Cell-based cancer immunotherapy
Krina K. Patel, MD Judy S. Moyes, MA (Cantab), MB, BChir, FRCPC, FRCPCH
Laurence J. Cooper, MD, PhD
Overview
For decades, scientists have applied cell-based immunotherapies for treatment of cancers. The successful administration of polyclonal populations of donor-derived or autologous T cells is based in part on defining the precise cellular effectors and identifying tumor-associated antigens (TAAs) that can be targeted to safely achieve an antitumor effect without engendering on-target, but off-tissue toxicity, such as manifested by graft-versus-host-disease (GvHD). The adoptive transfer of T cells can reconstitute or enhance resident immunity, which is in contrast to the apparent limitations associated with vaccine-based strategies in recipients whose endogenous immune system may fail to respond to immunogen. Other cytolytic circulating lymphocyte populations can be harnessed for infusion. These include allogeneic NK cells that may mediate an antitumor effect without a priori defining the TAA and perhaps without the risk of GvHD. In some cases, a TAA can be identified, for example, the B-lineage TAA CD19, but host immune tolerance prevents the emergence of an effective immune response. Genetic engineering has been combined with T-cell therapy to generate antigen-specific cells ex vivo, through the enforced expression of chimeric antigen receptors (CARs). The adoptive transfer of CD19-specific CAR+ T cells has shown promise in initial clinical trials for successful disease control in patients with B-cell malignancies resistant to conventional therapeutic modalities. Here, we describe the current status of cell-based immunotherapy for cancer.
Introduction
Allogeneic hematopoietic stem-cell transplantation (HSCT) can be considered as the first effective form of cellular-based therapy for hematologic malignancies. Successful outcome for patients undergoing allogeneic-HSCT is directly linked to the emergence of a graft-versus-tumor (GvT)-effect mediated by donor-derived immune cells. The relevant cellular immune components of the allograft include T cells, natural killer (NK) cells, and B cells that are recognized as potential effectors of the GvT-effect. The observation that engrafted T cells can eliminate residual tumor cells led to the use of donor leukocyte infusions (DLI) to treat a subset of relapsed leukemias, which provided direct evidence for a GvT reaction.1 While cytotoxic T cells are the primary mediators of the GvT effect, unfortunately, they also are closely associated with development of graft-versus-host-disease (GvHD)2 as major and minor histocompatability antigens can be shared between malignant and normal cells. In practical terms, DLI and allogeneic HSCT are limited by GvHD offsetting the benefits of the GvT effect.
For several decades, scientists have applied cell-based immunotherapy for cancer. The successful administration of polyclonal populations of donor-derived or autologous T cells is based in part on defining the precise cellular effectors and identifying tumor-associated antigens (TAAs) that can be targeted to safely achieve an antitumor effect without engendering on-target, but off-tissue toxicity, such as manifested by GvHD. The adoptive transfer of T cells can enhance resident immunity, which is in contrast to the limitations associated with vaccine-based strategies in patients whose endogenous immune system may fail to respond to an immunogen.3, 4 Other lymphocyte populations can be harnessed for infusion. These include allogeneic NK cells that may mediate an anti-tumor effect without a priori defining the TAA and without the risk of GvHD.5–7 In some cases, a TAA can be identified, for example, CD19, but tolerance prevents the emergence of an effective immune response. Genetic engineering has been combined with T-cell therapy to generate antigen-specific cells ex vivo, through the enforced expression of chimeric antigen receptors (CARs). Indeed, the adoptive transfer of CD19-specific CAR+ T cells has shown promise in initial clinical trials for successful disease control in patients with B-cell malignancies resistant to conventional therapeutic modalities, as summarized in Table 1. In this chapter, we will describe the current status of cell-based immunotherapy for cancer.
Table 1 Selected CD19 CAR T-cell trials
Type of CAR | Costimulatory domain | Cancer type | Outcomes | Total number of patients | Toxicities | References |
Lentivirus | 4-1BB | ALL | 27 CR | 30 | Severe CRS | Maude et al.8 |
Gammaretrovirus | CD28 | ALL, NHL | 14 CR | 19 | Severe CRS | Lee et al.9 |
Gammaretrovirus | CD28 | ALL | 14 CR | 16 | Severe CRS | Davila et al.10 |
Gammaretrovirus | CD28 | DLBCL, indolent lymphoma, CLL | 8 CR, 4 PR, 1 SD, and 2 were not evaluable | 15 | Neurologic, delirium | Kochenderfer et al.11 |
Lentivirus | 4-1BB | CLL | 3 CR, 5 PR, and 6 no response | 14 | Fever | Porter et al.12 |
ALL, acute lymphoblastic leukemia; NHL, non-Hodgkin lymphoma; DLBCL, diffuse large B-cell lymphoma; CLL, chronic lymphocytic leukemia; CR, complete remission; PR, partial response; SD, stable disease; CRS, cytokine-release syndrome.
Generation of cellular therapy products
T cells
The immune system of the patient with cancer can be manipulated through the administration of lymphocytes as well as immunomodulatory approaches aimed at enhancing the endogenous T cells’ adaptive ability to kill tumor cells. Both of these approaches have led to antitumor effects. Clinical trials infusing antibodies to break checkpoint blockade against CTLA4 and the PD-1/PDL-1 axis lead to activation of an endogenous antitumor immune response and have reported significant long-lasting responses.13–15 Analysis of biopsy samples reveals that by blocking the PD-1/PDL-1 axis, the antitumor effect is correlated with the baseline density and location of T cells in metastatic melanomas.16, 17 For some tumors, there may not be sufficient quality or quantity of infiltrating T cells in the tumor microenvironment. These tumor types may be targeted by an exogenous source of ex vivo expanded effector cells. Thus, adoptive cellular therapy (ACT) has emerged as a highly effective modality capable of eliciting sustained clinical responses for some hematologic malignancies as well as solid tumors as described in Table 2.
Table 2 Selected cellular therapy trials for solid tumors
Cancer type | Type of ACT | Target antigen | Outcomes | Total number of patients | Toxicities | References |
Melanoma | TCR | MART-1; gp-100 | 8 PR; 1 CR | 36 | Skin, eyes, and ear | Johnson et al.18 and Morgan et al.19 |
Melanoma, synovial sarcoma, and esophageal cancer | TCR | MAGE-A3 | 1 CR, 4 PR, 4 no response, and 2 deaths | 9 | Recognized MAGE-A12 expressed in the brain and led to neurotoxicity: 3 patients with mental status changes, 2 of whom went into comas and subsequently died | Morgan et al.20 |
Melanoma, high-risk multiple myeloma | TCR | MAGE-A3 | Both patients developed fever, progressive hypoxia, and hypotension and expired 4–5 days after infusion | 2 | Crossreacted with Titin protein on cardiac myocytes which led to heart failure and death | Linette et al.21 |
Synovial sarcoma; melanoma | TCR | NY-ESO-1 | 2 CR; 7 PR | 17 | None | Robbins et al.22 |
Renal cell carcinoma | 1st gen CAR | CAIX | No objective responses | 11 | Hepatotoxicity | Lamers et al.23, 24 |
Colorectal cancer | TCR | CEA | 1 PR | — | Colitis | Ma et al.25 |
Colorectal cancer | 3rd gen CAR | ErbB2 | 1 death | 1 | Fatal lung toxicity and organ failure from recognition of normal lung ErbB2 expression | Morgan et al.26 |
Ovarian cancer | 1st gen CAR | αFR | No objective responses | 14 | IL-2 related | Kershaw et al.27 |
Neuroblastoma | 1st gen CAR | GD2 | 3 CR | 19 | Pain | Pule et al.28 and Louis et al.29 |
In general terms, five forms of T cells have been manipulated for human application. These are (1) lymphokine-activated killer (LAK) cells; (2) cytokine-induced killer (CIK) cells; (3) tumor-infiltrating lymphocyte (TIL) using lymphocytes expanded from a tumor biopsy; (4) antigen-specific T cells based on identification and/or selection of endogenous and typically circulating T cells; and (5) genetically engineered T cells that express a TAA-specific T-cell receptor (TCR) or CAR.
Infusion of LAK cells, produced from peripheral blood after culturing with high doses of interleukin-2, was one of the first applications of ACT.30 Unfortunately, high dose IL-2 led to terminal differentiation and inability to recycle effector function. Furthermore, the positive responses seen in early clinical trials with LAK cells could have been the result of the IL-2 coinfusion.31 However, the clinical data associated with LAK cells led to the development of TIL, which have antitumor activity, especially for patients with melanoma. CIK cells are a heterogeneous subset of ex vivo expanded T-lymphocytes that present a mixed T-NK phenotype and are endowed with major histocompatibility complex (MHC)-unrestricted antitumor activity32; antitumor cytotoxic activity is represented by surface markers for both T cells (CD3+TCR α+β+) and natural killer T (NKT) cells (CD3+CD56+).33 T cells that express a TCR composed of αβ heterodimers are the most prevalent T-cell population in peripheral blood; thus, they have been the most widely studied for ACT. Both effector (CD8+) and helper (CD4+) T cells have been evaluated for ACT as bulk populations, antigen-specific clones, or subsequent to genetic modification to express TAA-specific receptors.
Tumor infiltrating lymphocytes (TILs)
Animal studies demonstrated that TIL were significantly more effective than LAK cells in eliminating tumors,34 prompting clinical trials infusing in vitro expanded TIL for patients with metastatic melanoma.35, 36 These trials were begun at the NIH and are now being undertaken worldwide.37–39 These clinical data reveal that subsets of the inoculum recognize TAAs that can be identified as mutated epitopes based on whole-exon sequencing of tumor samples.40, 41
Rosenberg and colleagues at the Surgery Branch of the National Cancer Institute (NCI) pioneered and standardized methods for TIL expansion for ACT.42 First, during the pre-expansion phase, surgically excised tumor specimens were digested and cultured in single-cell suspensions with media containing IL-2, examined for growth and reactivity, and then selected for further expansion and characterization. Early studies showed promising results35 with remarkable objective response rates of about 50% in metastatic melanoma. Inopportunely, only a subset of patients who were initially enrolled in most of these trials actually underwent infusion of TIL.43 The lack of an available tumor for surgical harvest, difficulty to isolate and grow TIL from harvested tumor, or inability to show robust, specific effector function of isolated TIL were all reasons that have confined this treatment strategy to academic centers skilled in the generation of this type of cellular product. Toxicities such as hypotension, arrhythmias, and vascular leak syndrome were attributed to the high doses of recombinant IL-2, which was coinfused to improve TIL persistence. The judicious selection of recipients for TIL therapy and management of patients receiving high-dose IL-2 has improved outcomes in subsequent trials. Specifically, those patients who achieved complete remissions (approximately 1/5 of all patients treated) remained disease-free 3 years or greater after treatment.44 Furthermore, it appears that IL-2 at high doses may not be needed, which will likely broaden the application of this therapy.45, 46
In efforts to improve the outcome of TIL therapy, a lymphodepletion regimen was added before TIL transfer.47–49 Lymphodepletion is thought to enhance the persistence of T lymphocytes by providing a pro-proliferative environment through elimination of cytokine sinks (which leads to increased availability of prosurvival cytokines, e.g., IL-7 and IL-15), myeloid-derived suppressor cells and T regulatory cells.50 The addition of lymphodepletion led to the engraftment of TIL and improved the clinical responses. As a consequence though, the loss of a normal immune defense system led to increased rates of opportunistic infections.
The degree of lymphodepletion appears to impact the antitumor response. Non-myeloablative lymphodepletion regimens with cyclophosphamide and fludarabine achieved response rates reaching 51%.51, 52 When total body irradiation (TBI) was included at doses that required rescue of hematopoiesis with autologous hematopoietic cell transplantation, the overall response rate increased to 70% with complete remission (CR) of 40%; however, the increased intensity of the conditioning regimen was associated with more serious adverse toxicities including febrile neutropenia, infections, and thrombotic microangiopathy.44, 48
A limitation of using TIL has been patient attrition owing to disease progression or overall clinical decline while waiting for TIL to meet release criteria for administration. Thus, strategies are being developed to decrease the time in culture from TIL collection to infusion.53 In a recent trial, shortening of the pre-expansion phase allowed TIL to be produced within 28 days which led to a 74% enrollment. These “younger TIL” may also have improved therapeutic benefit as they contain T cells that are not terminally differentiated and avoid replication senescence.54 Approaches using activation and propagation cells (AaPC) as irradiated feeders to numerically expand TILs are also emerging.55 These feeder cells may thus help broaden the number of patients who can benefit from TILs and enable tumor-specific T cells to be generated without the need to harvest tumor.
As knowledge about immunotherapy grows rapidly, the number of current clinical trials employing TIL therapy also increases. These include comparing lymphodepleting regimens (NCT01807182; Fred Hutchinson Cancer Center), combination immunotherapy with CTLA-4 (NCT01701674; Moffitt Cancer Center) or PD-1/PDL-1 axis blockade or vaccines (NCT00338377; MD Anderson Cancer Center), and evaluating TIL efficacy in tumor types other than melanoma (NCT01174121; National Cancer Institute).
Peripheral blood T cells
TAA-specific T cells can be collected from peripheral blood using MHC multimers containing peptide epitopes. These are complexed to a fluorescent reagent to stain circulating T cells. The desired population of autologous effector cells can then be sorted, activated, and expanded in vitro and infused into the patient to induce tumor regression and long-term immunoprotection.45, 56 The prevalence of TAA-specific effector T cells in peripheral blood can be rare (on the order of 0.2%); however, some studies in melanoma have reported T cells specific for melanocyte antigens such as gp100, tyrosinase, and MART-1 at frequencies reaching 2%.57 This in vitro study also suggested that even though systemic TAA-specific T-cell responses can develop de novo in cancer patients, antigen-specific unresponsiveness may explain why such cells are unable to control tumor growth. Therefore, activation of the TCR in the presence of costimulatory molecules, followed by cytokine-induced expansion, is needed to enrich the TAA-specific effector T cells.58 TCR engagement is mediated by the peptide–MHC complex that presents the antigen to the T cell.
The very low frequency of T cells recognizing commonly expressed antigens limits the applicability of this approach. To overcome this instability, Pollack et al.59 tested the strategy combining IL-21 modulation during in vitro stimulation with tetramer-guided cell sorting to generate TAA specific cytotoxic T lymphocytes. T cells stained with the TAA NY-ESO-1 tetramer were enriched from frequencies as low as 0.4% to >90% after single pass through a clinical grade sorter. NY-ESO-1-specific T cells were generated from all patients included in the study and the final products expanded on average 1200-fold were oligoclonal and contained 67–97% CD8+, tetramer+ T cells with a memory phenotype that recognized endogenous NY-ESO-1.
Peripheral blood dendritic cells
Autologous dendritic cells (DCs), a class of professional antigen-presenting cells, are used to selectively propagate autologous T cells by coculture in vitro. To obtain DCs, monocytes from peripheral blood are cultured in IL-4 and granulocyte-macrophage colony-stimulating factor (GM-CSF) to dedifferentiate into immature DCs. These immature DCs can then be loaded with tumor antigen or lysate and cultured in cytokines to mediate maturation and upregulation of costimulatory ligands such as CD80 and CD86. Alternatively, the use of peptide to pulse mature DCs generates high-affinity CD8+ and CD4+ T cells recognizing only a single antigenic epitope. Artificial antigen-presenting cells (aAPCs) have also been developed as an alternative to DCs, derived from the human erythroleukemia cell line, K562. These cells express HLA (human leukocyte antigen) C alleles and no other classical HLA class I or II molecules and can be genetically modified to express TAA, HLA, and costimulatory molecules to optimize generation of the antigen-specific T-cells.55, 60
Both TIL and antigen-specific T-cell therapy provide complementary strategies for the treatment of patients with solid tumor malignancies and represent promising prospects in the development of ACT as a treatment modality. They not only provide effective treatment for patients but also supply a rich source of data for immunobiological discovery.
Table 3 Methods typically used to eliminate mispairing between introduced and endogenous alpha/beta TCR
Various methods to facilitate introduced αβ TCR pairing | References |
Use of full or partial mouse TCR α and β | Cohen et al.,74 Sommermeyer and Uckert,75 and Cohen et al.76 |
Addition of another disulfide bond within constant region | Kuball et al.77 and Cohen et al.78 |
Use of Knob-into-hole configuration | Voss et al.79 |
Application of short hairpin RNAs (shRNAs) against TCRαβ constant regions to suppress endogenous TCRαβ expression | Okamoto et al.80, 81 |
Introduction of zinc finger nucleases (ZFNs) or other artificial nucleases to completely disrupt endogenous TCRαβ expression at genomic level | Provasi et al.,82 Poirot, et al.,83 Torikai et al.84 |
Genetically engineered T cells
With advancements in immunology and gene transfer, T-cell function has been enhanced by altering receptor specificity and signaling functions that control ability to recycle antigen-dependent effector functions.61 A justification for genetic retargeting of T cells is that the endogenous TAA-specific T-cell repertoire undergoes immune-mediated tolerance rendering these cells unable to either recognize or effectively respond to malignant cells. Two types of genetically modified T cells are in current clinical trials for oncology based on redirecting specificity through enforced expression of defined TCRs or CARs.
Efficient gene transfer into T cells is necessary to generate both TCR and CAR cellular products. γ-Retroviral and lentiviral transduction techniques rely on the propensity of these viruses to integrate permanently into the host genome. GMP (good manufacturing practice)-grade viral vector production is a costly, time-consuming, and specialized technique. Despite early concerns about insertional mutagenesis, decades of experience with transduction of primary T cells have failed to show any examples of this theoretical complication.62 Nonviral gene transfer is an alternative and relatively cheaper approach. The most commonly used approaches are transposon/transposase systems such as Sleeping Beauty. This system results in sustained expression of CAR on T cells, and anti-CD19 transposon CAR T cells are in early-phase clinical trials.63 Another novel approach is the electroporation of T lymphocytes with in vitro transcribed RNA.64 This leads to high, but transient, expression of the CAR. With the exception of the mRNA electroporation technique, gene transfer into T cells is typically carried out at the beginning of a period of in vitro culture and stimulation. This system leads to more efficient transduction and a greatly expanded number of T cells for subsequent adoptive transfer.
T-cell receptors (TCRs)
The rationale for engrafting high-affinity TCRs is that the endogenous repertoire for TCRs is generally of low affinity when targeting self-TAAs. The assumption is that as such low-affinity TCR chains are unable to control malignancy, increasing the affinity will initiate an effective antitumor response.65 Indeed, there is precedent based on animal studies.66 There are three general approaches to increasing the affinity of TCRs (1) in silico changes to antigen-binding domains, especially CDR367; (2) using combination platforms such as the XPRESIDENT technology that uses mass spectrometry, gene expression profiling, literature-based functional assessment, in vitro human T-cell assays, and bioinformatics to select the most appropriate tumor-associated peptides68; and (3) CARs that recognize peptide in context of restricting HLA molecules.69, 70 The TCR is composed of a complex of six polypeptide chains (α, β, γ, δ, ϵ, and ζ), which connect on the cell surface as part of the immunological synapse to initiate a T-cell activation signal. The α and β chains comprise a heterodimer to form the primary binding domain of the TCR, which recognize intracellularly processed peptides presented on the surface of target cells by classical HLA class I and II molecules. Other constituents of the immunological synapse such as costimulatory and adhesion molecules increase the strength and quality of the TCR-HLA-peptide engagement leading to a fully competent T-cell activation event, defined at a minimum as (1) serial killing, (2) sustained proliferation, (3) Tc1 cytokine production, and (4) protection from activation-induced cell death (AICD). The genetic modification of T cells to enforce expression of TCR has been translated from the bench71 to the bedside. Initial clinical efforts adoptively transferred autologous T cells that had been rendered specific for melanoma.19 The clinical responses were inferior to recipients of (nongenetically modified) TIL. However, these data established the proof-of-principle that T cells could be engineered to target TAAs and provided justification for targeting tumors other than melanoma. They also provided a glimpse of the future as T cells expressing high-affinity TCRs participated in on-target and off-tissue recognition of normal melanin expressing cells in the inner ear and retina.18
The broad human application of T cells genetically modified to express TAA-specific TCR faces two significant challenges. The first is the unwanted mispairing between the introduced αβ TCR and endogenous αβ TCR, which could (1) reduce the number of appropriately paired chains that can recognize HLA-peptide and (2) generate TCR heterodimers that have not undergone thymic selection and tolerance, which could lead to deleterious recognition of normal structures. This has been demonstrated in the mouse72, 73 but has not led to clinical compromise in human trials. Table 3 provides a current list of approaches to eliminate mispairing between introduced and endogenous TCR. The second problem is associated with the affinity of the engrafted TCR. Methods to increase affinity are also not subject to thymic selection and tolerance mechanisms, which, when coupled with an incomplete understanding of their specificity, exposes the recipient to on target but off-tissue side effects. Most approaches currently rely on targeting nonmutated self-antigens, the most promising of which may be gene products from the cancer testis family of genes.22 An affinity-enhanced NY ESO-1-specific and HLA-A2-restricted TCR was tested in patients with melanoma and synovial cell sarcoma and no serious toxicity was seen.22 However, the adoptive transfer of autologous T cells expressing an affinity-enhanced21 HLA-A1-restricted MAGE A3-specific TCR led to lethal cardiac toxicity in two patients owing to off-target recognition of Titin. In another trial,20 in which autologous T cells with affinity-enhanced TCR recognizing MAGE A3 in the context of HLA-A2 were infused in patients with melanoma, significant neurotoxicity from a cross-reactive antigen MAGE A12 present on brain cells occurred; specifically, three patients experienced mental status changes and two lapsed into comas and died. Systemic administration of corticosteroids did not help to reverse the toxicities. While these events illustrate the potency that engineered autologous T cells can display, they highlight the urgent need for improved preclinical systems to uncover off-target adverse events before initiation of clinical trials.
Although TCR-based ACT approaches have great potential, they do have inherent limitations. The HLA-restricted specificity of engineered TCR limits the number of potential patients to those expressing the relevant HLA allele recognized by the T cells. In addition, because tumors often downregulate HLA alleles and interrupt proteasomal processing pathways, antigen escape variants and lack of complete remissions are relevant.
Chimeric antigen receptors (CARs)
Prototypical CARs combine an extracellular single-chain variable fragment (scFv) of an antibody with a transmembrane (TM) domain and intracellular signaling domains derived from molecules involved in T-cell signaling complex. Typically, clinically appealing CARs use the signaling motif from the CD3-ζ chain in combination with signaling domains from molecules involved in T-cell activation or costimulation.85, 86
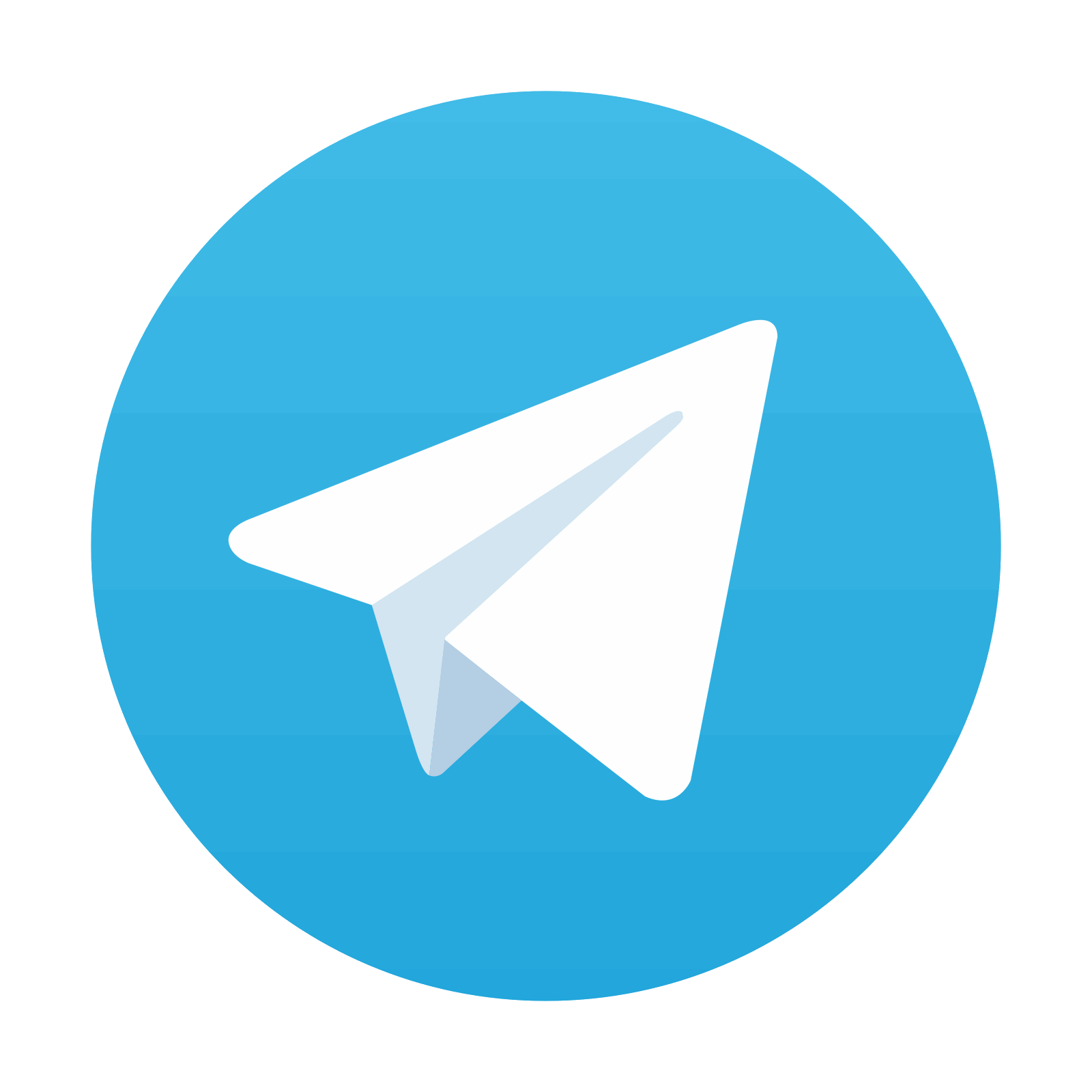
Stay updated, free articles. Join our Telegram channel

Full access? Get Clinical Tree
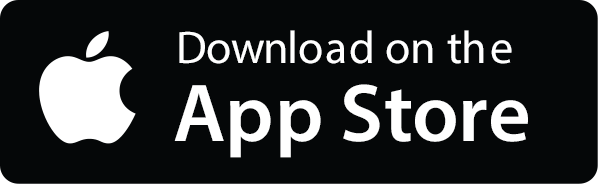
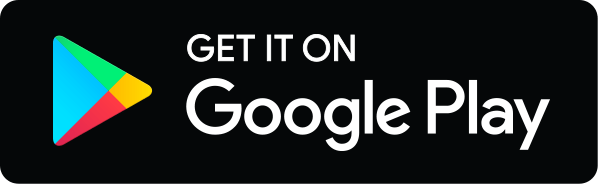