Advances in the molecular characterization of human tumors have led to increased interest in the development of targeted therapeutics to include monoclonal antibodies and cancer vaccines. Interest in the development of cancer vaccines has increased since these advances led to the identification of tumor-associated antigens (TAAs).1-3 TAAs expressed by tumors are able to elicit a specific immune response. In addition to having an antigen that serves as a target for the immune response, a successful tumor vaccine requires a platform to present the antigen to the immune system and an environment that is conducive to immune stimulation. The goal of researchers, therefore, has been to identify TAAs and deliver them to the immune system in the context of a vaccine with the appropriate secondary signals required to prompt a robust, protective immune response.
Breast cancer vaccines are appealing because they represent a nontoxic therapeutic modality with great specificity. Another potential benefit of breast cancer vaccines is that they stimulate an immunologic memory response, potentially allowing for a sustained effect without recurrent therapy. In addition, because vaccines are designed to stimulate the immune system, patients are unlikely to develop resistance to this form of targeted therapy. In this chapter, we will review the basics of the immune system’s response to vaccination, discuss vaccine formulations, including the TAAs that serve as targets in breast cancer, and present current strategies for incorporating vaccines into treatment algorithms for patients with breast cancer.
The human immune system consists of both innate and adaptive arms. The innate immune system is made up of cells such as mast cells, phagocytes, natural killer cells, basophils, and eosinophils that defend the host in a nonspecific manner. This system provides immediate defense against infection but is unable to confer long-lasting or protective immunity. The major functions of the innate immune system therefore include the recruitment of immune cells to sites of infection or inflammation through release of cytokines, activation of the complement cascade, removal of foreign substances, and activation of the adaptive immune system by antigen presentation. The adaptive immune system then is able to confer long-lasting immunity by mounting 2 types of a response: humoral and cellular. Humoral immunity (the antibody response) involves B cells interacting with a foreign antigen, leading to differentiation into plasma cells or memory cells. Plasma cells are able to secrete specific antibodies against the antigen, while memory cells are longer lasting and function to respond quickly to future exposures of the antigen. Cellular immunity (the T-cell response) involves an interaction between T cells and processed fragments of proteins (peptides) that are present on the surface of other cells in association with major histocompatibility (MHC) molecules.
There are 2 types of MHC molecules: class I and class II (Fig. 91-1). Class I MHC molecules are found on nearly all nucleated cells. They can be loaded with peptides of 8 to 10 amino acids that are generated endogenously within the cytosol of the cell. Peptides associated with class I MHC molecules are translocated to the cell surface, where they can be recognized by specific receptors on CD8+ lymphocytes, also known as cytotoxic T cells (CTLs). CTLs confer cytolytic activity by releasing perforin and cytotoxins that induce apoptosis in the target cell. Historically, the majority of cancer vaccines have been designed to stimulate a CTL response.
Figure 91-1

Basic tumor immunology. Tumor cells express tumor associated antigens (TAA) such as HER-2 on their cell surface. These TAA can be processed by tumor cells such that peptides derived from the TAA are complexed with MHC class I or MHC class II molecules and presented on the cell surface. MHC class I molecules complexed with peptides are recognized by cytotoxic T cells, which when activated, release granzyme and perforin, which induce apoptosis in the target cell. MHC class II molecules complexed with peptides are recognized by helper T cells. Helper T cells release multiple different cytokines including IL-2 and INF-γ, which stimulate CTL and IL-4, IL-5, IL-6, IL-10, and IL-13, which in turn stimulate B cells and the humoral immune response.
Class II MHC molecules are found on a few specialized cell types, including dendritic cells, B cells, and macrophages, all of which are professional antigen-presenting cells (APC). APCs are efficient at phagocytosis and provide the co-stimulatory molecules needed to activate T cells. An APC is able to engulf a protein, break it apart, and present the peptides on the APC surface during a class II MHC response. Once loaded with the peptide on its cell surface, the APC is then able to stimulate CD4+ lymphocytes, also known as T helper (Th) cells. In addition, there are some tumor cells that express MHC class II molecules including breast, melanoma, and lung cancer.4 These tumor cells are also able to load peptide associated with MHC class II molecules on their surface therefore they can also stimulate Th cells. Two predominant Th cell subtypes exist, Th1 and Th2, and each of these subtypes has a unique role. Th1 cells are responsible for activating and regulating the development and persistence of cell-mediated immunity.5 Cytokines produced by Th1 cells include interleukin (IL)-2 and interferon gamma (INF-γ). Using a transgenic mouse model, Park and colleagues demonstrated that Th1 cells are also essential to induce complement fixing antibody and that this resulted in the prevention of breast cancer development.6 Th2 cells are associated with the humoral immune response and produce several different cytokines, including IL-4, IL-5, IL-6, IL-10, and IL-13, which have roles in B-cell maturation, clonal expansion, and class switching.7 Clearly, Th cells mediate an antitumor immune response through multiple mechanisms, and there is increasing evidence that generation of a Th-cell response will be required for a vaccine strategy to be effective.
In designing breast cancer vaccines, investigators have employed different platforms for delivering antigens to the immune system. Several of these platforms, their purported benefits, and their potential drawbacks are discussed in the next sections. Additional vaccine formulations, including genetically engineered plasmid DNA vectors, recombinant viral vectors, and recombinant bacteria, which deliver defined TAAs using gene transfer, are in the early stages of development and will not be discussed in detail in this chapter.
One strategy involves delivering vaccines derived from whole tumor cells, which has the potential advantage of delivering the complete antigen pool of a tumor, theoretically activating a polyclonal immune response. These vaccines can be composed of autologous or allogeneic tumor cells. Autologous tumor cell vaccines are produced by isolating tumor cells from an individual patient and processing these tumor cells into a vaccine in vitro by combining the cells with an immunoadjuvant (described further below) or by genetically modifying the cells. The vaccine is then administered to the patient from whom the tumor cells were initially isolated. One purported benefit of this strategy is that it delivers antigens that may be unique to an individual’s tumor.8 Unfortunately, this strategy is limited by the need to obtain a sufficient quantity of tumor cells from the patient.8 Allogeneic tumor cells are an alternative that utilize tumor cells isolated from one patient or an established tumor cell line that are subsequently killed and processed, and then administered to another patient. Allogeneic tumor cells can be used as a source of antigen because tumors from various patients have overlapping antigen expression profiles8-10 and because the tumor antigen-specific immune response can be initiated by cross-priming.8,11,12 Because tumor cells are not inherently immunogenic, either autologous or allogeneic tumor cells must be delivered in the presence of a strong immune-stimulating adjuvant, such as bacillus Calmette-Guérin (BCG), granulocyte macrophage colony-stimulating factor (GM-CSF), or cytokines known to promote T-cell proliferation. Another disadvantage of a whole tumor cell strategy is that the majority of the tumor cell is made up of normal antigens, and therefore only a small amount of relevant, immunogenic antigen is actually delivered.
Peptide-based vaccines use antigenic peptides derived from TAAs to induce peptide-specific immune regulators, including antibodies, Th cells, and CTLs, that can recognize and lyse tumor cells expressing the immunogenic peptide on their surface. The element of the immune system that is stimulated depends on the peptide utilized and the class of MHC molecule with which the peptide is complexed. Because peptides are only weakly immunogenic, they too must be combined with an immunoadjuvant to stimulate a response.
Peptides can be easily manufactured on a large scale and at a low cost; therefore peptide-based vaccines are simple to construct. One drawback of peptide vaccines is that they are HLA restricted, which limits the number of patients who could benefit from this type of vaccination. For instance, E75, a HER-2-derived peptide discussed in the “HER-2” section later in the chapter, is HLA-A2/A3 restricted.13 Approximately 50% of patients are HLA-A2+, and an additional 15% are HLA-A3+,14 suggesting that approximately two-thirds of patients with breast cancer could benefit from an E75 peptide vaccine. To overcome this limitation, investigators have begun identifying peptides for other common HLA types; therefore, it is conceivable that someday every patient could receive an HLA-specific peptide vaccine. A second drawback of vaccination with a single peptide is that the vaccine activates only one arm of the immune system. Using E75 again as an example, because E75 is presented by class I MHC molecules, it stimulates only CTLs. Thus, strategies to incorporate multiple peptides, including those that stimulate CTLs and others that stimulate Th cells, into a single, multi-epitope vaccine, are currently being investigated.15 Other investigators have utilized longer class II MHC helper peptides that contain class I MHC CTL peptides sequestered completely within their sequence. Patients immunized with the longer peptides mount both a Th cell and CTL response for an enhanced overall immunologic response.7,16
Dendritic cells (DCs) are the immune system’s most effective APCs. When activated, DCs express high levels of both class I and class II MHC molecules for priming CD8+ and CD4+ T cells. In addition, DCs provide potent costimulatory molecules and cytokines required for T-cell activation, suggesting that they may be used to induce a broad, potent, tumor-specific immune response.
Constructing a DC vaccine involves extracting DCs from a patient, using stimulants to mature and expand large quantities of the DCs, exposing these DCs to antigens from the patient’s cancer cells, and then injecting the completed vaccine into the patient so the DCs can stimulate an immune response. Although it appears simple, this process is complex, which has limited the clinical development of this vaccination strategy. One issue is with acquiring DCs, which can be obtained from the peripheral blood or derived from peripheral blood precursors, such as monocytes or mobilized CD34+ precursors. The peripheral blood concentration is low and, therefore, obtaining quantities sufficient for repetitive vaccinations is difficult. In addition, peripheral DCs are frequently functionally defective in patients with breast cancer.8,17 Monocyte-derived DCs can be obtained in large quantities but require a maturation step for immune activation. CD34-derived DCs can be robustly expanded but are expensive to generate. Once obtained, DCs can be loaded with antigen in the form of peptides, proteins, mRNA, or whole tumor cells or their lysates.
Additional challenges exist for an effective DC vaccine strategy. For example, investigators have recognized the importance of secretion of the cytokine IL-12 by DCs in order to enhance the survival and functional avidity of CTLs. CTLs sensitized in the presence of IL-12 are able to recognize the peptide at 10- to 100-fold lower concentrations than CTLs sensitized in its absence.18 IL-12 also plays a role in Th development. Given the importance of IL-12 in generating an antitumor immune response, the timing of IL-12 secretion must be accounted for when determining the optimal time-point for harvesting in vitro–activated DCs for administration to patients.19
Identifying antigens that can serve as targets for an immune response is a critical, but challenging, aspect of vaccine development, as tumors arise from tissues normally present in the body; therefore, many of the antigens presented on a tumor’s cell surface are normal proteins, or self-antigens. Self-antigens are routinely sampled by the immune system, which may lead to the development of tolerance, limiting the ability of these antigens to stimulate an immune response. There is evidence, however, that the immune system can recognize tumor cells based on the absolute level and/or timing of the expression of certain “normal” proteins.20,21 Additional factors that determine the suitability of an antigen to serve as a target for immunotherapy include its tissue expression profile; the diversity, scope, and avidity of the antigen-specific T-cell repertoire; and the commonality of the antigen between patients.8,22 A number of antigens have been tested as potential targets for breast cancer vaccines (Table 91-1).23-29 Two proteins that have been investigated extensively for use in breast cancer vaccines are mucin-1 (MUC-1) and HER-2.
Antigen | Description | Relevance in Breast Cancer | Reference |
---|---|---|---|
Carcinoembryonic antigen | Glycoprotein involved with cell adhesion | Expressed in breast cancer as well as carcinoma of the colon, rectum, lung, and pancreas | 25 |
HER-2 | Member of the epidermal growth factor family of receptor tyrosine kinases | Overexpressed in up to 30% of breast cancers; associated with a poor prognosis | 27,28 |
hTERT | Catalytic protein component of telomerase that protects telomeric ends of chromosomes from decgradation during cell division | Expressed in nearly all human cancer cells including breast cancer cells | 23 |
Mammaglobin A | Glycoprotein of unknown function | Overexpressed in 80% of primary and metastatic breast cancers | 29 |
MUC-1 | Membrane-associated glycoprotein expressed in ductal tissues | Upregulated and aberrantly glycosylated in breast cancer | 24 |
p53 | Normal p53 contributes to DNA repair and apoptosis | Mutated in approximately 20% of breast cancers | 26 |
The MUC-1 gene is a member of the mucin family, which encodes a membrane-bound phosphoprotein expressed on the apical surface by many types of ductal epithelia. It is characterized by heavy glycosylation and a 20 amino acid tandem repeat segment. MUC-1 has multiple functions, including protecting mucous membranes by binding pathogens, signal transduction, and modulating the immune system.30 In breast cancer, MUC-1 is up-regulated and aberrantly glycosylated. Changes in glycosylation result in shortening of the complex carbohydrate side chains, which in turn results in exposure of peptide and carbohydrate epitopes that can serve as targets for immunotherapy.31 Several clinical trials of MUC-1–based vaccines, using both peptide and DC platforms, have been reported. One of the earliest clinical trials involved a peptide vaccine consisting of a synthetic MUC-1 peptide mixed with BCG administered to 63 patients with adenocarcinomas of the breast, pancreas, or colon.32 Overall, the vaccine was well tolerated. Skin biopsies at the injection sites showed delayed-type hypersensitivity (DTH) reactions to MUC-1 peptides, as well as intense T-cell infiltration. Approximately one-third of the patients demonstrated a 2- to 4-fold increase in MUC-1–specific CTLs.32 Additional early clinical trials investigating MUC-1 vaccines reported inconsistent results on whether MUC-1 vaccination induced a humoral response or a peptide-specific CTL response.
Subsequently, investigators have explored alternative strategies for targeting the MUC-1 antigen. Sialyl-Tn (STn) is a carbohydrate associated with MUC-1 on a number of human cancer cells that is associated with more aggressive disease. The cancer vaccine Theratope (STn-KLH, where KLH [keyhole limpet hemocyanin] is an immunogenic carrier protein to which antigens can be coupled) was designed by incorporating a synthetic STn antigen that emulates the STn seen in human tumors. Theratope was investigated in the largest breast cancer vaccine trial to date.33 This phase III trial enrolled 1028 women with metastatic breast cancer who had stable disease or who achieved a response after initial chemotherapy. Patients were randomized to receive either Theratope (STn-KLH) or control (KLH alone). While Theratope was demonstrated to be safe and capable of generating an immune response, no differences were found in time to disease progression or overall survival between the patients who received Theratope and those who received the control.33 Additional analyses of the data from this trial did show a trend toward improved time to disease progression and overall survival in patients who also received concomitant hormone therapy.34 In addition, the median overall survival was longer in patients who had an adequate antibody response to STn.35
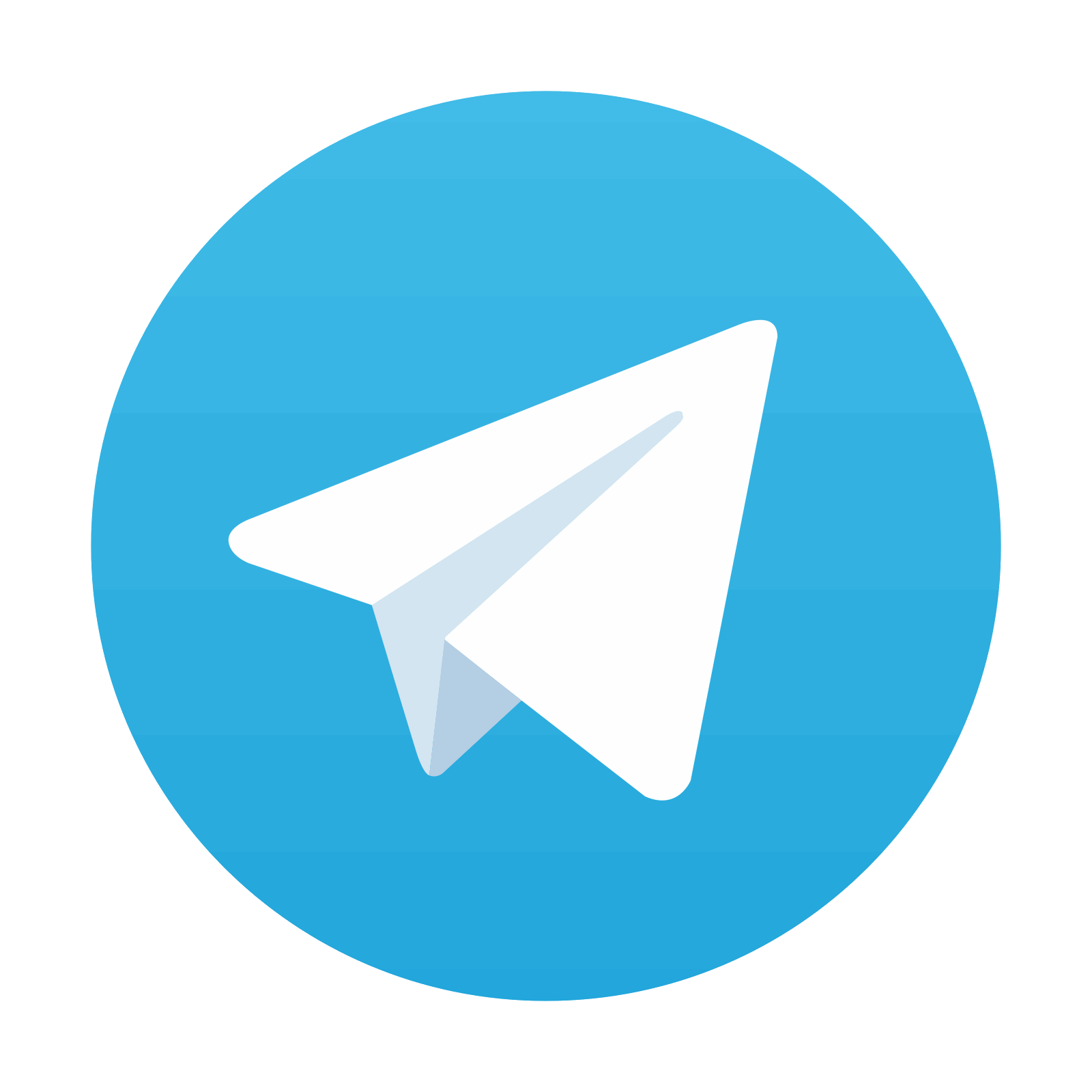
Stay updated, free articles. Join our Telegram channel

Full access? Get Clinical Tree
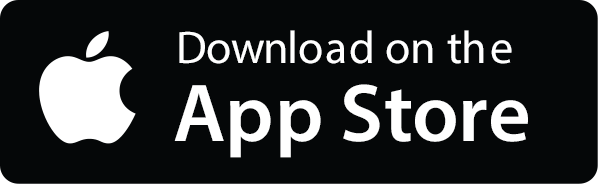
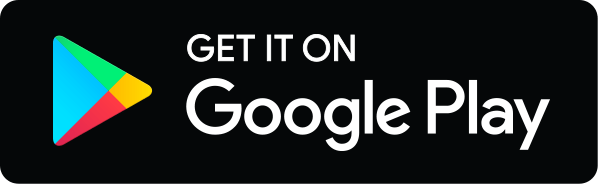