10% of neonates with Down syndrome [68, 70, 72]. The only available prospective study published to date, the Oxford Down Syndrome Cohort Study, included a cohort of babies with Down syndrome who had a blood count, blood smear, and GATA1 mutation analysis in the first week of life whether or not they had any clinical signs of TMD [71]. Taking this unbiased approach, the study found a similar prevalence of TMD based on clinical and hematologic features (9%), but identified a much higher frequency of GATA1 mutations overall (28%). Thus, the majority of neonates with GATA1 mutations did not have clear clinical or hematologic evidence of TMD and were termed “silent TAM (TMD).” The clinical significance of silent TMD is discussed below (see Table 21.2). Even though the GATA1 gene is on the X chromosome, there is no gender difference in the rate or severity of TMD. TMD has been reported in all ethnic groups with no apparent differences between groups [71, 80].
Clinical Features of TMD
The clinical features of transient myeloproliferative disorder are presented in Table 21.2. The classical clinical features of TMD are hepatomegaly, with or without splenomegaly; pleural and/or pericardial effusion and/or ascites; skin rash; and jaundice. In practice, only a minority of neonates will have all of these features. Overall, clinically severe TMD (liver failure/fibrosis, ascites, pleural/pericardial effusion, renal failure and/or coagulopathy) affects 10%–30% of babies with TMD [71, 72, 74, 80]. The skin rash in TMD is typically papular or vesiculopustular, although skin nodules have occasionally been reported [81].
Clinical features of TMD | % of TMD cases in which features seen | Additional comments |
---|---|---|
Jaundice | 70% | Very common, but also seen in around 50% of DS neonates without TMD |
Hepatomegaly | 40% | |
Splenomegaly | 30% | Often due to portal venous obstruction and more commonly in association with hepatomegaly than in isolation. Splenic infiltration rare. |
Pleural and/or pericardial effusion and/or ascites | 25% | |
Skin rash | 11% | Typically, papular and/or vesiculopapular. May be non-specific. Rashes also seen in DS without TMD. |
Bleeding | 10% | Secondary to multiple causes including thrombocytopenia, liver failure, coagulopathy (disseminated intravascular coagulation). |
Hepatic fibrosis +/− failure | ~5% | |
Hydrops fetalis | <5% | Need to exclude TMD but other causes should be considered in a neonate with DS. |
Hematological features of TMD | ||
Leukocytosis | 50% | Common but not diagnostic. Also seen in many DS neonates without TMD and normal in at least a third of TMD cases. |
Circulating blast cells | >20% | Peripheral blood blasts are common in DS neonates both with and without TMD. Importance of GATA1 mutational analysis to confirm diagnosis of TMD, particularly if blast % is <20%. |
Thrombocytopenia | 50% | Common but not diagnostic to TMD. Occurs at a similar frequency in DS neonates without a GATA1 mutation and neonates with TMD may have a normal platelet count or, in some cases, thrombocytosis. |
Neutrophilia | 10%–15% | Neutrophilia is also seen in ~25% of DS neonates without a GATA1 mutation. Increased basophil and myelocyte counts also reported. |
Eosinophilia | 10%–16% | |
Anemia | 5%–10% | Since uncommon as a TMD-related feature, consider other causes of anemia |
It is important not to rely on clinical signs alone to make a diagnosis of TMD. In particular, the presence of jaundice is a very poor diagnostic indicator of TMD because ~50% of neonates with DS but without any evidence of TMD will have jaundice [71]. In addition, neonates with TMD who have a very florid, typical hematologic picture of TMD with high numbers of circulating blasts, may have none of the typical clinical features of the disease. This heterogeneity of clinical presentations means that a blood count and blood smear are essential to make a diagnosis of TMD and also to plan management (see below). Most of the clinical features of TMD are caused by tissue or organ infiltration by leukemic blasts. However, splenomegaly, which is found in 30% of cases, is often due to portal venous obstruction [82]. Splenic infiltration is rarely reported [83, 84].
TMD typically presents at birth or during the first few days after birth. Later presentations are usually because the diagnosis was not considered (and a blood count and blood smear not performed) in the first few days of life rather than because the clinical features appear late. However, in a small proportion of cases signs of liver fibrosis and associated liver failure, including conjugated hyperbilirubinemia and/or coagulopathy, may progress from mild, clinically insignificant features at birth to severe disease 2–3 weeks later. Around 4% of neonates with TMD present with hydrops fetalis, although this is not a specific sign of TMD as there are other causes of hydrops in neonates with Down syndrome, including cardiac disease and infection [83]. TMD may also occasionally present in fetal life when it usually leads to intrauterine or neonatal death [72, 74, 85].
Hematologic Features of TMD
Table 21.2 shows the most common (and the most reliable) hematologic findings in TMD are a raised leukocyte count and the presence of increased blast cells on a peripheral blood smear. Typically, neutrophils, basophils and myelocytes are also increased [71] and a small proportion of neonates with TMD have eosinophilia [86]. There is no difference in platelet count between neonates with and without TMD. This is because trisomy 21 itself causes thrombocytopenia. Indeed, the frequency of thrombocytopenia is ~50% in neonates with Down syndrome whether or not they have TMD. Furthermore, a proportion of neonates with TMD have a raised platelet count (>400 × 109/L). Therefore, it is important to recognize that the absence of thrombocytopenia does not exclude TMD and, equally, that the presence of thrombocytopenia does not identify babies at increased likelihood of having TMD. Giant platelets and megakaryocyte cytoplasmic fragments are commonly seen on the blood smear in neonates with TMD presumably because the acquired GATA1 mutation interferes with normal platelet and megakaryocyte development. The hematocrit and hemoglobin are normal in the majority of neonates with TMD; very few affected neonates are anemic [71, 74].
Blast cells in TMD are extremely pleomorphic. They range from typical myeloblasts with few distinguishing features to characteristic megakaryoblasts with “blebby” cytoplasm to very large cells with a high nuclear to cytoplasmic ratio, which are sometimes binucleate. The percentage of blast cells in TMD is best determined by manual examination of a peripheral smear. In addition, as blast cells often disappear quickly, smears from a sample collected on day 7 of life may look relatively normal. It is therefore important to arrange for blood smear evaluation as soon as possible after birth.
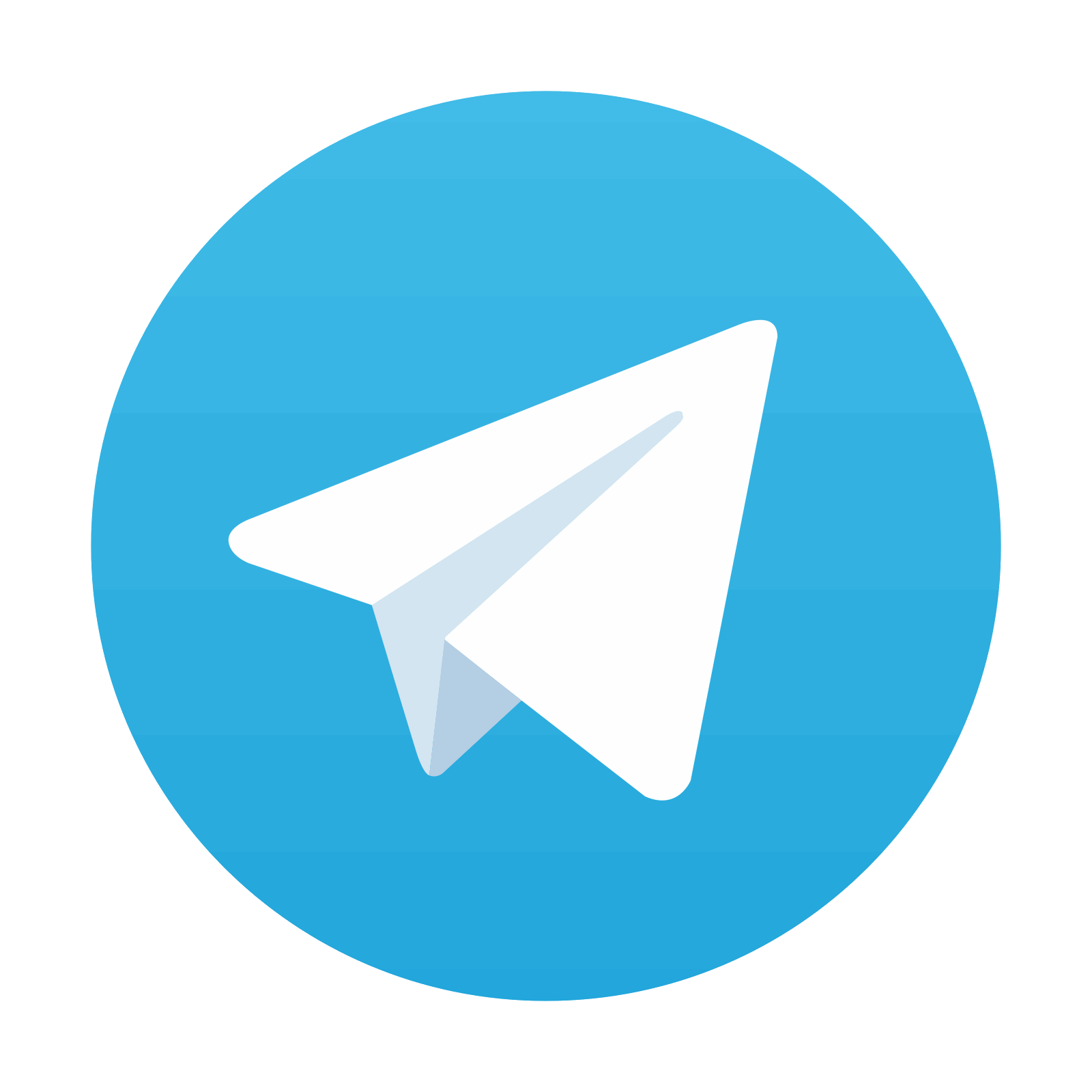
Stay updated, free articles. Join our Telegram channel

Full access? Get Clinical Tree
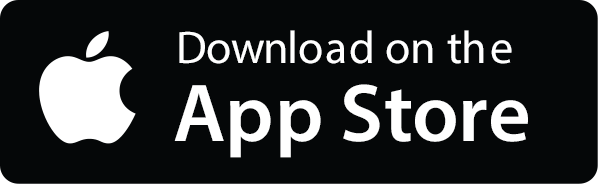
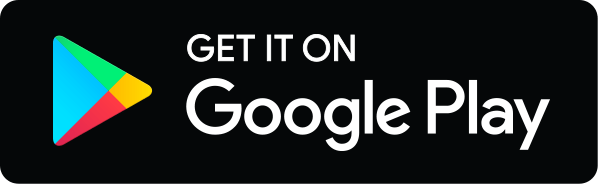
