37329 Cellular Therapy The application of cellular therapies for the treatment of sarcoma is in its infancy, but is rapidly evolving in an era of technological advancement with increasing integration into multidisciplinary oncology care. Given the increasingly prominent role of immunotherapy, clinicians must learn the intricacies of emerging cellular immunotherapies for solid tumors. For solid tumors, progress has been limited, consequent to a unique set of challenges imposed by the tumor microenvironment and the technical challenges of delivering cells into tumors. Sarcomas are certainly challenging with respect to these factors as well as the complexity imposed by extreme biologic heterogeneity across a myriad of subtypes. These caveats result in some tumor types being more amenable to immunotherapeutic approaches than others. This chapter provides an overview of history and development of cellular therapy, which notably originated in transplantation and has experienced early success in treatment of hematopoietic malignancies. The chapter considers challenges currently limiting the field and incorporates more recent and modest attempts at applying cellular therapies toward treating sarcoma. cellular immunotherapy, adoptive cellular transfer (ACT), tumor-infiltrating lymphocytes (TIL), chimeric antigen receptor T cells (CAR-T), engineered T cells, T cell receptor (TCR) T cells, tumor microenvironment (TME), sarcoma cellular immunotherapies, clinicians, sarcoma, solid tumors Cell- and Tissue-Based Therapy, Immunotherapy, Nurse Clinicians, Sarcoma INTRODUCTION Nature often gives us hints to her profoundest secrets.1 —William B. Coley, Memorial-Sloan Kettering surgeon and immunotherapy pioneer Given the increasingly prominent role of immunotherapy, clinicians must learn the intricacies of emerging cellular immunotherapies for solid tumors. For solid tumors, progress has been limited, consequent to a unique set of challenges imposed by the tumor microenvironment and the technical challenges of delivering cells into tumors. Sarcomas are certainly challenging with respect to these factors, in addition to complexity imposed by extreme biologic heterogeneity across a myriad of subtypes. These caveats result in some tumor types being more amenable to immunotherapeutic approaches than others. This chapter begins with an overview of the history and development of cellular therapy, which notably originated in transplantation and has experienced early success in treatment of hematopoietic malignancies. Following introduction of key molecular and immunologic underpinnings, challenges currently limiting the field will be considered, in addition to the unique set of complications and adverse events that are imposed. Throughout the chapter, more recent and modest attempts at applying these therapies toward treating sarcoma will be contextually incorporated. CELLULAR IMMUNOTHERAPY: HISTORICAL BACKGROUND AND PRINCIPLES Historical Background It has been more than a century since William B. Coley boldly repurposed live cultures of Streptococcus pyogenes and Serratia marcescens for the treatment of soft tissue sarcoma (STS) and bone sarcoma patients—a strategy that would later employ heat-killed strains in a process known as “mixed bacterial vaccination.”2 Despite the profound insight gleaned by Coley, only recently has immunotherapy gained broad acceptance as an integral component of multidisciplinary cancer care. Indeed, the term “Coley’s toxins” was far from a term of endearment, a label applied by his many fierce critics based on the treatment’s associated toxicities. Thankfully, with the establishment of several key immunologic principles, the wisdom and rationale of using cellular products to stimulate an immune response is now better understood. While Coley’s approach aimed to stimulate, or otherwise induce an anticancer immune response through vaccination, much of modern-day cell therapy focuses on isolating, expanding, or manipulating immune cell products that have tumor killing capacity. Early efforts centered around isolation of tumor reactive T cells from patients and then reinfusion following stimulation and expansion. Presently, our group and others use genetically engineered cell therapy products to engender tumor antigen recognition through T-cell receptor, antibody, or natural receptor/ligand-based systems. Previously, these approaches were limited in their feasibility and scalability, but now these products are readily manufactured. To date, much of the success with cellular immunotherapy for cancer has been realized with leukemia and lymphoma, commonly referred to as “liquid tumors.”3 Progress with solid tumors, such as STS, has been more difficult, save some notable clinical results in melanoma patients.4 Promising clinical programs are underway for synovial cell sarcoma, but there are no effective immunotherapies for 374the majority STS subtypes.5,6 Success for solid tumors will require a more comprehensive approach to account for immunosuppressive tumor microenvironments and delivery issues.7,8 Clinical Adaptation and Classes of Cellular Immunotherapies Cellular immunotherapy arose from a series of cumulative advances in genetic and molecular engineering, which synergized with parallel advances in cell and tissue culture technique. While working in the field of transplant tolerance in the 1950s, Mitchison made the seminal observation that the cellular arm of the immune system is responsible for tissue rejection.9 When Billingham, Brent, and Medawar transferred cellular material to fetuses in utero, seeking to induce a state of “actively-acquired tolerance,” they performed the first adoptive cellular transfer (ACT) and provided a delivery platform that could be exploited for a panoply of future cellular immunotherapies.10 Other major discoveries that contributed to the modern-day manifestation of cellular therapies included the theory of clonal selection and the technique of hybridoma formation for the generation of monoclonal antibodies.11 The theory of clonal selection solidified the concept that the immune system is composed of distinct functional cell populations and provided irrefutable evidence of the clonality of adaptive immune cells. Meanwhile, monoclonal antibodies provided an armamentarium of specific investigative probes that facilitated the identification of novel molecules and subsequent knowledge of immune signaling pathways. While details of these discoveries are outside the scope of this text, their collective influence undoubtedly shaped the evolution of cellular immunotherapies and the availability of monoclonal antibodies enabled the development of chimeric antigen receptor T cell (CAR-T) products (see later discussion of CAR-T). Lymphokine-Activated Killer Cells In a key technological advance, the Gallo Laboratory of Tumor Cell Biology at the National Cancer Institute (NCI) in Bethesda, Maryland, published reliable methodology for in vitro culture of T lymphocytes isolated from human bone marrow.12 Shortly after the identification, cloning and recombinant expression of interleukin 2 (IL-2), also known as T-cell growth factor, allowed for selection and expansion of tumor-specific lymphocytes for therapeutic purposes.13–15 One of the first applications of ACT against cancer involved the transfer of lymphokine-activated killer (LAK) cells, which are bulk, heterogeneous, nonspecific autologous lymphocytes cultured in recombinant IL-2.16 In a preclinical model of metastatic sarcoma, LAKs were significantly more effective at reducing the number of pulmonary micrometastases than were splenocytes or IL-2 alone.17 A subsequent NCI clinical trial examined the therapeutic response to LAK cells with supplemental IL-2 infusions against a variety of human cancers, including melanoma, renal cell carcinoma, and four sarcoma subtypes—osteosarcoma, neurofibrosarcoma, synovial cell sarcoma, and undifferentiated pleomorphic sarcoma—with objective clinical responses observed in 11 of 25 patients, though none with sarcoma responded. The therapeutic utility of LAK cells was short-lived, however, as a subsequent study in a larger cohort of 181 melanoma and renal cell carcinoma patients compared LAK + IL-2 with IL-2 alone, with no significant benefit seen in patients who received LAK cells.18 Tumor-Infiltrating Lymphocytes Given that LAK therapy involved ACT of nonspecific lymphocytes without defined tumor reactivity, the next iteration of cellular therapies focused on isolation and expansion of tumor-infiltrating lymphocytes (TILs) from surgically resected tumor specimens. Contemporary TIL manufacturing generally involves tumor excision, culturing of tumor fragments supplemented with IL-2, and screening cells for tumor recognition prior to expansion.19 Production of TILs requires a resectable tumor specimen and the presence of infiltrating lymphocytes that can be selected on the basis of their avidity for a tumor-associated antigen (TAA).20 TIL can alternatively be expanded in bulk using anti-CD3/CD28, without the use of TAA, which offers a conceptual advantage over genetically engineered strategies that require a designated TAA. Further, since TILs occur naturally and have matured through the process of thymic selection, there is no concern with off-target tissue damage, which is a concern with other forms of cellular therapy.21 Frequency of TILs does vary by tumor type, as evidenced by Balch et al, who prepared single-cell suspensions from 120 human tumor specimens and quantified the percentage of TILs among tumor cells.22 Renal cell carcinoma had more TILs (65%) than tumor cells (35%), while melanoma had 30% to 35% TILs, and sarcoma had 36% TILs.22 The Rosenberg group from the NCI Surgery Branch were among the first to publish the effectiveness of TIL-based ACT in a mouse model of metastatic sarcoma that uses intravenously injected MCA-105 cells.23 In this model, TILs cleared pulmonary micrometastases and were 50 to 100 times more potent on a cell-by-cell basis as compared to LAK cells, but were unable to eradicate larger, more established 375metastases. To promote in vivo expansion of TILs, preconditioning treatment with cyclophosphamide was incorporated to lymphodeplete the host prior to ACT, which resulted in curing 50% of mice with advanced sarcoma pulmonary metastases with a median survival time (MST) of 84 days, as compared to 0% cure and MST of 21 days among untreated mice. Based on these preclinical findings, an NCI-sponsored clinical trial was similarly designed and conducted to examine therapeutic efficacy of TILs in patients with metastatic melanoma who were preconditioned with cyclophophamide.24 Eleven out of 20 subjects demonstrated an objective response with greater than 50% reduction in tumor burden, and one patient had a complete response (CR), though the durability of response was variable (range 2–13 months). This study also highlighted the difficulty using TILs in the clinical setting, as there were 17 additional enrolled patients who were intended for TIL ACT, but did not receive treatment owing to disease progression during treatment preparation. While the duration of TIL expansion ranged from 24 to 56 days among the 20 patients who received TIL, the inability or delay in culturing TIL for the remaining patients underscores the need for efficient cellular manufacturing processes. This remains an ongoing challenge in the field and has implications for more advanced technologies including autologous CAR-T cell platforms. A shift toward allogeneic, “off the shelf,” products has been advocated by some groups as a possible solution to progression during the production period. In summary, the genesis and early development of TIL ACT was primarily conducted at the NCI over the past three decades. Multiple Phase 1 and 2 trials were conducted to treat metastatic melanoma, in which objective remission rates of 49% to 72%, that is, complete and partial responses, were observed. Two separate publications provide an overview of the TIL experience at the NCI, spanning the periods from 1989 to 1993 and from 2002 to 2007.20,25 Improvements in the ability to culture TIL from tumor specimens are noted, with 60% of tumor specimens yielding TIL in the 1989 to 1993 cohort as compared to 94% from 2002 to 2007. Interestingly, extranodal tumor specimens had greater cytolytic activity in the older data set, while TILs were more easily cultured from lymph node specimens in the recent data set. TILs isolated from metastatic melanoma tumor deposits in gastrointestinal tissue were less likely to yield functional therapeutic TILs, whereas pulmonary and lymph node metastases were generally more successful. These series suggest that greater functional heterogeneity exists among TILs with respect to their tissue of origin and that further methodologic refinements may exist to improve their therapeutic utility. Preconditioning Lymphodepletion Regimens The majority of cell-based therapy protocols involve a preconditioning regimen intended to eliminate suppressive immune cells and create a cytokine milieu that will expand and stimulate adoptively transferred cells. Endogenous T regulatory (Treg) cells were thought to significantly influence local cytokine concentrations in the tumor microenvironment (TME) by acting as “IL-2 sinks” or by inhibiting adoptively transferred TILs through direct cell-cell contact mechanisms. Preclinical models had demonstrated a correlation between the extent of pre-ACT lymphodepletion and clinical response.26 Thus, to improve the objective response rate in humans and to further mitigate the contributions by endogenous Treg cells, investigators incorporated total-body irradiation (TBI) and cytotoxic agents to preconditioning regimens. Compared to a 49% response rate seen with cyclophosphamide and fludarabine preconditioning, addition of TBI, at a dose of 2 or 12 Gy, increased response rates to 52% and 72%, respectively, in metastatic melanoma patients.27 Aggressive preconditioning regimens have been associated with increased serum concentrations of IL-7 and IL-15, which are integral to lymphocyte homeostasis and also promote engraftment. Unfortunately, preconditioning regimens may also increase the likelihood of uncontrolled cell therapy activity, which may lead to severe cytokine release syndrome (CRS) and neurotoxic manifestations, both of which will be addressed below. We have adopted a multidose regional infusion strategy to avoid such complications and provide a more controllable cell therapy clinical treatment platform (NCT01373047, NCT02416466, NCT02850536, NCT03682744).28,29 However, the need for preconditioning will likely vary with the nature of the cell therapy product, disease burden, and clinical indication. Characteristics of Effective TIL Seeking to identify histopathologic correlates of effective TILs, the number of TILs in resected colorectal and ovarian tumors were examined with respect to clinical outcomes, where patients with a higher frequency of T helper type 1 (Th1) TILs demonstrated a survival advantage.30 Th1 cells are activated and differentiated CD4+ T helper cells, which characteristically secrete cytokines, such as interferon-γ (IFN-γ) and IL-2 to promote cell-mediated antitumor responses. Additional phenotypic characteristics of TILs, such as telomere length and expression of the costimulatory molecule, CD28, correlate with 376objective clinical responses and TIL persistence/engraftment following TIL ACT.31 Defining an ideal TIL phenotype for broad application in solid tumors will be challenging, given the vast biologic heterogeneity among patients, and even further among each patient’s varied metastatic tumor deposits. TIL Approaches in Sarcoma The application of TIL ACT for the treatment of sarcoma has been limited, partly because of the difficulty posed by the existence of more than 50 histologic subtypes with a broad range of biologic features.32,33 In one sample of 249 STSs containing at least eight histologic subtypes, only 15% of patients had high-frequency CD8+ TILs.34 Yet, in another study of more common STS subtypes, such as well-differentiated liposarcoma, a substantial number of CD3, CD4, and CD8 lymphocytes were observed.35 To potentially improve lymphocyte frequency within sarcoma specimens prior to TIL production, a Phase 2 trial for patients with advanced/metastatic STS opened in October 2018 and is designed to evaluate the safety and efficacy of an oncolytic peptide injection (LTX-315), which is hypothesized to induce T-cell infiltration prior to tumor resection (NCT03725605). The study is projected to complete enrollment in February 2023. TIL Summary TIL-based ACT has shown objective response rates of approximately 40% in patients with metastatic melanoma, even among those who have failed checkpoint inhibitor therapy.36 However, widespread adoption of this approach has been impeded by the technical difficulties in generating TILs from all patients, the amount of time required to expand TILs, and the toxicities associated with harsh preconditioning regimens. As will be discussed in the next section, T-cell receptor (TCR) or chimeric antigen receptor (CAR) engineered strategies offer alternative cellular therapies for patients whose tumors are not suitable for TIL isolation and production. Furthermore, advanced cell therapy products have shown more promise for sarcoma patients as compared to TIL therapy. Chimeric Antigen Receptor T Cells (CAR-T) Introduction Over the past decade, the introduction of CAR-T has led to meaningful improvements in outcomes for patients, largely for those with hematopoietic malignancies. These “liquid tumors” are readily treated by systemic infusion of CAR-T, but treatment of solid tumors, such as skeletal and soft tissue sarcomas, is hampered by delivery and trafficking challenges inherent to an organized tumor mass. Barriers to delivering CAR-T to solid tumors include aberrant vasculature, migration through dense stroma, and an elevated interstitial fluid pressure (IFP). Furthermore, systemic CAR-T infusions are associated with severe CRS, among other toxicities including on-target/off-tumor effects. Herein, the cellular and molecular principles behind CAR-T immunotherapy, the current limitations of CAR-T treatment for solid tumors, and recent progress in clinical trials using CAR-T to treat sarcoma will be reviewed. Evolution of CAR Therapy Reliable production of highly specific cellular therapeutics has become possible because of advances in genetic engineering of autologous T cells. In 1987, the first immunoglobulin T-cell receptor (IgTCR) fusion proteins were created by Kuwana et al.37 In contrast to TILs, these novel CARs allow production of antigen-specific T cells without isolation from patient tumor samples. Advancement in understanding the TCR signaling apparatus, whereby the TCR CD3 complex was identified as the conduit to T-cell activation, prompted development of the first-generation CAR, where CD3-ζ replaced the α and β TCR chains of the IgTCR (Figure 29.1). Further advances led to the inclusion of costimulatory molecules such as CD28 and 4–1BB in second-generation CAR to enhance activation and persistence in vivo. CAR platforms have the advantage of enabling products to exploit a major histocompatibility complex (MHC)-independent means of antigen-specific activation, thereby enabling its use in any patient with tumors that express the relevant antigen. Other investigators transduced autologous T cells with high-affinity, tumor-specific TCRs, which are MHC-dependent and require HLA haplotype matching between the TCR clone and the patient to avoid the issue of graft–host histocompatibility. One significant advantage of TCR and TIL approaches is that intracellular antigens, presented by MHC, can be targeted. CAR-T, on the other hand, are capable of binding only to tumor antigens expressed on the cell surface. CAR Construct Design and Delivery CAR constructs are composed of an ectodomain, a transmembrane domain, and an endodomain (Figure 29.1). The ectodomain typically consists of an antigen-binding, single chain variable fragment 377(scFv) derived from the light and heavy chains of an immunoglobulin, as well as a peptide linker and a hinge domain. The endodomain contains the CD3-ζ chain to propagate the signal, as well as a costimulatory domain(s). CAR constructs are generally introduced to activated lymphocytes using a lentiviral or retroviral vector, which randomly integrates in the host genome and may cause deleterious effects. Consequently, newer CAR strategies have employed knock-in approaches with gene-editing technologies such as CRISPR-Cas9 to achieve a more controlled, targeted insertion of the CAR transgene.38 FIGURE 29.1 Evolution of chimeric antigen receptors (CARs). (A) Native immunoglobulin structure consisting of 2 heavy (H) chains and 2 light (L) chains, each with variable (VH, VL) and constant (CH, CL) regions. Antigen (Ag) binding site occurs in the variable fragment (Fv) region. Single-chain Fv (scFv) regions are engineered for use in CAR. (B) Native antibody T-cell receptor (TCRs) with associated CD3 subunits propagate intracellular signals through immunoreceptor tyrosine-based activation motifs. (C) First-generation CARs (left) are typically made of scFv and CD3z, joined by a transmembrane linker. Second-generation CARs (middle) are similar to first-generation CARs but have an additional intracellular costimulatory domain (e.g., CD28 or 4–1BB). Third-generation CARs (right) have >1 costimulatory domain. (D) CAR T cells (CAR-T) engage targeted cell surface Ag, resulting in cytokine secretion and tumor cell lysis. Source: Reprinted with permission from Hardaway JC, Prince E, Arepally A, Katz SC. Regional infusion of chimeric antigen receptor T cells to overcome barriers for solid tumor immunotherapy. J Vasc Interv Radiol. 2018;29:1019. doi:10.1016/j.jvir.2018.03.001. Currently, the two FDA-approved CAR-T cell products utilize a second-generation design. More recently, third-generation CAR constructs containing multiple costimulatory domains have emerged, but have not shown clear superiority over first- or second-generation CARs. Fourth-generation CARs that incorporate additional transgenes have also emerged. This class is diverse and covers inducible on/off promoters to regulate CAR expression, suicide genes to eliminate CAR-T in settings of severe immune-mediated toxicities, cytokines, decoy checkpoint receptors, or other immune-modulatory molecules to combat the hostile tumor microenvironment. CAR-T Successes and Challenges Hematopoietic Malignancy The most successful CAR-T therapy to date is CTL019, which targets the B-cell surface antigen CD19. CTL019 was initially approved for treatment of relapsed acute lymphoblastic leukemia (ALL). 378Remarkably, CTL019 achieved complete remission in 90% of patients with refractory ALL, which was sustained in 67% at 6 months.3 Negative effects of CAR-T therapy included CRS, with symptoms ranging from a mild flu-like illness to hypotension, increased vascular permeability, and multiorgan system failure. Neurotoxicity was also seen in the CTL019 trial, occurring mostly as a self-limited encephalopathy but causing seizure activity in some patients. CAR-T Trafficking and Delivery Barriers in Solid Tumors Systemic administration of CAR-T for solid malignancy suffers from an inability to discretely traffic to the tumor bed, which may result in “on target, off tumor” toxicity. This ineffective delivery can result in dose-limiting toxicities, as was the case in Phase 1 trial patients with metastatic colorectal adenocarcinoma, who developed severe colitis upon systemic infusion of CAR-T directed against carcinoembryonic antigen (CEA).39 More devastating consequences occurred in a patient with colorectal metastases to the lung and liver who developed massive CRS after systemic infusion of CAR-T directed against ERBB2 (human epidermal growth factor receptor 2/neu).40 The patient developed respiratory distress, cardiac arrest within 12 hours of infusion, and ultimately died 5 days later. Postmortem analysis revealed low-level ERBB2 expression on lung epithelia, which was thought to have engaged CAR-T and caused massive cytokine release. Two important factors that determine the efficiency of CAR-T delivery into solid tumors include the pressure gradient and distribution of infused cells based on normal blood flow patterns. Pressures within solid tumors can be substantial and can reach up to 130 mmHg, due to disorganized neovasculature with highly permeable capillaries and high levels of extracellular matrix proteins, such as hyaluronan.41,42 As a result, pressures are generally highest at the tumor center with lower levels toward the periphery. Effective intratumoral delivery of any cellular therapy via systemic infusion therefore occurs as a function of the pressure gradient, or the differential between intratumoral and mean arterial pressure. Thus, tumors with elevated pressures impede delivery of CAR-T when standard devices and methods are used. As such, we have combined our regional infusion strategies with high-pressure delivery devices to optimize CAR-T penetration into solid tumors (see “Regional Delivery of CAR-T”).43 From a molecular perspective, many receptor–ligand interactions influence CAR-T navigation into tumor tissue. Our conceptual understanding of T cell homing mechanisms has accumulated from homeostatic and infectious disease studies, whereas manipulation of lymphocyte homing mechanisms is a common immunoevasive strategy employed by tumors.44 More recently, coexpression of the CCR2 chemokine receptor in mesothelin-directed CAR-T was able to increase infiltration by 12.5-fold into mesotheliomas.45 In the sarcoma context, infantile fibrosarcoma (IFS) tumor cells have been shown to express high levels of CXCL10 and CXCL16 chemokines, yet no sarcoma-directed ACT has yet incorporated the use of additional homing mechanisms to increase their efficacy.46 Presumptively, we will see an increasing number of fourth-generation CAR-T that leverage inherent lymphocyte homing machinery to increase therapeutic delivery of CAR-T. Immunosuppressive Tumor Microenvironment Even if CAR-T are adequately delivered to, or effectively trafficked to, a solid tumor, their effect is limited by the tumor microenvironment (TME), which exploits preexisting counterregulatory mechanisms that exist to prevent immune-mediated damage to normal host tissue. The TME is thus immunosuppressed, bathed with inhibitory cytokines secreted by a diverse constituency of myeloid derived suppressor cells (MDSC), Tregs, and tumor-associated macrophages (TAMs). Tregs and MDSCs also express cell surface programmed death ligand-1 (PD-L1) and CD80/86, which directly inhibit CAR-T, TAM, and cytotoxic T lymphocytes (CTLs) through programmed death-1 (PD-1) and cytotoxic T lymphocyte-associated antigen 4 (CTLA-4) receptors. In an osteosarcoma xenograft model using disialoganglioside (GD2)-directed CAR-T, tumor cells were eradicated in vitro, but were ineffective when delivered in vivo due to expansion of murine MDSC.47 However, when mice were treated with all-trans retinoic acid (ATRA), a clinically approved drug that differentiates immature myeloid cells to a nonsuppressive phenotype, GD2-CAR-T regained antitumor effect against osteosarcoma xenografts. Studies from our laboratory have implicated a similar role of hepatic MDSCs as potent mediators of CAR-T suppression in a model of colorectal liver metastasis.7,8 Many studies have identified prognostic correlates of the TME, such as the presence of TILs and improved survival seen among patients with Ewing sarcoma, gastrointestinal stromal tumor (GIST), cutaneous angiosarcoma, and for mixed subtypes, such as leiomyosarcoma, synovial sarcoma, liposarcoma, and undifferentiated pleomorphic sarcoma (UPS).48,49 In another TME analysis of 163 STS specimens—predominantly UPS, synovial sarcoma, fibrosarcoma, and liposarcoma subtypes—PD-L1 379expression and Treg infiltration were associated with tumors that were high grade in patients of advanced age (>65 years old), implicating a potential immunoevasive synergism between PD-L1 expression and Tregs.50 As such, strategies that disrupt PD-L1 and/or deplete Tregs may prove as useful adjuncts in overcoming the immunosuppressive TME in context of cellular therapies. TAMs are also significant contributors to the sarcoma TME, but do not always behave according to classical M1 and M2 subtypes. That is, M1 macrophages are regarded as proinflammatory, secrete both tumor necrosis factor α (TNFα) and IL-12, and directly mediate tumor lysis, whereas M2 macrophages secrete IL-10 and proangiogenic factors to quell inflammation and favor tumor growth. Based on these functional designations, M2-polarized TAMs are characteristically found in the vast majority of human and murine tumors, but not among GISTs, which are M1-polarized at baseline. When mice with spontaneous GIST were treated with imatinib, TAM transitioned to M2, followed by a return to an M1 phenotype among imatinib-resistant tumors. While the unique oncogenes that drive GIST pathophysiology likely influence the behavior of GIST TAM, these observations underscore the likelihood of unique and complex interrelationships between TME constituents and therapeutic interventions.51 As data regarding the sarcoma TME accumulate, it is apparent that the heterogeneous behavior of the sarcoma TME mirrors the diversity of sarcoma subtypes. Regional Delivery of CAR-T Regional infusion of cellular immunotherapies for treatment of hepatic and pancreatic malignancies has been performed using dendritic cells, macrophages, or LAK cells via portal vein injection or hepatic artery infusion (HAI).52 Radiolabeled lymphocytes infused via HAI persist in the liver for up to 120 hours, with only 20% escaping to systemic circulation. Side effects of these therapies are self-limited in nature, with fever and chills most commonly observed. Safety of HAI for delivery of anti-CEA CAR-T (Sorrento/TNK Therapeutics, San Diego, CA) for adenocarcinoma liver metastases was demonstrated in our Phase 1 Hepatic Immunotherapy for Metastases (HITM) trial (NCT01373047), in which the maximum dose was reached without observation of any grade 4 or 5 adverse events.53 Reduced serum tumor marker levels, increased serum interferon-γ, and increased fibrosis/necrosis in posttreatment biopsy specimens were observed. The safety profile of the HITM trial protocol contrasts with the dose-limiting severe colitis that was observed with systemic delivery of anti-CEA CAR-T. We have expanded the HITM method in subsequent Phase 1 trials: HITM-SIR (NCT02416466), which utilized adjunctive yttrium-90 resin microspheres (SIR-TeX Medical, Sydney, Australia) sequentially delivered in delayed fashion after CAR-T and HITM-SURE (NCT02850536), which incorporated the use of a pressure-enabled drug delivery (PEDD) catheter (TriSalus Life Sciences, Westminster, CO) to increase CAR-T delivery.43,54 In the HITM-SURE trial, two of five treated patients demonstrated a complete metabolic response by PET, with one patient remaining alive after 20 months. Recently, we opened a first-in-human, dose-escalation Phase 1 trial with intraperitoneal CAR-T (IPC) infusion for peritoneal metastases or malignant ascites (NCT03682744). As cellular immunotherapies emerge and are refined for the treatment of sarcoma, regional delivery strategies may prove useful to overcome the physical barriers that impede effective delivery. Several abdominal sarcoma subtypes, including GIST and leiomyosarcoma, may metastasize to the liver or recur as disseminated peritoneal sarcomatosis, thereby potentially benefiting from the HITM and IPC methods.55,56 CHALLENGES RELATED TO SARCOMA BIOLOGIC HETEROGENEITY Sarcomas display myriad anatomic, biologic. and patient-related heterogeneity. This diversity demands a complementary armamentarium. Thus, a diverse array of cellular targets will likely need to be elucidated, with additional therapeutic considerations made for their respective TMEs, anatomic restraints, and age-associated influences. Current data sets have been curated from similarly heterogeneous populations or specimens and are inadequate toward these goals. For example, a study seeking to examine factors relevant to local recurrence of sarcoma among a population of patients largely composed of leiomyosarcoma has failed to acknowledge the biologic tendency of leiomyosarcoma to recur distantly.57 Investigators who are developing autologous cellular therapies for pediatric sarcomas are working with a functionally different leukocyte donor population than would be expected from a geriatric donor, based on observations that geriatric CAR-T are functionally impaired with respect to proliferation, signaling activation, and perforin/granzyme B mediated cytotoxicity.58 Combining the intricacies and complexities of cellular immunotherapy with the multifaceted heterogeneity of sarcoma, the corresponding investigative approaches must be cogently designed to account for unique biologic features of specific sarcoma subtypes. 380Target Antigens in Sarcoma Before delving into relevant trials in which engineered TCR and CAR-T were used to treat sarcomas, a discussion of suitable target antigens is warranted. The family of cancer testis antigens (CTAs) have garnered attention as target antigens for sarcoma, as well as other malignancies. Expression of CTA is restricted to germline tissues, placental trophoblasts, and a variety of malignancies.59 Further, germline cells do not express MHC molecules, which renders them immunologically privileged and thus “invisible” to TCR-based approaches, which rely on MHC recognition. Thus, any cellular therapy directed against these CTAs would preferentially attack cancerous cells. Expression of several HLA*02.01 restricted CTA family members NY-ESO-1, LAGE-1, PRAME, MAGE-A3, MAGE-A4, MAGE-A9, and SSX-2 were reported across multiple sarcoma subtypes.60 Though there were fewer than 10 tumor specimens examined for each subtype, characteristic expression patterns were observed for synovial cell sarcoma, liposarcoma, and leiomyosarcoma, as seen in Table 29.1. The majority of synovial cell sarcomas express NY-ESO-1, particularly the monophasic histologic type; however they typically do not express MAGE-A1 or CT7.61 Myxoid and nonmyxoid liposarcoma were found to be positive for LAGE in >70% and 60% of specimens tested, respectively.62 Expression of MAGE-A3 was seen in 66% of uterine leiomyosarcomas, but only in 14% nonuterine leiomyosarcomas.63 Lastly, treatment with 5-aza-2-deoxycitabine (5-Aza-dC) was shown to induce expression of NY-ESO-1, LAGE-1s, and PRAME among chondrosarcoma cell lines and tumors that previously had no or low-level expression of these CTAs.64 GD2 was shown to be highly expressed (>60% of tumor cells) among 83% of pediatric osteosarcomas and 13% of pediatric rhabdomyosarcomas, with 20% of pediatric Ewing sarcomas having low-level expression.47 Additional osteosarcoma targets include ERBB2 and IL-11 receptor α (IL-11Rα), both of which are expressed on the cell membrane and are suitable for CAR-T therapies.65,66 CAR-T and TCR Trials for Sarcoma Clinical trials of cellular therapy for sarcoma patients have been challenging and have evolved over time. Table 29.2 provides an overview of completed and ongoing sarcoma clinical trials. Owing to the general difficulty in generating TILs from nonmelanoma tumor specimens, early clinical trials using cellular immunotherapy for sarcoma utilized autologous T cells isolated from peripheral blood that were engineered to express TCRs against NY-ESO-1, because NY-ESO-1 is expressed in >80% of synovial cell sarcomas. Robbins et al. from the Surgery Branch of the NCI were the first to report a series of six patients with metastatic synovial cell sarcoma, previously treated with high-dose IL-2, who received a single dose of anti-NY-ESO-1 TCR T cells and supplemental IL-2 infusion after a preconditioning regimen of cyclophosphamide and fludarabine.6 Partial responses were observed in four of six patients, which lasted up to 18 months in one patient who received two infusions of TCR-transduced T cells, whereas one of the patients with progressive disease had only received one-third of the dose that others in the cohort received.6 A follow-up report from the same group 3 years later included an additional 11 patients, with six partial responses (maximum duration 47+ months) and one complete response that had persisted beyond 20 months.5 Cumulatively, 11 of 18 patients (61%) developed an objective response, and there was one treatment-related death during the course of the trial, which occurred as a consequence of neutropenic septic shock 3 days after ACT. The number of T cells specifically reactive to NY-ESO-1 peptide-pulsed target cells correlated with response to therapy, while the overall persistence of adoptively transferred T cells did not. An additional trial is currently underway to more broadly examine NY-ESO-1 TCR-based approaches for skeletal and STS (NCT03462316). Other CTAs, such as MAGE-A3, MAGE-A4, and MAGE-A10 are being targeted with TCR-engineered T cells for multiple sarcoma subtypes (NCT03132922, NCT03132922, NCT02989064, NCT03139370, NCT02111850, NCT02153905).67
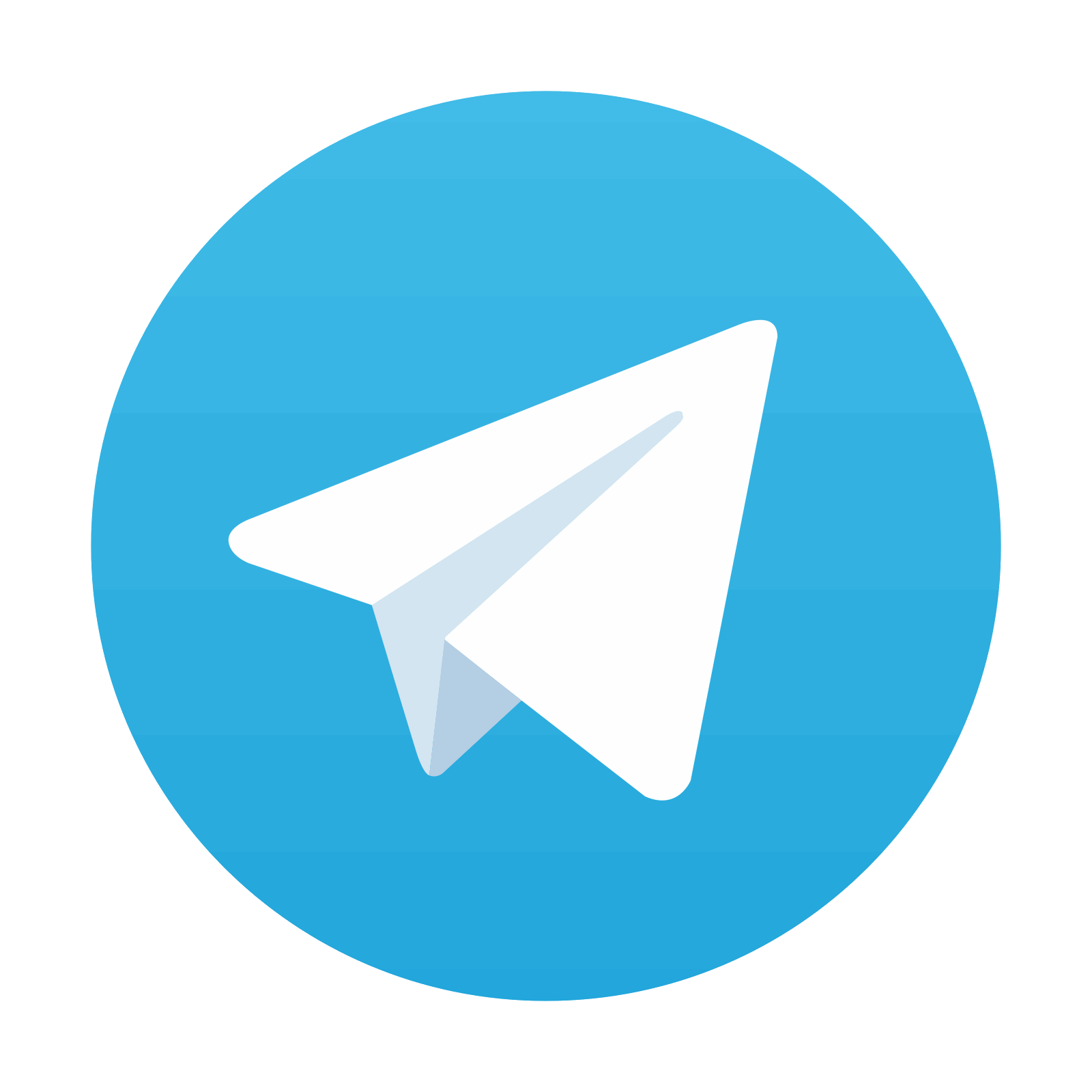
Stay updated, free articles. Join our Telegram channel

Full access? Get Clinical Tree
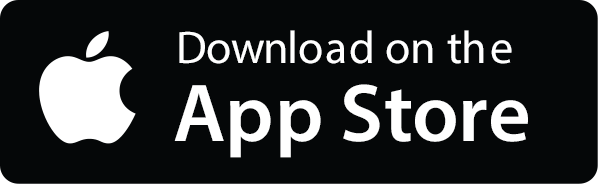
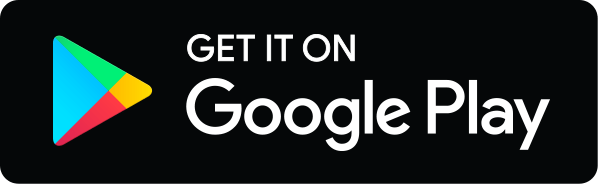