■ Sensitivity is the proportion of persons designated positive by the screening test among all individuals who have the disease: true positive (TP)/(TP + false negative [FN]).
■ Specificity is the proportion of persons designated negative by the screening test among all individuals who do not have the disease: true negative TN/(TN + false positive [FP]).
■ Positive predictive value is the proportion of individuals with a positive screening test who have the disease: (TP)/(TP + FP).
■ Negative predictive value is the proportion of individuals with a negative screening test who do not have the disease: (TN)/(TN + false negative [FN]).2
For a given screening test, sensitivity and specificity are inversely related. For example, as one lowers the threshold for considering a serum prostate-specific antigen (PSA) level to represent a positive screen, the sensitivity of the test increases and more cancers will be detected. This increased sensitivity comes at the cost of decreased specificity (i.e., more men without cancer will have positive screenings tests and, therefore, will be subjected to unnecessary diagnostic procedures).
Some screening tests, such as mammograms, are more subjective and operator dependent than others. For this reason, the sensitivity and specificity of screening mammography varies among radiologists. For a given radiologist, the lower his or her threshold for considering a mammogram to be suspicious, the higher the sensitivity and lower the specificity will be for them. However, mammography can have both a higher sensitivity and higher specificity in the hands of a more experienced versus a less experienced radiologist.
As opposed to sensitivity and specificity, the PPV and NPV of a screening test are dependent on disease prevalence. PPV is also highly responsive to small increases in specificity. As shown in Table 34.2, given a disease prevalence of 5 cases per 1,000 (0.005), the PPV of a hypothetical screening test increases dramatically as specificity goes from 95% to 99.9%, but only marginally as sensitivity goes from 80% to 95%. Given a disease prevalence of only 1 per 10,000 (0.0001), the PPV of the same test is poor even at high sensitivity and specificity. The positive association between breast cancer prevalence and age is the major reason why screening mammography is a better test (higher PPV) for women aged 50 to 59 than for women 40 to 49 years of age.
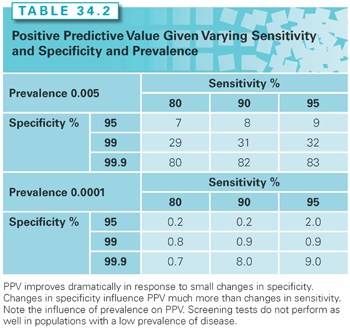
ASSESSING SCREENING TESTS AND OUTCOMES
Screening Test Results
Lead time bias occurs whenever screening results in an earlier diagnosis than would have occurred in the absence of screening. Because survival is measured from the time of diagnosis, an earlier diagnosis, by definition, increases survival. Unless an effective intervention is available, lead time bias has no impact on the natural history of a disease and death will occur at the same time it would have in the absence of early detection (Fig. 34.1).
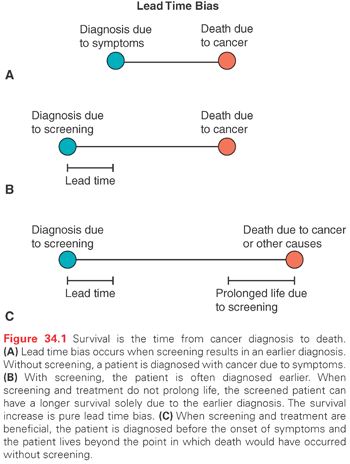
Length bias is a function of the biologic behavior of a cancer. Slower growing, less aggressive cancers are more likely to be detected by a screening test than faster growing cancers, which are more likely to be diagnosed due to the onset of symptoms between scheduled screenings (interval cancers). Length bias has an even greater effect on survival statistics than lead time bias (Fig. 34.2).
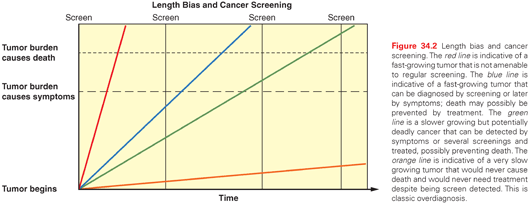
Overdiagnosis is an extreme form of length bias and represents pure harm. It refers to the detection of tumors, often through highly sensitive modern imaging modalities and other diagnostic tests, that fulfill the histologic criteria for malignancy but are not biologically destined to harm the patient (see Fig. 34.2).
There are two categories of overdiagnosis: the detection of histologically defined cancers not destined to metastasize or harm the patient, and the detection of cancers not destined to metastasize or cause harm in the life span of the specific patient. The importance of this second category is illustrated by the widespread practice in the United States of screening elderly patients with limited life expectancies, who are thus unlikely to benefit from early cancer diagnosis.
Overdiagnosis occurs with many malignancies, including lung, breast, prostate, renal cell, melanoma, and thyroid cancers.3 Neuroblastoma provides one of the most striking examples of overdiagnosis.4 Urine vanillylmandelic acid (VMA) testing is a highly sensitive screening test for the detection of this pediatric disease. After screening programs in Germany, Japan, and Canada showed marked increases in the incidence of this disease without a concomitant decline in mortality, it was noticed that nearby areas that did not screen had similar death rates with lower incidence.4,5 It is now appreciated that screen-detected neuroblastomas have a very good prognosis with minimal or no treatment. Many actually regress spontaneously.
Stage shift—i.e., a cancer diagnosis at an earlier stage than would have occurred in the absence of screening—is necessary, but not sufficient, for a screening test to be effective in terms of reducing mortality. Both lead time bias and length bias contribute to this phenomenon. Although it is tempting to speculate that diagnosis at an earlier stage must confer benefit, this is not necessarily the case. For example, a substantial proportion of men treated with radical prostatectomy for what appears to be a localized prostate cancer relapse after undergoing surgery. Conversely, some men who are treated with definitive therapy would never have gone on to develop metastatic disease in the absence of treatment.
Selection bias occurs when enrollees in a clinical study differ from the general population. In fact, people who voluntarily participate in clinical trials tend to be healthier than the general population, perhaps due to a greater interest in health and health-care research. Screening studies tend to enroll individuals healthier than the general population. This so-called healthy volunteer effect6,7 can introduce a powerful bias if not adequately controlled for by randomization procedures.
Assessing Screening Outcomes
The usual primary goal of cancer screening is to reduce mortality from the disease in question (a reduction in disease-specific mortality). Screening studies generally do not have sufficient statistical power to assess the impact of screening for a specific malignancy on overall mortality. (Lung cancer screening provides an exception to this rule; see the following.) As discussed previously, the fact that a screening test increases the percentage of people diagnosed with early stage cancer and decreases that of late stage cancer (stage shift) is not equivalent to proof of mortality reduction. Further, due to the healthy volunteer effect, case control and cohort studies cannot provide definitive evidence of mortality benefit. Prospective, randomized clinical trials are required to address this issue. In such trials, volunteers are randomized to be screened or not and are then followed longitudinally to determine if there is a difference in disease-specific or overall mortality.
A reduction in mortality rates or in the risk of death is often stated in terms of relative risk. However, this method of reporting may be misleading. It is preferable to report both the relative and absolute reduction in mortality. For example, the European Randomized Study of Screening for Prostate Cancer (ERSPC) showed that screening reduced the risk of prostate cancer death by 20%. However, this translates into only 1 prostate cancer death averted per 1,000 men screened (5 prostate cancer deaths per 1,000 men not screened versus 4 prostate cancer deaths per 1,000 men screened) and a relatively modest lifetime reduction in the absolute risk of prostate cancer death of only 0.6%, from 3.0% to 2.4%.8
PROBLEMS WITH RANDOMIZED TRIALS
It is important to acknowledge that even prospective, randomized trials can have serious methodologic shortcomings. For example, imbalances caused by flaws in the randomization scheme can prejudice the outcome of a trial. Other flaws include so-called drop-in or contamination, in which some participants on the control arm get the intervention. Patients on the intervention arm may also drop out of the study. Both drop-ins and drop-outs reduce the statistical power of a clinical trial.
In the United States, it is now considered standard to obtain informed consent before randomization takes place. However, there have been several published studies that randomized participants from rosters of eligible subjects such as census lists. In these trials, informed consent was obtained after randomization and only among those randomized to the screening arm of the study. Those randomized to the control arm were not contacted, and indeed, did not know they were in a clinical trial. They were followed through national death registries. Although the study was analyzed on an intent-to-screen basis, this method can still introduce biases. For example, only patients on the intervention arm had access to the screening facility and staff for counseling and treatment if diagnosed; those in the control group were more likely to be treated in the community as opposed to high-volume centers of excellence and were less likely to be treated with surgery and more likely to be treated with hormones alone than those on the screened arm. The study arms would also tend to differ in their knowledge of the disease, which may contribute to an overestimate of the benefits of a screening test.9
Virtually every screening test is a balance between known harms and potential benefits. The most important risk of screening is the detection and subsequent treatment of a cancer that would never have come to clinical detection or harmed the patient in the absence of screening (i.e., overdiagnosis and overtreatment). Treatment can cause emotional and physical morbidity and even death.10 Even when screening has a net mortality benefit, there can be considerable harm. For example, in the recent randomized trial of spiral lung computed tomography (CT) scan, approximately 27,000 current smokers and former smokers were given three annual low-dose CT scans. More than 20% had a positive screening CT scan, necessitating further testing. About 1,000 subsequently underwent invasive diagnostic procedures and 16 deaths were reported within 60 days of the procedure.11 It is not known how many of these deaths were directly related to the screening.
It can be dangerous to extrapolate estimates of benefit from one population to another. In particular, studies showing that a radiographic test is beneficial to average risk individuals may not mean that it is beneficial to a population at high risk, and vice versa. For example, women at high risk for breast cancer due to an inherited mutation of a DNA repair gene may be at higher risk for radiation-induced cancer from mammographies compared to the general population; a screening test (e.g., spiral lung CT scan), shown to be efficacious in a high-risk population of heavy smokers may result in net harm if applied to a low or average risk population.
SCREENING GUIDELINES AND RECOMMENDATIONS
A number of organizations develop cancer screening recommendations or guidelines. These organizations use varying methods. The Institute of Medicine (IOM) has released two reports to establish standards for developing trustworthy clinical practice guidelines and conducting systematic evidence reviews that serve as their basis.12,13 The U.S. Preventive Services Task Force (USPSTF) and the American Cancer Society (ACS) are two organizations that issue respected and widely used cancer guidelines (Table 34.3). Both have changed their methods to comply with the IOM standards.
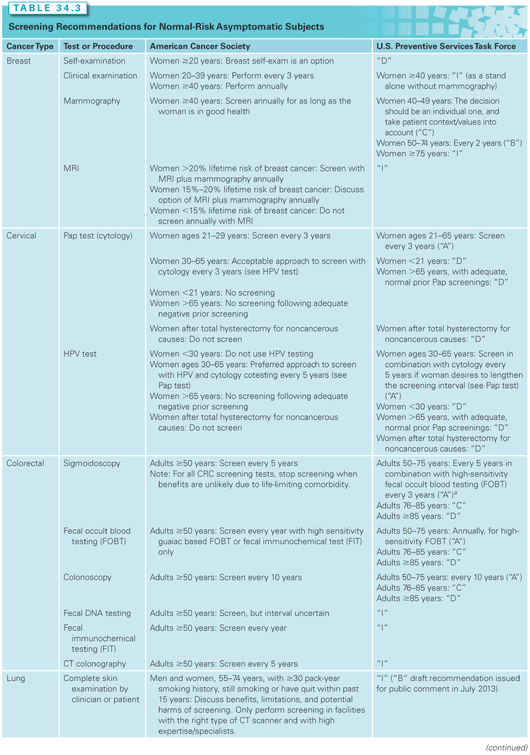
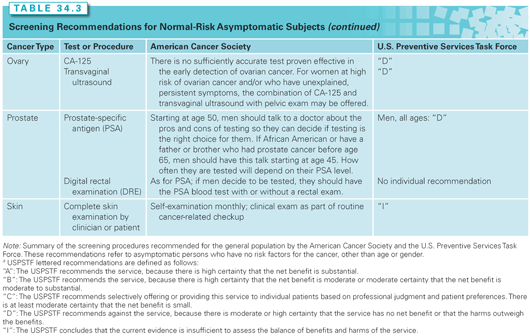
The USPSTF is a panel of experts in prevention and evidence-based medicine.14 They are primary care providers specializing in internal medicine, pediatrics, family practice, gynecology and obstetrics, nursing, and health behavior. The task force process begins by conducting an extensive structured scientific evidence review. The task force then develops recommendations for primary care clinicians and health-care systems. They adhere to some of the highest standards for recommending a screening test. They are very much concerned with the question, “Does the evidence supporting a screening test demonstrate that the benefits outweigh its harms?”
The ACS guidelines date back to the 1970s. The current process for making guidelines involves commissioning academics to do an independent systematic evidence review. A single generalist group digests the evidence review, listens to public input, and writes the guidelines. The ACS panel tries to clearly articulate the benefits, limitations, and harms associated with a screening test.15
Mammographies, clinical breast examinations (CBE) by a health-care provider, and breast self-examinations (BSE) have long been advocated16 for the early detection of breast cancer. In recent years, ultrasound, magnetic resonance imaging (MRI), and other technologies have been added to the list of proposed screening modalities.
Mammographic screening was first advocated in the 1950s. The Health Insurance Plan (HIP) Study was the first prospective, randomized clinical trial to formally assess its value in reducing death from breast cancer. In this study, started in 1963, about 61,000 women were randomized to three annual mammograms with clinical breast examination versus no screening, which was the standard practice at that time. HIP first reported that mammography reduced breast cancer mortality by 30% at about 10 years after study entry. With 18 years of follow-up, those in the screening arm had a 25% lower breast cancer mortality rate.16
Nine additional prospective randomized studies have been published. These studies provide the basis for the current consensus that screening women 40 to 75 years of age does reduce the relative risk of breast cancer death by 10% to 25%. The 10 studies demonstrate that the risk–benefit ratio is more favorable for women over 50 years of age. Mammography has also been shown to be operator dependent, with better performance characteristics (higher sensitivity and specificity and lower FP rates) reported by high-volume centers (Table 34.4).
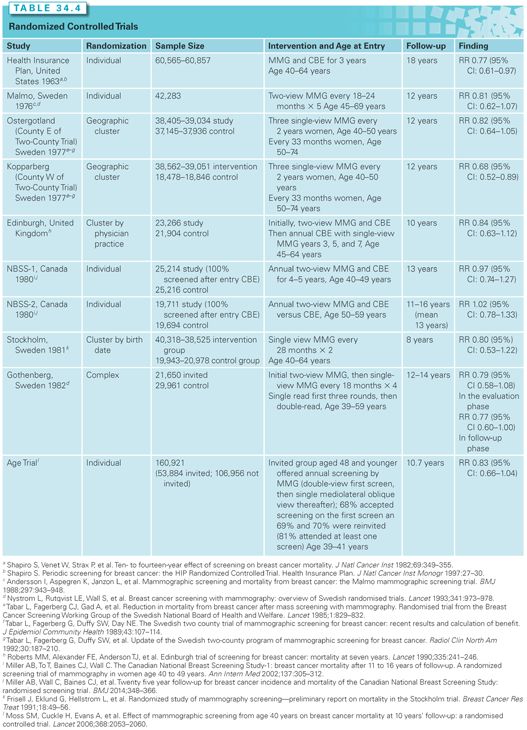
It is important to note that every one of these studies has some flaws and limitations. They vary in the questions asked and their findings. The Canadian screening trial suggests mammographies and clinical breast examinations do not decrease risk of death for woman aged 40 to 49 and that mammographies add nothing to CBEs for women age 50 to 59 years.17 On the other extreme, the Kopparberg Sweden study suggests that mammographies are associated with a 32% reduction in the risk of death for women aged 40 to 74 years.18
To date, no study has shown that BSEs decrease mortality. BSEs have been studied in two large randomized trials. In one, approximately 266,000 Chinese women were randomized to receive intensive BSE instruction with reinforcements and reminders compared to a control group receiving no instruction on BSE. At 10 years of follow-up, there was no difference in mortality, but the intervention arm had a significantly higher incidence of benign breast lesions diagnosed and breast biopsies preformed. In the second study, 124,000 Russian women were randomized to monthly BSEs versus no BSEs. There was no difference in mortality rates, despite the BSE group having a higher proportion of early stage tumors and a significant increase in the proportion of cancer patients surviving 15 years after diagnosis.
Ultrasonography is primarily used in the diagnostic evaluation of a breast mass identified by palpation or mammography. There is little evidence to support the use of ultrasound as an initial screening test. This modality is highly operator dependent and time consuming, with a high rate of FP findings.19 An MRI is used for screening women at elevated breast cancer risk due to BRCA1 and BRCA2 mutations, Li-Fraumeni syndrome, Cowden disease, or a very strong family history. MRI is more sensitive but less specific than mammography, leading to a high FP rate and more unnecessary biopsies, especially among young women.20 The impact of MRI breast screening on breast cancer mortality has not yet been determined.
Thermography, an infrared imaging technology, has some advocates as a breast cancer screening modality despite a lack of evidence from several small cohort studies.21 Nipple aspirate cytology and ductal lavage have also been suggested as possible screening methods. Both should be considered experimental at this time.22
Effectiveness of Breast Cancer Screening
Breast cancer screening has been associated with a dramatic rise in breast cancer incidence. At the same time, there has been a dramatic decrease in breast cancer mortality rates. However, in the United States and Europe, incidence-by-stage data show a dramatic increase in the proportion of early stage cancers without a concomitant decrease in the incidence of regional and metastatic cancers.23 These findings are at odds with the clinical trials data and raise questions regarding the extent to which early diagnosis is responsible for declining breast cancer mortality rates.
From 1976 to 2008, the incidence of early-stage breast cancer for American women aged 40 and older increased from 112 to 234 per 100,000. This is a rise of 122 cases per 100,000, whereas the absolute decrease in late-stage cancers was only 8 cases per 100,000 (from 102 to 94 cases per 100,000). These data raise questions regarding the magnitude of benefit, as well as the potential risks, of breast cancer screening. The discrepancy between the magnitude of the increase of early disease and the decrease of late-stage cancer and cancer mortality suggests that a proportion of invasive breast cancers diagnosed by screening represents overdiagnosis. These data suggest that overdiagnosis accounts for up to 31% of all breast cancers diagnosed by screening.24 Others have estimated that up to 50% of breast cancers detected by screening mammography are overdiagnosed cancers. In an exhaustive review of the screening literature, a panel of experts concluded that overdiagnosis does exist and estimated it to be 11% to 19% of breast cancers diagnosed by screening.25
A confounding factor with regard to the mortality benefits of breast cancer screening is the improvement that has occurred in breast cancer treatment over this period of time. The effects of the advances in therapy are supported by cancer modeling studies. Indeed, the Cancer Intervention and Surveillance Modeling Network (CISNET), supported by the U.S. National Cancer Institute (NCI), has estimated that two-thirds of the observed breast cancer mortality reduction is attributable to modern therapy, rather than to screening.26
Questions have also been raised regarding the quality of the randomized screening trials that demonstrated the mortality benefits of mammography and clinical breast examination because these trials suffered from a variety of design flaws. In some, randomization methods were suboptimal, others reported varying numbers of participants over the years, and still others had substantial contamination (drop-ins). Perhaps more importantly, most trials were started and concluded before the widespread use of more advanced mammographic technology, before the modern era of adjuvant therapy, and before the advent of targeted therapy.
Although randomized control trials (RCT) remain the gold standard for assessing the benefits of a clinical intervention, they cannot take into account improvements in both treatment and patient awareness that occurred over time. For this reason, observational and modeling studies can provide important, complementary information.
One systematic review of 17 published population-based and cohort studies compared breast cancer mortality in groups of women aged 50 to 69 years who started breast cancer screening at different times. Although these studies are subject to methodologic limitations, only four suggested that breast cancer screening reduced the relative risk of breast cancer mortality by 33% or more and five suggested no benefit from screening. The review concluded that breast cancer screening likely reduces the risk of breast cancer death by no more than 10%.27
Even with these limitations, a systematic review of the data sponsored by the USPSTF concluded that regular mammography reduces breast cancer mortality in women aged 40 to 74 years.28 The task force also concluded that the benefits of mammography are most significant in women aged 50 to 74 years.
Screening Women Age 40 to 49
Experts disagree about the utility of screening women in their forties. In the HIP Randomized Control Trial, women who entered at age 40 to 49 years had a mortality benefit at 18 years of follow-up. However, to a large extent, the mortality benefit among those aged 45 to 49 years at entry was driven by breast cancers diagnosed after they reached age 50 years.16
Mammography, like all screening tests, is more efficient (higher PPV) for the detection of disease in populations with higher disease prevalence (see Table 34.2). Mammography is, therefore, a better test in women age 50 to 59 years than it is among women age 40 to 49 years because the risk of breast cancer increases with age. Mammography is also less optimal in women age 40 to 49 years compared to women 50 to 59 years of age for the following reasons:
■ A larger proportion have increased breast density, which can obscure lesions (lower sensitivity).
■ Younger women are more likely to develop aggressive, fast-growing breast cancers that are diagnosed between regular screening visits. By definition, these interval cancers are not screen detected.29
The USPSTF meta-analysis of eight large randomized trials suggested a 15% relative reduction in mortality (relative risk [RR], 0.85; 95% confidence interval [CI], 0.75 to 0.96) from mammography screening for women aged 40 to 49 years after 11 to 20 years of follow-up. This is equivalent to a needing to invite 1,904 women to screenings over 10 years to prevent one breast cancer death. Studies, however, show that more than half of women aged 40 to 49 years screened annually over a 10-year period will have an FP mammogram necessitating further evaluation, often including biopsy. In addition, estimates of overdiagnosis in this group range from 10% to 40% of diagnosed invasive cancers.30
In an effort to decrease FP rates, some have suggested screening every 2 years rather than yearly. Comparing biennial with annual screening, the CISNET Model consistently shows that biennial screening of women ages 40 to 70 only marginally decreases the number of lives saved while halving the false positive rate.29 Notably, the Swedish two-county trial, which had a planned 24-month screening interval (the actual interval was 33 months) reported one of the greatest reductions in breast cancer mortality among the RCTs conducted to date.
Screening Women at High Risk
There is interest in creating risk profiles as a way of reducing the inconveniences and harms of screening. It might be possible to identify women who are at greater risk of breast cancer and refocus screening efforts on those most likely to benefit.
Risk factors for breast cancer include the following:
■ Extremely dense breasts on mammography or a first-degree relative with breast cancer are each associated with at least a twofold increase in breast cancer risk
■ Prior benign breast biopsy, second-degree relatives with breast cancer, or heterogeneously dense breasts each increase risk 1.5- to twofold
■ Current oral contraceptive use, nulliparity, and age at first birth 30 years and older increase risk 1- to 1.5-fold.31
Importantly, these are risk factors for breast cancer diagnosis, not breast cancer mortality. Few studies have assessed the association between these factors and death from breast cancer; however, reproductive factors and breast density have been shown to have limited influence on breast cancer mortality.32,33
Genetic testing for BRCA1 and BRCA2 mutations and other markers of breast cancer risk has identified a group of women at high risk for breast cancer. Unfortunately, when to begin and the optimal frequency of screening have not been defined. Mammography is less sensitive at detecting breast cancers in women carrying BRCA1 and BRCA2 mutations, possibly because such cancers occur in younger women in whom mammography is known to be less sensitive.
MRI screening may be more sensitive than mammography in women at high risk, but specificity is lower. MRIs are associated with both an increase in FP and an increase in the detection of smaller cancers, which are more likely to be biologically indolent. The impact of MRIs on breast cancer mortality with or without concomitant use of mammographies has not been evaluated in a randomized controlled trial.
Breast Density
It is well established that mammogram sensitivity is lower in women with heterogeneously dense or very dense breasts.29,32 However, at this time, there are no clear guidelines regarding whether or how screening algorithms should take breast density into account.
In the American College of Radiology’s Imaging Network (ACRIN)/NCI 666 Trial, breast ultrasound was offered to women with increased mammographic breast density and, if either test was positive, they were referred for a breast biopsy.34 The radiologists performing the ultrasounds were not aware of the mammographic findings. Mammography detected 7.6 cancers per 1,000 women screened; ultrasound increased the cancer detection rate to 11.8 per 1,000. However, the PPV for mammography alone was 22.6%, whereas the PPV for mammography with ultrasound was only 11.2%.
It has yet to be determined whether supplemental imaging reduces breast cancer mortality in women with increased breast density. Although it continues to be strongly advocated by some, systematic reviews have concluded that the evidence is currently insufficient to recommend for or against this approach.35 There are also a number of barriers to supplemental imaging, including inconsistent insurance coverage, lack of availability in many communities, concerns about cost-effectiveness (particularly with regard to MRI), and the increased FP rate associated with supplemental imaging leading to unnecessary biopsies.36
Newer technologies may improve screening accuracy for women with dense breasts. Compared to conventional mammography, full field digital mammography (FFDM) appears to have less FPs. This could reduce the number of women needing supplemental imaging and biopsies.37 Digital breast tomosynthesis (DBT) uses x-rays and a digital detector to generate cross-sectional images of the breasts. Data are limited, but compared to mammograms, DBT appears to offer increased sensitivity and a reduction in the recall rates.38 Another potential supplementary imaging modality currently under investigation is three-dimensional (3-D) automated breast ultrasound, and having screening ultrasounds performed by technologists rather than radiologists.
Ductal Carcinoma In Situ
The incidence of noninvasive ductal carcinoma in situ (DCIS) has increased more than fivefold since 1970 as a direct consequence of widespread screening mammographies.39 DCIS is a heterogeneous condition with low- and intermediate-grade lesions taking a decade or more to progress. Nevertheless, women with this diagnosis are uniformly subjected to treatment. A better understanding of this entity and an increased ability to predict its biologic behavior may enable more judicious, personalized treatment of DCIS.
There is little evidence that the early detection and aggressive treatment of low- and intermediate-grade DCIS reduces breast cancer mortality. The standard of care for all grades of DCIS is lumpectomy with radiation or mastectomy, followed by tamoxifen for 5 years. Interestingly, patterns of care studies indicate that mastectomy rates are increasing,40 and that women are more often choosing double mastectomies for the treatment of DCIS.41 Genomic characterization will hopefully lead to the identification of a subset of noninvasive cancers that can be treated less aggressively or even observed.
Harms
The harms and disadvantages of mammography screening include overdiagnosis, FP tests, FN tests, and the possibility of radiation-induced breast cancer.
The fact that mammography screening has increased the incidence of localized disease without a significant change in metastatic disease at the time of diagnosis suggests that there is some degree of overdiagnosis. The risk of overdiagnosis is greatest at the first screening3 and varies with patient age, tumor type, and grade of disease.
FP screening tests lead to substantial inconvenience and anxiety in addition to unnecessary invasive biopsies with their attendant complications. In the United States, about 10% of all women screened for breast cancer are called back for additional testing, and less than half of them will be diagnosed with breast cancer.39 The risk of a FP mammogram is greater for women under the age of 50.37
FN tests delay diagnosis and provide false reassurance. They are more common in younger women and in women with dense breasts.42,43 Certain histologic subtypes are also more difficult to see on mammogram. Mucinous and lobular tumors and rapidly growing tumors tend to blend in with normal breast architecture.44
A typical screening mammogram provides approximately 4 mSv of radiation. It has been estimated that annual mammographies will cause up to 1 case of breast cancer per 1,000 women screened from age 40 to age 80 years. Radiation exposure at younger ages causes a greater risk of breast cancer.45 There is also concern that ionizing radiation from mammographies might disproportionately increase the breast cancer risk for women with certain BRCA1 or BRCA2 mutations, because these genes are related to DNA repair.46
Recommendations
Women at Average Risk
The ACS and most other medical groups recommend that average risk women undergo a CBE every 3 years starting at age 20 and that women 40 years of age and over should undergo CBEs and screening mammograms annually. Women should be informed of the benefits, limitations, and harms associated with breast cancer screening. A mammography will not detect all breast cancers, and some breast cancers detected with mammographies may still have a poor prognosis. The harms associated with breast cancer screening also include the potential for FP results, causing substantial anxiety. When abnormal findings cannot be resolved with additional imaging, a biopsy is required to rule out the possibility of breast cancer. A majority of biopsies are benign. Finally, some breast cancers detected by a mammography may be biologically indolent, meaning they would not have caused a problem or have been detected in a woman’s lifetime had she not undergone a mammography.
The USPSTF, the American College of Physicians, and the Canadian Task Force on the Periodic Health Examination recommend routine screening beginning at age 50 years.30,47,48 For women aged 40 to 49 years of age, these groups advise physicians to enter into a discussion with the patient. The physician and patient should take into account individual risks and concerns before deciding to screen.47
An Advisory Committee on Cancer Prevention in the European Union recommends that women between the ages of 50 and 69 years be offered mammogram screening in an organized screening program with quality assurance.49 This committee says women aged 40 to 49 years should be advised of the potential harms of screening and, if mammographic screening is offered, it should be performed with strict quality standards and double reading.
Women at High Risk
The ACS has issued guidelines for women who were known or likely carriers of a BRCA mutation and other rarer high-risk genetic syndromes, or at high risk for other reasons.50 Annual screening mammographies and MRIs starting at age 30 is recommended for women:
■ With a known BRCA mutation
■ Who are untested but have a first-degree relative with a BRCA mutation
■ Who had been treated with radiation to the chest for Hodgkin disease
■ Who have an approximately 20% to 25% or greater lifetime risk of breast cancer based on specialized breast cancer risk estimation models.
Colorectal cancer screening with the rigid sigmoidoscope dates back to the late 1960s. The desire to examine the entire colon led to the use of a barium enema and the development of fecal occult blood tests. With the development of fiber optics, flexible sigmoidoscopies and, later, colonoscopies were employed. Today, fecal occult blood testing (FOBT), stool DNA testing, flexible sigmoidoscopies, colonoscopies, and CT colonographies and, occasionally, barium enemas are all used in colorectal cancer screening. MRI colonoscopy is in development.
Screening examinations of the colon and rectum can find cancer early, but also find precancerous polyps. Randomized trials have demonstrated that endoscopic polypectomies reduce the incidence of colorectal cancer by about 20%.51–53
FOBT was the first colorectal screening test studied in a prospective randomized clinical trial. The Minnesota Colon Cancer Control Study randomized 46,551 adults to one of three arms: annual FOBTs, biennial screening, or usual care. A rehydrated guaiac test was used. With 13 years of follow-up, the annual screened arm had a 33% relative reduction in colorectal cancer mortality compared to the usual care group.54 At 18 years of follow-up, the biennially screened group had a 21% reduction in colorectal cancer mortality.55 This study would subsequently show that stool blood testing was associated with a 20% reduction in colon cancer incidence.51 These results were confirmed by two other randomized trials.56,57 A reduction in colon cancer–specific mortality persisted in the Minnesota trial through 30 years of follow-up. Overall mortality was not affected.
Rehydration increases the sensitivity of FOBT at the expense of lowering specificity.58 Indeed, rehydrated specimens have a very high FP rate. Overall, 1% to 5% of FOBTs are positive, but only 2% to 10% of those with a positive FOBT have cancer.
Fecal immunochemical tests (FIT) are stool tests that do not react to hemoglobin in dietary products. They appear to have higher sensitivity and specificity for colorectal cancer when compared to nonrehydrated FOBT tests.59
Fecal DNA testing is an emerging modality. These tests look for DNA sequences specific to colorectal polyps and colorectal cancer. They may have increased sensitivity and specificity compared to FOBT. Although fecal DNA tests appear to find cancer, the body of evidence on their ability to reduce colorectal cancer mortality is limited due to a lack of study. This test has been intermittently available.
Flexible sigmoidoscopies are, of course, limited to an examination of the rectum and sigmoid colon. A prospective randomized trial of once-only flexible sigmoidoscopies demonstrated a 23% reduction in colorectal cancer incidence and a 31% reduction in colorectal cancer mortality after a median 11.2 years of follow-up.60 In the NCI’s Prostate, Lung, Colorectal, and Ovarian Cancer Screening Trial (PLCO), there was a 21% reduction in colorectal cancer incidence and a 26% reduction in colorectal cancer mortality with two sigmoidoscopies done 3 to 5 years apart compared with the usual care group after a median follow-up of 11.9 years.53 In both studies, there was no effect on proximal lesions (i.e., right and transverse colon) due to the limited reach of the scope. It is estimated that flexible sigmoidoscopies can find 60% to 80% of cancers and polyps found by colonoscopies.61
In two meta-analyses of five randomized controlled trials of sigmoidoscopies, there was an 18% relative reduction in colorectal cancer incidence and a 28% relative reduction in colorectal cancer mortality.62,63 Participants ranged in age from 50 to 74 years. Follow-up ranged from 6 to 13 years.
The colonoscopy has become the preferred screening method of many, although there have been no prospective, randomized trials of colonoscopy screening. A positive FOBT, FIT, fecal DNA test, or sigmoidoscopy warrants a follow-up diagnostic colonoscopy. Perhaps the best support for colonoscopy screening is indirect evidence from the Minnesota Colon Cancer Control Study, which required that all participants with a positive stool blood test have diagnostic imaging of the entire colon. In the Minnesota study, more than 40% of those screened annually eventually received a colonoscopy. One can also make the argument that the sigmoidoscopy studies indirectly support the efficacy of colonoscopy screening, although it can be argued that embryologic and epidemiologic evidence indicate that the right and left colon are biologically distinct and, therefore, the mortality benefits from sigmoidoscopies do not constitute proof that a colonoscopy would similarly reduce mortality from proximal colon lesions.
In studies involving repeat colonoscopies by a second physician, 21% of all adenomas were missed, including 26% of 1 to 5 mm adenomas and 2% of adenomas 10 mm or more in length.64 Other limitations of colonoscopies include the inconvenience of the bowel preparation and the risk of bowel perforation (about 3 out of 1,000 procedures, overall, with nearly all of the risk among patients who undergo colonoscopic polypectomies). The cost of the procedure and the limited number of physicians who can do the procedure are also of concern.
A CT colonography or virtual colonoscopy allows a physician to visually reproduce the endoscopic examination on a computer screen. A CT colonography involves the same prep as a colonoscopy, but is less invasive. It might have a higher compliance rate. In experienced hands, the sensitivity of a CT colonography for the detection of polyps ≥6 mm appears to be comparable to that of a colonoscopy. In a meta-analysis of 30 studies, 2-D and 3-D CT colonographies performed equally well.65
The disadvantages of a CT colonography include the fact that it requires a colonic prep and a finding on CT requires a follow-up diagnostic colonoscopy. The rate of extracolonic findings of uncertain significance is high (~15% to 30%), and each one must be evaluated, thereby contributing to additional expense and potential morbidity. The long-term, cumulative radiation risk of repeated colonography screenings is also a concern.
Current Recommendations
The ACS, the American College of Gastroenterology, the American Gastroenterological Association, the American Society for Gastrointestinal Endoscopy, and the American College of Radiology have issued joint colorectal cancer guidelines. These groups consider FOBT, FIT, rigid and flexible sigmoidoscopies, colonoscopies, and CT colonographies to all be reasonable screening methodologies.
They recommend the following: (1) Screening modalities be chosen based on personal preference and access, and (2) average risk adults should begin colorectal cancer screening at age 50 years with one of the following options:
1. Annual high sensitivity FOBT or FIT
2. A flexible sigmoidoscopy every 5 years
3. A colonoscopy every 10 years
4. A double contrast barium enema every 5 years
5. A CT colonography every 5 years
No test is of unequivocal superiority. Patient preferences should be incorporated into screening in order to increase compliance. The guidelines also stress that a single screening examination is far from optimal and that patients should be in a program of regular screening.
Although some colorectal cancers are diagnosed in persons under the age of 50 years, screening persons age 40 to 49 years has low yield.66 The guidelines also state that patients with less than a 10-year life expectancy should not be screened.
The USPSTF issued colorectal cancer screening guidelines in 2008.67 The guidelines were based on a systematic literature review and decision models. The task force concluded that three screening strategies appear to be equivalent for adults age 50 to 75 years:
1. An annual FOBT with a sensitive test
2. A flexible sigmoidoscopy every 5 years, with a sensitive FOBT every 3 years
3. A colonoscopy every 10 years
The task force recommends that patients age 76 to 85 years be evaluated individually for screening. They found “insufficient evidence” to recommend CT colonographies or fecal DNA testing.
Patients at Increased Risk of Colorectal Cancer
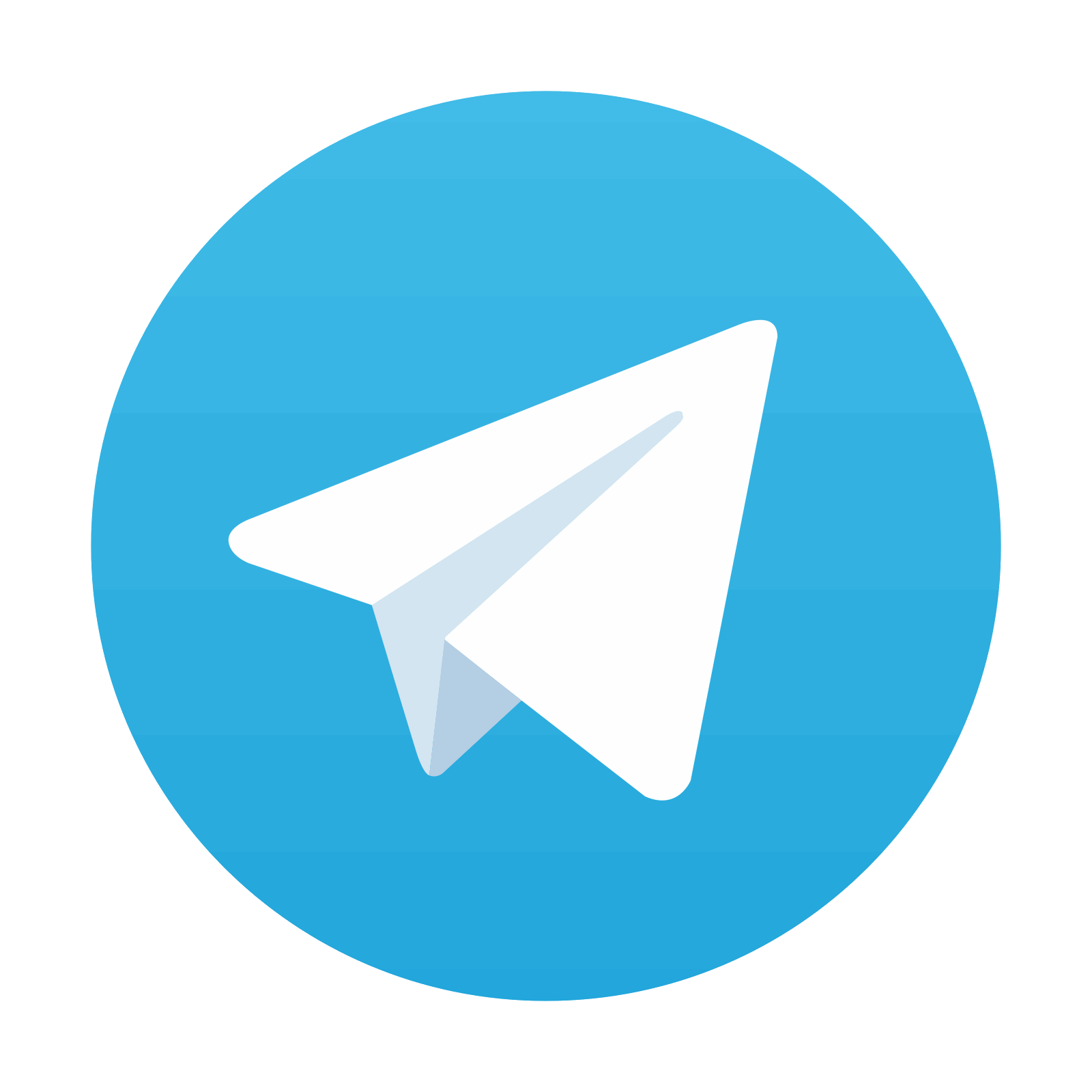
Stay updated, free articles. Join our Telegram channel

Full access? Get Clinical Tree
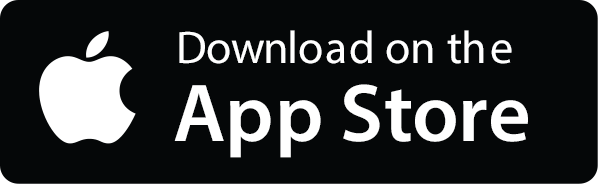
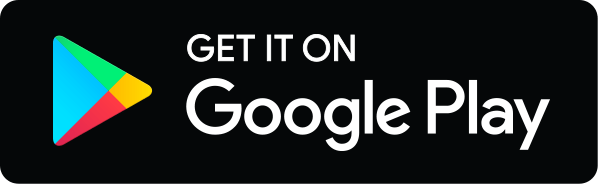