Objectives
- 1.
Describe the structure and synthesis of parathyroid hormone (PTH), the regulation of PTH secretion, and the nature of the PTH receptor.
- 2.
Describe the structure and synthesis of 1,25-dihydroxyvitamin D, the regulation of 1,25-dihydroxyvitamin D production, and the receptor for 1,25-dihydroxyvitamin D.
- 3.
Discuss the roles of the gastrointestinal (GI) tract, bone, and kidneys in calcium (Ca 2+ )/phosphate (Pi) homeostasis.
- 4.
Discuss the actions of calcitonin, PTH–related peptide (PTHrP), fibroblast growth factor-23 (FGF23), and gonadal and steroid hormones on Ca 2+ /Pi metabolism.
- 5.
Discuss the pathophysiology associated with imbalances in PTH and 1,25-dihydroxyvitamin D.
Calcium ( Ca 2+ ) and phosphate (Pi ) are essential to human life, playing important structural roles in hard tissues (i.e., bones and teeth) and important regulatory roles in metabolic and signaling pathways. The two primary sources of circulating Ca 2+ and Pi are the diet and the skeleton ( Fig. 4.1 ).
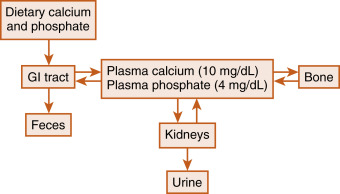
Two hormones, 1,25-dihydroxyvitamin D (also called calcitriol ) and parathyroid hormone (PTH) , regulate intestinal absorption of Ca 2+ and Pi and the release of Ca 2+ and Pi into the circulation during bone resorption. The primary processes for removal of Ca 2+ and Pi from the blood are renal excretion and bone formation (see Fig. 4.1 ), and 1,25-dihydroxyvitamin D and PTH regulate these processes as well. Other hormones and paracrine growth factors, including the rapidly emerging role of fibroblast growth factor-23 (FGF23), also have clinical relevance to Ca 2+ and Pi homeostasis.
Calcium and Phosphorus are Important Dietary Elements That Play Many Crucial Roles in Cellular Physiology
Calcium is an essential dietary element. In addition to getting calcium from the diet, humans contain a vast store (>1 kg) of calcium in their bones, which can be called on to maintain normal circulating levels of calcium in times of dietary restriction and during the increased demands of pregnancy and nursing. Circulating calcium exists in three forms ( Table 4.1 ): free ionized calcium (Ca 2+ ), protein-bound calcium, and calcium complexed with anions (e.g., phosphates, bicarbonate, citrate). The ionized form represents about 50% of circulating calcium, and because this form is so critical to many cellular functions, Ca 2+ levels in both extracellular and intracellular compartments are tightly controlled (see Chapter 1 for discussion of Ca 2+ -dependent signaling pathways). Circulating Ca 2+ is under direct hormonal control and is normally maintained in a relatively narrow range. Either too little Ca 2+ ( hypocalcemia ; total serum Ca 2+ < 8.5 mg/dL [2.1 mM]) or too much Ca 2+ ( hypercalcemia ; total serum Ca 2 + > 10.5 mg/dL [2.6 mM]) in the blood can lead to a broad range of pathophysiologic changes, including neuromuscular dysfunction, central nervous system dysfunction, renal insufficiency, calcification of soft tissue, and skeletal pathologies.
Ion | Concentration (mg/dL) | Ionized (%) | Protein Bound (%) | Complexed (%) |
---|---|---|---|---|
Ca 2+ ∗ | 10 | 50 | 45 | 5 |
Pi | 4 | 84 | 10 | 6 |
∗ Ca 2+ is bound (i.e., complexed) to various anions in the plasma, including <SPAN role=presentation tabIndex=0 id=MathJax-Element-1-Frame class=MathJax style="POSITION: relative" data-mathml='HCO3−’>HCO−3HCO3−
HCO 3 −
, citrate, Pi, and <SPAN role=presentation tabIndex=0 id=MathJax-Element-2-Frame class=MathJax style="POSITION: relative" data-mathml='SO4−’>SO−4SO4−
SO 4 −
. Pi is complexed to various cations, including Na + and K + .
Phosphorus is also an essential dietary element and is stored in large quantities in bone complexed with calcium. In the blood at physiologic pH, inorganic phosphate (Pi) exists as hydrogen phosphate (HPO 4 2- ) or dihydrogen phosphate (H 2 PO 4 – ). Most circulating Pi is in the ionized form, but some Pi (<20%) circulates as a protein-bound form or complexed with cations (see Table 4.1 ). Phosphorus also exists as pyrophosphate (two Pi groups in a covalent linkage), an important inhibitor of mineralization. Unlike Ca 2+ , phosphate is incorporated covalently as single or multiple phosphate groups into many molecules, and consequently, soft tissues contain about 10-fold more phosphate than Ca 2+ . This means that significant tissue damage (e.g., crush injury with massive muscle cell death) can result in hyperphosphatemia , which can then complex with Ca 2+ to cause acute hypocalcemia. Phosphate represents a key intracellular component. Indeed, it is the high-energy phosphate bonds of adenosine triphosphate (ATP) that maintain life. Phosphorylation and dephosphorylation of proteins, lipids, second messengers, and cofactors represent key regulatory steps in numerous metabolic and signaling pathways, and phosphate also serves as the backbone for nucleic acids.
Physiologic Regulation of Calcium and Phosphate: Parathyroid Hormone, 1,25-Dihydroxyvitamin D, and Fgf23
PTH and 1,25-dihydroxyvitamin D have long been recognized as the physiologically important hormones that are dedicated to the maintenance of normal blood Ca 2+ and Pi levels in humans. As such, they are referred to as calciotropic hormones . The structure, synthesis, and secretion of these two hormones and their receptors will be discussed here. In the following section, the detailed actions of PTH and 1,25-dihydroxyvitamin D on the three key sites of Ca 2+ /Pi homeostasis (i.e., gut, bone, and kidney) will be discussed. The emerging role of FGF23 in the regulation of Pi metabolism and its interaction with PTH and 1,25-dihydroxyvitamin D will then be addressed.
Parathyroid Hormone
PTH is a key hormone that maintains serum calcium levels and protects against a hypocalcemic challenge. The primary targets of PTH are bone and kidneys. PTH also functions in a positive feed-forward loop by stimulating 1,25-dihydroxyvitamin D production.
Parathyroid Glands
The parathyroid glands develop from the endodermal lining of the third and fourth branchial pouches. They usually develop into four glands, with the two superior glands derived from the fourth branchial pouch and the two inferior glands from the third. The embryonic anlage of the parathyroids is associated with the caudal migration of the thyroglossal duct, so the parathyroid glands usually become situated on the dorsal side of the right and left lobes of the thyroid gland ( Fig. 4.2 ). The exact positions of the parathyroid glands are variable, and more than 10% of humans harbor a fifth parathyroid gland. The predominant parenchymal cell type in the parathyroid gland is the principal (also called chief ) cell . With age, a large mitochondria-rich, eosinophilic cell type, the oxyphil cell , appears. Although the oxyphil cell is not normally important in PTH secretion, PTH-overproducing tumors (i.e., primary hyperparathyroidism) can be derived from both principal and oxyphil cells.
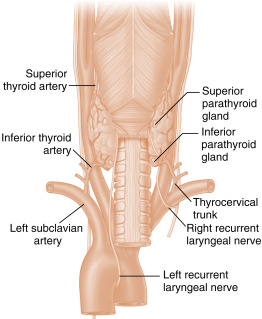
Structure, synthesis, and secretion of parathyroid hormone
Secreted PTH is an 84-amino acid polypeptide. PTH is synthesized in principal cells as prepro-PTH , which is proteolytically processed to pro-PTH in the endoplasmic reticulum, then to mature PTH in the Golgi and secretory vesicles. The primary signal that stimulates PTH secretion is a decrease in circulating Ca 2+ levels ( Fig. 4.3 ). The extracellular Ca 2+ concentration is sensed by the parathyroid principal cells through a Ca 2+ -sensing receptor (CaSR) . The CaSR is a member of the seven-transmembrane G-protein–coupled receptor superfamily that forms disulfide-linked dimers in the plasma membrane. The CaSR is also expressed in calcitonin-producing C cells, renal tubules, and several other tissues. In the parathyroid gland, increasing amounts of extracellular Ca 2+ bind to the CaSR and activate downstream signaling pathways that inhibit PTH secretion Conversely, decreasing amounts of Ca 2+ reduces CaSR signaling, resulting in increased PTH secretion. The serum Ca 2+ concentration that results in a half-maximal rate of PTH secretion has been referred to as the setpoint. Although the CaSR binds to extracellular Ca 2+ with relatively low affinity, it is extremely sensitive to changes in extracellular Ca 2+ above or below the setpoint. A 0.1 mM difference in serum Ca 2+ spans the entire range of PTH secretion rates from basal (5% of maximum) to maximal levels ( Fig. 4.4 ). Thus the CaSR regulates PTH output in response to subtle fluctuations in Ca 2+ on a minute-to-minute basis. It should be noted that the CaSR is also stimulated by high levels of magnesium, so hypermagnesemia also inhibits PTH secretion.
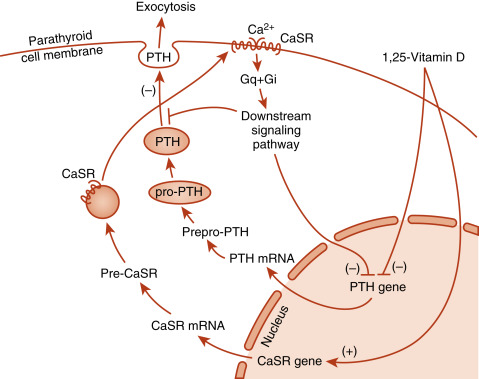
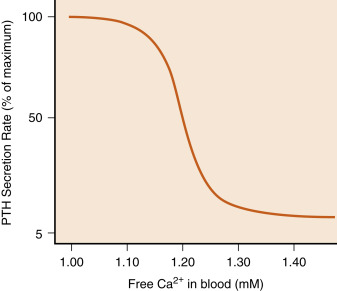
In addition to inhibiting secretion, the CaSR also regulates degradation of newly synthesized PTH. Intracellular degradation of PTH is increased during hypercalcemia, thereby reducing the availability of intact PTH, while increasing the secretion of inactive fragments. This, coupled with rapid extracellular degradation of PTH, necessitates serum PTH assays that are specific for intact hormone. Consistent with its role in minute-to-minute regulation of serum Ca 2+ , circulating intact PTH has a very short half-life (< 5 minutes). This allows intraoperative assessment of serum PTH levels during a surgical procedure to remove a PTH-secreting parathyroid adenoma.
Patients with familial hypocalciuric hypercalcemia (FHH) are heterozygous for inactivating mutations of the CaSR. In these patients, reduced expression of the CaSR requires higher levels of Ca 2+ to inhibit PTH, resulting in an elevated setpoint. The CaSR also plays a direct role in Ca 2+ reabsorption in the kidney (specifically the thick ascending limb of the loop of Henle) to protect against hypercalcemia. The hypocalciuria (i.e., the inappropriately low Ca 2+ excretion in the face of high circulating Ca 2+ levels) in patients with FHH is due to the reduced ability of the CaSR to respond to hypercalcemia by increasing urinary Ca 2+ excretion. FHH is a benign condition that must be distinguished from primary hyperparathyroidism in a hypercalcemic patient by clinical criteria, including normal to only slightly elevated serum PTH levels and low urinary calcium.
PTH production is also regulated at the level of gene transcription (see Fig. 4.3 ). Chronic activation of the CaSR signaling pathway causes repression of PTH gene expression. The PTH gene is also repressed by 1,25-dihydroxyvitamin D (acting through vitamin D–responsive elements, discussed later). The ability of 1,25-dihydroxyvitamin D to hold PTH gene expression in check is reinforced by the coordinated upregulation of CaSR gene expression by positive vitamin D–responsive elements in the promoter of the CaSR gene (see Fig. 4.3 ).
Parathyroid Hormone Receptor
The classic PTH receptor (PTH1R) is a seven-transmembrane, G-protein–coupled membrane receptor that is activated by both PTH and PTH–related peptide (PTHrP). PTH1R is primarily coupled to a Gαs signaling pathway that leads to increased cyclic adenosine monophosphate (cAMP), although it also is coupled to Gαq/11-phospholipase C–dependent pathways. PTH1R is expressed on osteoblasts in bone, and in the proximal and distal tubules of the kidney, as the receptor for the systemic actions of PTH. However, PTH1R is also expressed in many developing structures (e.g., the growth plate) in which PTHrP has an important paracrine function.
Vitamin D
Vitamin D is actually a prohormone that must undergo two successive hydroxylations to become the active hormone, 1,25-dihydroxyvitamin D ( Fig. 4.5 ). Vitamin D plays a critical role in Ca 2+ absorption, and to a lesser extent Pi absorption, by the small intestine. Vitamin D also regulates aspects of bone remodeling and facilitates renal reabsorption of Ca 2+ .
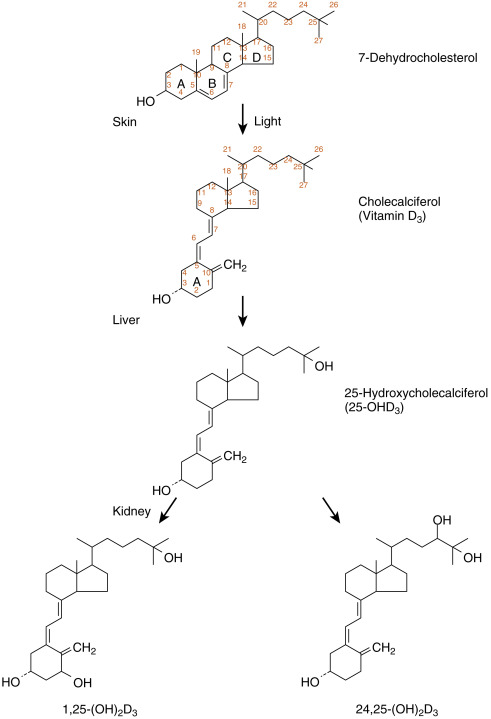
Structure, Synthesis, and Transport of Active Vitamin D Metabolites
Vitamin D 3 (D 3 ; also called cholecalciferol ) is synthesized by ultraviolet (UV) light–mediated conversion of 7-dehydrocholesterol in the more basal layers of the skin ( Fig. 4.6 ). UV radiation (specifically UVB) opens up the B ring of cholesterol, generating previtamin D 3 , which then undergoes a temperature-dependent isomerization to D 3 . Vitamin D 3 is therefore referred to as a secosteroid , a class of steroids in which one of the cholesterol rings is opened. Vitamin D 2 (D 2 , also called ergocalciferol ) is the form produced in plants. Vitamin D 3, and to a lesser extent D 2, are absorbed from the diet and are both effective after conversion into active hydroxylated forms.
The balance between UV-dependent, endogenously synthesized vitamin D 3 and the absorption of dietary forms of vitamin D becomes important in certain situations. Individuals with higher epidermal melanin content and those who live at higher latitudes convert less 7-dehydrocholesterol into vitamin D 3 and thus are more dependent on dietary sources of vitamin D, especially in winter. Some dairy products, including milk, are enriched in vitamin D 3 , but not all individuals tolerate or consume dairy products. Institutionalized, sedentary elderly patients who stay indoors and avoid dairy products are particularly at risk for developing vitamin D deficiency .
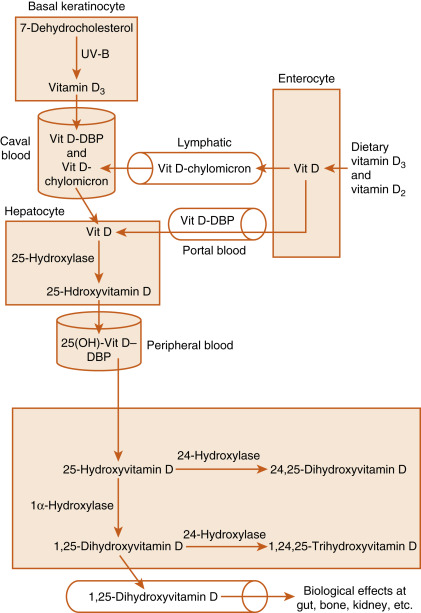
D 3 is transported in the blood from the skin to the liver. Dietary D 3 and D 2 reach the liver directly via the portal circulation and indirectly through chylomicrons (see Fig. 4.6 ). In the liver, D 2 and D 3 are hydroxylated at the 25-carbon position to yield 25-hydroxyvitamin D (no further distinction will be made between D 3 and D 2 metabolites). Hepatic 25-hydroxylase is constitutively expressed, so circulating levels of 25-hydroxyvitamin D reflect the amount of precursor available for 25-hydroxylation. For this reason, assay of serum 25-hydroxyvitamin D levels is used to assess a patient’s vitamin D status. Because the hydroxyl group at the 25-carbon position represents the second hydroxyl group on the molecule, 25-hydroxyvitamin D is also referred to as calcifediol (see Fig. 4.6 ).
25-Hydroxyvitamin D is further hydroxylated in the mitochondria of the proximal tubules of the kidney at either the 1α-carbon or 24-carbon position (see Figs. 4.5 and 4.6 ). The 1α-hydroxylase (gene symbol CYP27B1) generates 1,25-dihydroxyvitamin D (also called calcitriol ), which is the most active form of vitamin D. Hydroxylation at the 24 position, generating 24,25-dihydroxyvitamin D and 1,24,25-trihydroxyvitamin D , represents an inactivation pathway.
Vitamin D and its metabolites circulate in the blood primarily bound to vitamin D–binding protein (DBP) . DBP is a serum glycoprotein of about 60 kDa that is related to the albumin gene family and is synthesized by the liver. DBP binds more than 85% of 25-hydroxyvitamin D and 1,25-dihydroxyvitamin D. As a result of binding to other proteins, only 0.4% of the active metabolite, 1,25-dihydroxyvitamin D, circulates as free steroid. DBP allows for the movement of the highly lipophilic molecules within the aqueous environment of the blood and provides a reservoir of vitamin D metabolites that protects against vitamin D deficiency. The bound fractions of vitamin D metabolites have a circulating half-life of several hours.
There are several factors that regulate renal 1α-hydroxylase activities (see Fig. 4.6 ). Low serum Ca 2+ is associated with increased 1α-hydroxylase activity, an effect that is primarily mediated by elevated levels of PTH. Similarly, it has long been known that hypophosphatemia is associated with increased production of 1,25-dihydroxyvitamin D. Recent elucidation of the role of FGF23 on Pi metabolism has shed light on the mechanism. FGF23 acts on the FGFR1/Klotho receptor complex in the proximal tubule to inhibit Pi reabsorption and 1α-hydroxylase activity. Conversely, low serum Pi inhibits the production of FGF23 in bone, leading to enhanced Pi reabsorption and stimulation of 1α-hydroxylase activity in the kidney. Lastly, there is evidence for a short feedback loop in which 1,25-dihydroxyvitamin D inhibits 1α-hydroxylase expression. Regulation and activity of the 24-hydroxylase inactivation pathway generally exhibits reciprocity to that of the 1α-hydroxylase.
Vitamin D Receptor
1,25-Dihydroxyvitamin D exerts its actions primarily through binding to the nuclear vitamin D receptor (VDR) . The VDR is a 50-kDa protein and is a member of the nuclear hormone receptor superfamily, which also includes steroid and thyroid hormone receptors and metabolic receptors such as the peroxisome proliferator–activated receptors (see Chapter 1 ). The VDR is a transcription factor that binds to DNA sequences ( vitamin D–response elements ) as a heterodimer with the retinoid X receptor (RXR). Thus a primary action of 1,25-dihydroxyvitamin D is to regulate gene expression in its target tissues, including the small intestine, bone, kidneys, and parathyroid gland.
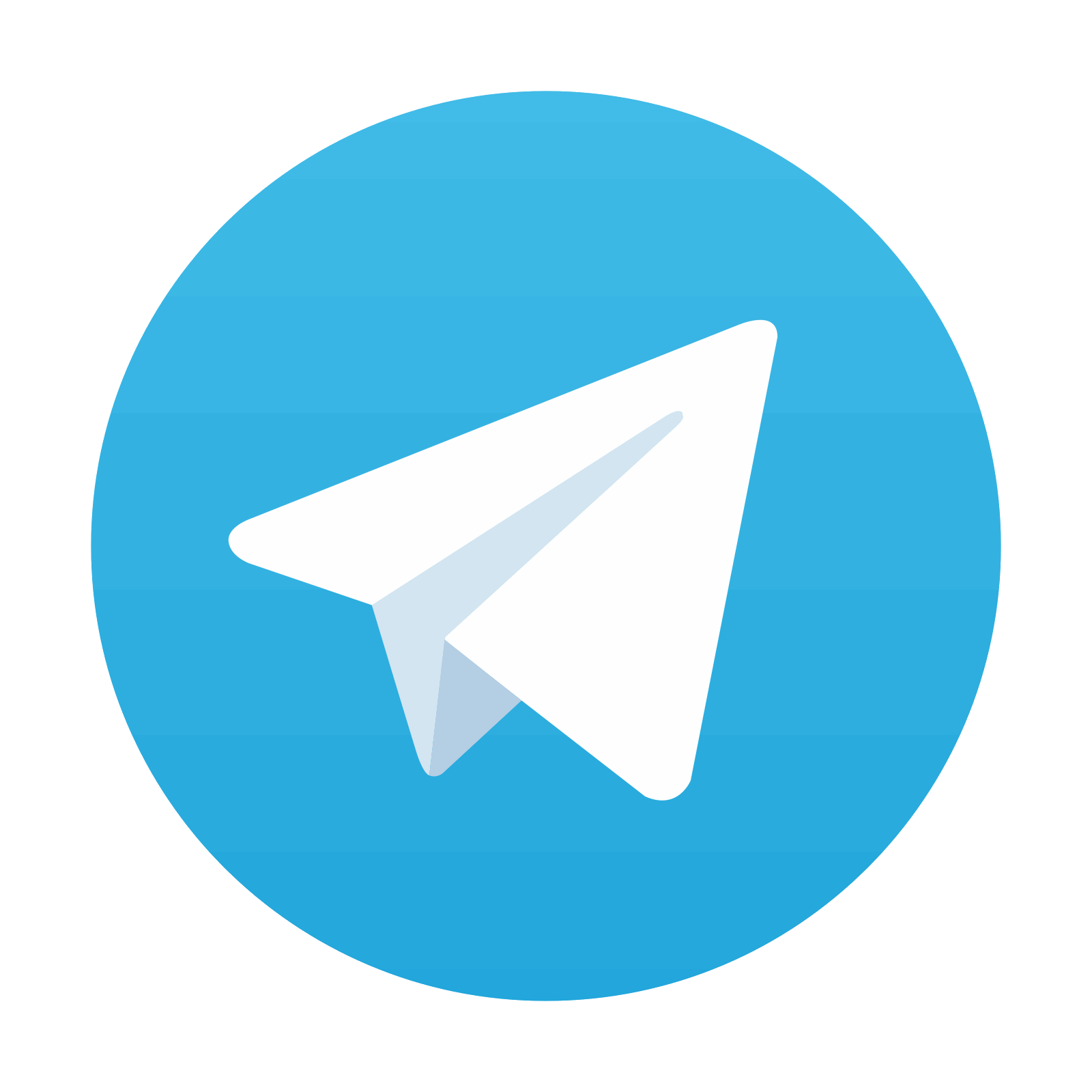
Stay updated, free articles. Join our Telegram channel

Full access? Get Clinical Tree
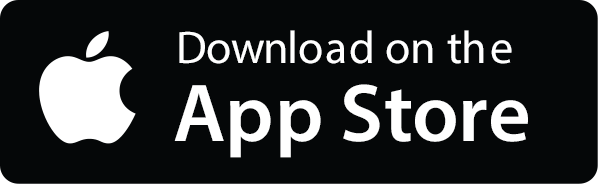
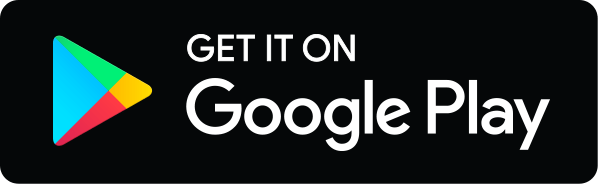