Breast Cancer: Early Stage
Radiation therapy plays an essential and critical role in the management of breast cancer. In a general radiation oncology practice, breast cancer typically comprises approximately 25% of total patient caseload. This chapter will provide an overview of general concepts in breast cancer and will then focus on management of early-stage invasive disease. The conservative management of early-stage disease by lumpectomy with or without radiation is a major focus of this chapter. Postmastectomy radiation, as well as advanced invasive disease and local-regional recurrence, will be covered in the advanced disease chapter that follows. Management of ductal carcinoma in situ and lobular carcinoma in situ are the focus of the previous chapter.
ANATOMY
The female breast lies on the anterior chest wall superficial to the pectoralis major muscle.1 The breast can extend from the midline to near the midaxillary line and cranial caudally from the second anterior rib to the sixth anterior rib. The upper-outer quadrant of the breast extends into the region of the low axilla and is frequently referred to as the axillary tail of Spence. This anatomical feature results in the upper outer quadrant of the breast containing a greater percentage of total breast tissue compared with the other quadrants, and, therefore, a greater percentage of breast cancers occur in this anatomical location.
The breast is made up of the mammary gland, fat, blood vessels, nerves, and lymphatics2 (Fig. 56.1). The surface of the breast has deep attachments of fibrous septa, called Cooper’s ligament, which run between the superficial fascia (attached to the skin) and the deep fascia (covering the pectoralis major and other muscles of the chest wall). Skin dimpling may be caused by tumors affecting these supporting structures. It is important to realize, from a staging perspective, that the chest wall includes the ribs, intercostal muscles, and the serratus anterior muscle, but not the pectoral muscles.
The breast parenchyma is composed of lobules and ducts. The function of the lobules is to produce milk and the function of the ducts is to transport lactation products to the nipple. The peripheral ducts converge into major lactiferous ducts, which then communicate with the nipple–areola complex. Most breast cancers develop at the interface between the ductal system and the lobules, a region called the terminal ductal lobular unit.
The breast parenchyma is intermixed with connective tissue, which has a rich vascular and lymphatic network. Mammary gland lymphatics begin in the interlobular or prelobular spaces, follow the ducts, and end in the subareolar network of lymphatics of the skin. The predominant lymphatic drainage of the breast is to axillary lymph nodes, which is commonly described in three levels, based on the relation of the lymph node regions to the pectoralis minor muscle (Fig. 56.2). The level I axilla is caudal and lateral to the muscle, level II is beneath the muscle, and level III (also known as the infraclavicular region) is cranial and medial to the muscle. A standard axillary lymph node dissection resects the tissue and lymph nodes within levels I and II. It is very unusual to have involvement of level III of the axilla without disease in level I or II. The axillary lymph nodes continue underneath the clavicle to become the supraclavicular lymph nodes, which can be involved in locally advanced breast cancers.
Lymphatics can also drain directly into the internal mammary lymph node chain (IMC), which are intrathoracic structures located in the parasternal space. Although these nodes are not usually visualized on computed tomography (CT), the anatomical region of the IMC can be determined by the internal mammary artery and vein, which are easily visualized by CT (Fig. 56.3) and usually lie 3 to 4 cm lateral to midline. When breast cancer involves the IMC, the majority of patients will have disease that is limited to lymph nodes in the first three interspaces. Regardless of location in the breast, the axilla is the most common site of lymphatic involvement. However, breast cancers that develop in the medial, central, or lower breast more commonly drain to the IMC (in addition to the axilla) than those occurring in the lateral and upper quadrants.
The use of lymphoscintigraphy, by injecting technicium-99 radiocolloid into the peritumoral region followed by scintillation scanning, is used now for sentinel lymph node imaging. This technique has helped to delineate primary lymphatic drainage patterns of breast cancer. The distribution of axillary and internal mammary drainage is summarized in Figure 56.4.3 Even in inner quadrant lesions, axillary drainage is more common than internal mammary drainage. However, internal mammary drainage was present in over 50% of lower inner quadrant lesions.
FIGURE 56.1. Anatomy of the breast and lymphatic drainage. (From Osborne MP. Breast development and anatomy. In: Harris JR, Hellman S, Henderson IC, et al., eds. Breast diseases. Philadelphia: JB Lippincott, 1987:1–14, with permission.)
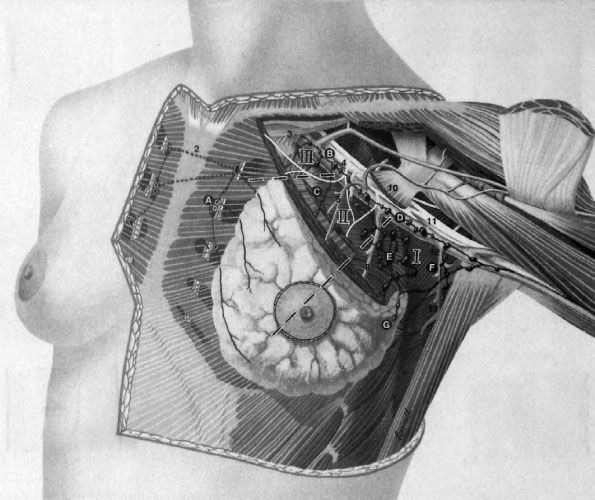
FIGURE 56.2. Location of the three levels of axillary lymph nodes. (Redrawn from Morrow M. Axillary node dissection: what role in managing BCa? Contemp Oncol 1994;8(4):16–27, copyright Medical Economics. Adapted from an illustration by John Daughterty, copyright 1994.)
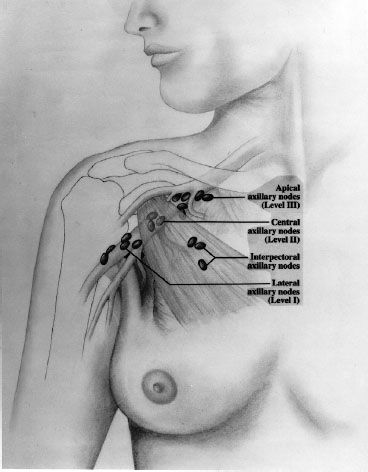
FIGURE 56.3. Treatment planning computed tomography scan of the chest demonstrating the location of the internal mammary vessels, which are typically located approximately 3 to 4 cm lateral to midline and approximately 3 cm deep to the surface. The internal mammary nodes are in close proximity to the vessels, with the most critical nodes being located in the first three intercostal spaces.
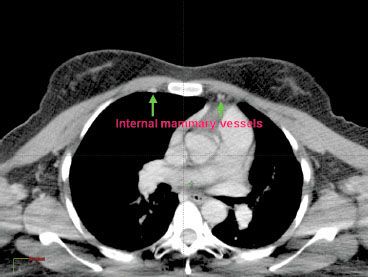
FIGURE 56.4. Distribution of lymphatic drainage of the breast to axillary and internal mammary chains according to the location within the breast. (Data extracted from study of 700 patients undergoing sentinel lymph node mapping by Estourgie et al. Lymphatic drainage patterns from the breast. Ann Surg 2004;239(2):232–237.)
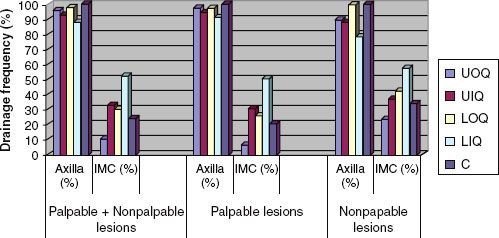
FIGURE 56.5. Age-adjusted cancer death rates for women. Statistics show a recent decrease in breast cancer mortality due to an increase in screening detected malignancies and improvements in treatment. (From Jemal A, Siegel R, Xu J, et al. Cancer statistics, 2010. CA Cancer J Clin 2010;60:277–300, with permission.)
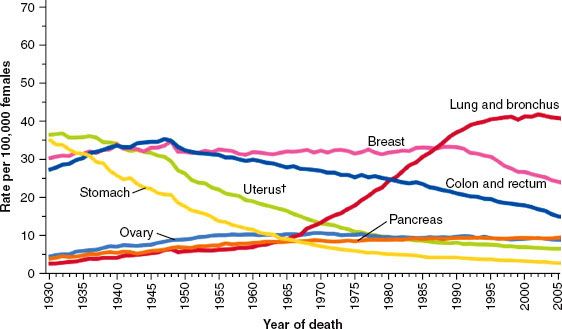
EPIDEMIOLOGY
Breast cancer is the most frequently diagnosed cancer in women, and it is estimated that there will be 229,060 new cases of invasive breast cancer and 63,300 new cases of in situ breast cancers among women in the United States in 2012.4 Primarily due to increased utilization of screening mammography, breast cancer incidence rates increased rapidly in the 1980s. It is estimated that 39,920 breast cancer deaths will occur in 2012, with breast cancer ranking second among cancer deaths in women (after lung cancer). In contrast to the significant number of breast cancer cases in women, it is expected that 2,190 cases of breast cancer will be diagnosed in men in 2012, with approximately 410 breast cancer deaths in men. Due to a combination of early detection, increased awareness, and improvements in therapy, death rates from breast cancer actually declined by approximately 2.3% per year from 1990 to 2007. The decrease in breast cancer mortality is demonstrated in Figure 56.5.
There is considerable geographic, ethnic, and racial variability in breast cancer incidence. Ethnicity and national origin rank highly as predictors of risk for breast cancer, with up to a 10-fold variation throughout the world.5 Compared with other well-established risk factors such as age of menarche and menopause, age at first childbirth, and family history, geographic and ethnic variability is quite significant. It is likely that a complex interaction of multiple factors, including genetic, environmental, and socioeconomic, contribute to the wide variability in age-adjusted incidence across populations.
The potential contribution of environmental factors and lifestyle is clearly demonstrated in the increasing incidence of breast cancers among Japanese American women and in trends of increasing incidence of breast cancer in Japan with recent changes in lifestyle. It is well recognized that the relatively low incidence of breast cancer in Asian immigrants to the United States has gradually increased as these immigrants have adapted to Western lifestyles.6 In Japan, incidence rates have more than doubled from 1960 to 1990. This is likely a result of adaptation of Western lifestyles, including fewer children, later marriage, increasing rates of obesity, and possibly dietary influences.7
In the United States, the incidence of breast cancer in white women is higher than all other populations. Recent data from the National Cancer Institute’s Surveillance, Epidemiology, and End Results (SEER) program report incidence rates of 141 cases per 100,000 white women, compared with 122 in African American, 97 in Asian or Pacific Islanders, 90 in Hispanics, and 58 in Native Americans or Alaskan Natives.8
Although incidence is lower in African American women, the age of onset is younger and African American women are more likely to be diagnosed at a more advanced stage. Several studies have reported an earlier onset of breast cancer in African American, compared with white women, by approximately 10 years; other studies have indicated that after correcting for stage, African American women have more aggressive biology and a poorer overall prognosis.9,10
TABLE 56.1 RISK FACTORS FOR BREAST CANCER IN WOMEN
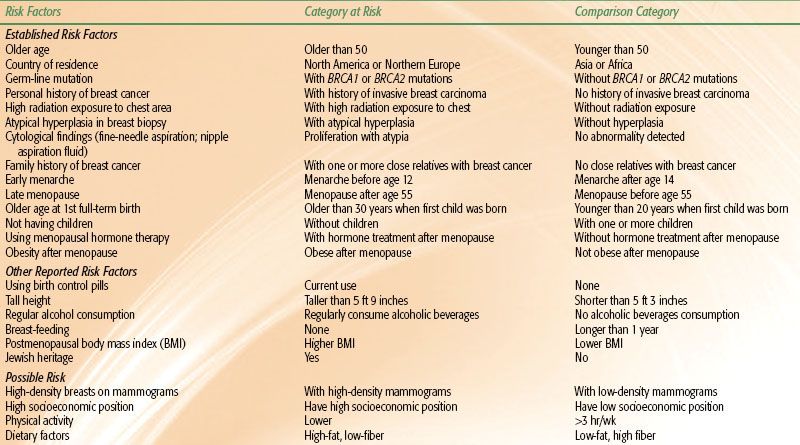
Risk Factors
Table 56.1 summarizes the major risk factors associated with development of breast cancer. With the exception of female gender, increasing age is the most consistent and significant risk factor, with most populations demonstrating increasing incidence rates with age. Other risk factors include personal history and family history of breast cancer, nulliparity or late age at first childbirth, early menarche and late menopause, prior breast biopsy with hyperplasia or atypical hyperplasia, high breast tissue density, radiation exposure at a young age, alcohol consumption, and use of postmenopausal hormone therapy. Some of the national origin or ethnicity variability discussed above may be explained in part by differences in established risk factors, such as age of menarche, parity, and age at first childbirth. However, these factors explain only part of the variability observed in national origin, indicating underlying genetic, environmental, and dietary factors are likely to contribute to the differences in the worldwide incidence of breast cancer.11 Breastfeeding, physical activity, and maintaining a healthy body weight have been demonstrated in various studies to be associated with a lower risk of breast cancer.11
Age
The risk of breast cancer increases exponentially up to the age of menopause, at which time the rate of increase in the risk slows significantly. After the age of 80, the incidence of breast cancer begins to show a slight decline. For women in their late 30s, the annual increase in risk of developing breast cancer is approximately 0.07% per year. This increases to 0.44% per year for women in their late 70s. Although these percentages may seem low, they represent only the risk for a given year; the lifetime risk is a summation of the annual breast cancer risks. Because younger women have a longer life expectancy than older women, younger women have a greater lifetime risk. Only 0.43% of women develop breast cancer before the age of 40, whereas 4% of women develop breast cancer between the ages of 40 and 59 and 6.88% of women develop breast cancer between the ages of 60 and 79.8
Child Bearing/Parity/Breastfeeding
The protective effect of child bearing at younger ages on breast cancer risk is well established. In a worldwide case control study, MacMahon et al.5,12 demonstrated a nearly linear relation between relative risk of breast cancer and age at first birth, with women aged 20 to 25 having nearly a 50% reduction in the relative risk of breast cancer compared with nulliparous women. Interestingly, for women whose first childbirth occurred over age 35, the risk appears greater than nulliparous women. Data on the effect of breastfeeding are not as strong as the data on age at first childbirth, but they do suggest a protective effect. The Oxford Collaborative Group conducted an analysis of 47 studies evaluating breastfeeding and breast cancer risk and reported a decrease in relative risk of breast cancer by 4.3% for each 12 months of breastfeeding.13
Ovarian Function
The relation between ovarian function and breast cancer risk has long been recognized, with long menstrual history (early menarche and late menopause) contributing significantly to breast cancer risk. In experimental models and observational studies, removal of the ovaries reduces the risk of breast cancer.5 Women with surgically induced menopause have been shown to have significantly reduced risks of breast cancer compared with women whose menopause occurred naturally. In comparison with women whose menopause occurs between the ages of 45 and 54 (relative risk = 1), women with early menopause before age 45 have a relative risk of breast cancer of 0.73 and women with late menopause at age 55 or older have a relative risk of 1.48. The data on early onset of menses and its association with breast cancer risk are also well established.5
Exogenous Hormone
The risk of breast cancer associated with hormonal therapy has been controversial. A collaborative meta-analysis from 51 epidemiological studies of over 150,000 women did show an increased relative risk of 1.35 for current or recent users of hormonal replacement therapy.14 The authors reported that postmenopausal hormone replacement therapy increased the annual relative risk of developing breast cancer by 2.3% for each year of hormonal therapy. A randomized trial of postmenopausal hormone therapy from the Women’s Health Initiative Study comparing estrogen and progestin with placebo was closed prematurely, demonstrating a 24% increase in breast cancer, coronary heart disease, stroke, and pulmonary emboli. This study of 46,000 women reported that the combined use of estrogen and progesterone increased the relative risk of breast cancer 8% compared with the risk in nonusers, whereas the use of estrogen alone increased the relative risk only 1%.15 After publication of this study, a dramatic decrease of almost 7% between 2002 to 2003 was primarily attributed to the reduction in the use of hormone replacement therapy following the publication of results from the Women’s Health Initiative in 2002. Since then, breast cancer incidence rates have been generally stable.4 Other studies have also demonstrated increased risks of breast cancer with long-term use of hormonal replacement therapy.16 However, short-term use of hormonal replacement, particularly in women with severe menopausal symptoms, has not been consistently associated with breast cancer risk. For women who have undergone hysterectomy, it seems that hormone replacement therapy with estrogen alone rather than estrogen and progesterone has a minimal effect on breast cancer risk. For women who have not undergone hysterectomy and who elect to be treated with hormone replacement therapy, combined estrogen and progesterone remains the standard for hormone replacement therapy to avoid the risk of endometrial cancer that is associated with unopposed estrogen replacement.16
The use of oral contraceptives has not been consistently shown to increase the risk of breast cancer. There is some evidence that use of oral contraceptives for more than 4 years prior to first pregnancy increases the risk of breast cancer. Other studies, however, have not demonstrated increased risks of breast cancer, even with long-term exposures of more than 15 years.17,18
Family History
The increased risk of breast cancer as a function of family history is well established. For women with a second-degree relative (aunt, grandmother) with breast cancer, the risk is about 1.5, and for women with a history in first-degree relatives (mother or sister), the risk is 1.7 to 2.5.19 This may be explained in part by inheritance of a genetic condition that predisposes an individual to breast cancer development (e.g., mutations in BRCA1 or BRCA2); shared lifestyle; and inheritance of genes that affect risk factors, such as body habitus and age at menarche. Between 20% and 25% of women diagnosed with breast cancer have a positive family history of the disease, and approximately 10% of women with breast cancer are from families who display an autosomal dominant pattern of breast cancer inheritance.20 The actual risk that family history conveys depends on the number of relatives affected and their age at diagnosis (having a first-degree relative with premenopausal breast cancer conveys a greater risk than does having a first-degree relative with postmenopausal cancer). Women with one first-degree relative affected by the disease have an increased relative risk of developing breast cancer two to three times that of women with no family history. Women with two or more first-degree relatives with a diagnosis of breast cancer have a still greater risk, four to six times that of women with no family history.19
Women with a strong family history, particularly those with multiple first- and second-degree relatives diagnosed with breast cancer in the premenopausal years, are at risk for carrying mutations in the breast cancer susceptibility genes, BRCA1 or BRCA2. Although these mutations are present in <1% of the population and account for approximately 5% to 10% of all breast cancer cases, women carrying these mutations have a lifetime risk of developing breast cancer of up to 70% to 80%.20 Genetic counseling or testing should be considered in women at risk for carrying these mutations. Recently, the National Comprehensive Cancer Network (NCCN) published guidelines for genetic testing.21,22 In the context of pre- and posttest counseling, the NCCN recommends that genetic testing be offered when:
1. The individual has a family history of a known BRCA1/BRCA2 mutation,
2. Personal history of breast cancer plus one of the following:
a. Diagnosed age 45 years or younger
b. Diagnosed age ≤50 years with one or more close blood relatives with breast cancer ≤50 years
c. Two breast primaries when first breast primary occurred before age 50
d. Diagnosed at any age, with two or more close blood relatives with breast and/or epithelial ovarian/fallopian tube/primary peritoneal cancer at any age
e. Close male relative with breast cancer
f. An individual of ethnicity associated with higher mutation frequency (e.g., Ashkenazi Jewish).
3. Personal history of epithelial ovarian/fallopian tube/primary peritoneal cancer, or
4. Personal history of male breast cancer.
Personal History of Breast Cancer and History of “Benign” Breast Biopsy
Women with a prior history of breast cancer are at an elevated risk to develop a second contralateral breast cancer.1 Studies with long-term follow-up have demonstrated a risk of breast cancer in the contralateral breast of approximately 10% to 15%, depending on the patient population and length of follow-up.23 Patients treated for invasive breast cancer or ductal carcinoma in situ (DCIS) have similar risks of developing a contralateral breast cancer, which does not appear to be effected by the type of local therapy for the initial lesion. A recent analysis of DCIS patients from the Connecticut Tumor Registry demonstrated a relative risk of developing contralateral breast cancer of 3.35 compared with women without a diagnosis of breast cancer.24 The risk of contralateral breast cancer as a function of prior radiation treatment is discussed in detail later.
Although women with a history of fibrocystic changes have been reported to have an elevated risk of breast cancer, recent evidence suggests that the majority of the elevated risk is due to the smaller proportion of women whose biopsy reveals atypical hyperplasia. Results from the Breast Cancer Detection Demonstration Project, which included over 280,000 women in 29 centers, demonstrated that women with atypical hyperplasia had 4.3 times the breast cancer risk of women without proliferative disease (95% confidence interval [CI], 1.7 to 11.0). In women with proliferative disease lacking atypical hyperplasia the relative risk was 1.3 (95% CI, 0.77 to 2.2). In that study the joint occurrence of family history and atypical hyperplasia had a strong synergistic effect on breast cancer risk.25
Radiation Exposure
Exposure to ionizing radiation during or after puberty increases the risk for development of carcinoma of the breast. Land et al.26,27 reviewed reports on three populations of patients exposed to ionizing radiation by atomic bombings, multiple fluoroscopic examinations for tuberculosis, and multiple examinations for mastitis. They concluded that the risk of radiation-induced cancer of the breast increased approximately linearly with increasing dose and was heavily dependent on age at exposure.
In a study of 31,710 women who had tuberculosis and were examined with repeated fluoroscopic studies, a substantial proportion (26.4%) received doses to the breast of ≥0.1 Gy; the breast cancer risk was greatest among women who had radiation exposure between the ages of 10 and 14 years (relative risk [RR] 4.5 per 0.01 Gy and an additive risk of 6.1 per 104 person-years per 0.01 Gy); there was substantially less excess risk with increasing age at first exposure.28
A high risk of solid tumors, especially breast cancer, has been described in women treated with radiation therapy at a young age for Hodgkin lymphoma. In a review of 1,380 women treated at 15 institutions before the age of 16 years, breast cancer developed in 17 women; 7 after radiation therapy alone and in 10 after irradiation and chemotherapy. Sixteen breast cancers appeared within or at the margin of the irradiation fields. The cumulative probability of breast cancer at 40 years of age was 35%. Women in this cohort of survivors had a risk of breast cancer 70 times higher than that of the general population.29
In a recent study, relative risks of breast cancer were defined by radiation dose to the chest (0, 20 ≤ 40 Gy, or ≥ 40 Gy). Estimates were from this case-control study conducted within an international population-based cohort of 3,817 female survivors of Hodgkin lymphoma diagnosed at age 30 years or younger. For a survivor who was treated at age 25 years with a chest radiation dose of at least 40 Gy without alkylating agents, estimated cumulative absolute risks of breast cancer by age 35, 45, and 55 years were 1.4% (95% CI, 0.9% to 2.1%), 11.1% (95% CI, 7.4% to 16.3%), and 29.0% (95% CI, 20.2% to 40.1%), respectively.30 A reduced volume of radiation fields has also been shown to reduce the breast cancer risk associated with treatment of Hodgkin lymphoma. The current practice of limiting radiation fields to involved nodal regions should help to further reduce the risk of radiation related breast cancers in Hodgkin’s survivors.31
Body Mass Index, Physical Activity, and Dietary Factors
The inherent complex interaction between body mass, physical activity, and diet complicates interpretation of epidemiologic studies correlating these factors with breast cancer risk. Body mass index (BMI) has been clearly associated with breast cancer risk in a number of studies, but it appears to influence breast cancer risk predominantly in postmenopausal women. In premenopausal women, most studies have not observed a strong relation between BMI and breast cancer risk. In postmenopausal women, a pooled analysis of prospective studies demonstrated the risk of breast cancer to be 30% higher in postmenopausal women with a BMI over 31 kg/m2 compared with women with a BMI of 20 kg/m2.32 The higher risk of breast cancer with increased BMI in postmenopausal women is likely due to higher estradiol levels associated with increased adipose tissue and increased aromatase, which is involved in the conversion of androgens to estradiol. In postmenopausal women, this is the primary source of estradiol, whereas in premenopausal women estradiol is predominantly from the ovaries so there is little association with BMI and estradiol levels.
Physical activity can have a significant impact on BMI, so it is sometimes difficult to separate these two effects in interpreting breast cancer risk. A majority of studies, however, have observed a lower risk of breast cancer among women who are more physically active compared with women who are sedentary.
Although it has been suggested that obesity and high intake of meat, dairy products, and fat may increases risk and fiber, fruits and vegetables, and phytoestrogens (soy products) may reduce risk, strong links between diet and breast cancer risk have not been clearly established.33 Accurate data regarding nutritional factors are difficult to evaluate in most epidemiologic studies. A pooled analysis of eight prospective studies did not conclude a relation between dietary fat intake and breast cancer risk. Similarly, large prospective studies have failed to demonstrate an association between dietary fiber intake and breast cancer risk.34 Phytoestrogens found in soy products and many cereals, tea, and vegetables may reduce the effects of estrogens. Given the lower incidence of breast cancer in Asian countries with high soy intake, one might hypothesize a relation between this dietary factor and breast cancer risk. Although animal studies suggest that high soy intake is protective, human studies have not been as conclusive.
Alcohol Consumption
In an analysis by the Oxford Group of 53 epidemiological studies, including 58,515 women with breast cancer and 95,067 women without breast cancer, women with daily consumption of four or more drinks a day had a 50% higher breast cancer risk.35 The average consumption of alcohol reported was 6.0 g per day (about half a unit or drink of alcohol per day). Compared with women who reported drinking no alcohol, the relative risk of breast cancer was 1.32 (1.19 to 1.45; P <.00001) for an intake of 35 to 44 g per day alcohol, and 1.46 (1.33 to 1.61; P <.00001) for ≥45 g per day or more of alcohol. The relative risk of breast cancer increased by 7.1% (95% CI, 5.5 to 8.7%; P <.00001) for each additional drink of alcohol consumed on a daily basis. Chen et al.36 reported on a prospective observational study of 105,986 women enrolled in the Nurses’ Health Study between 1980 and 2008. With 2.4 million person-years of follow-up, 7,690 cases of invasive breast cancer were diagnosed. They found that increasing alcohol consumption was associated with increased breast cancer risk that was statistically significant at levels as low as three to six drinks per week (RR 1.15; 95% CI, 1.06 to 1.24). Moreover, binge drinking, but not frequency of drinking, was associated with breast cancer risk after controlling for cumulative alcohol intake. They concluded that low levels of alcohol consumption were associated with a small increase in breast cancer risk, with the most consistent measure being cumulative alcohol intake throughout adult life.
Although the relation among physical activity, BMI, and dietary factors may be difficult to separate, it is apparent that maintaining a sound, varied diet, limiting alcohol intake, avoidance of obesity, and moderate physical activity are modifiable behaviors that can impact breast cancer risk (as well as other health-related issues) and should be encouraged.
Mammographic Density
There is a large body of evidence suggesting a correlation between mammographic breast density and breast cancer risk.37,38–39 The risk of breast cancer associated with the highest category of density has been estimated to be two to six times greater than in the lowest category. Although the causal link between mammographic density remains poorly understood, breast density is in part attributable to genetic factors.
Boyd et al.40 and Byrne et al.41 noted that women with 75% or greater breast density parenchymal patterns on the mammogram had a fivefold greater risk of breast cancer. This parameter was independent of other prognostic factors, such as family history, age at first birth, or alcohol consumption.
Determining an Individual’s Risk
It is important to consider the combination of risk factors when a generalized risk profile is determined. Gail et al.42 have used these epidemiologic risk factors to derive a model for predicting an individual’s annual and lifetime risks of breast cancer. In the Gail model, an individual’s annual risk of breast cancer is based on her present age, number of first-degree relatives with breast cancer, age at first birth, age at menarche, number of breast biopsies, and history of atypical ductal hyperplasia. The use of exogenous hormones is not considered in this model, and many of the other risk factors discussed above are not incorporated into this specific model.
TABLE 56.2 GENES ASSOCIATED WITH HEREDITARY BREAST CANCER
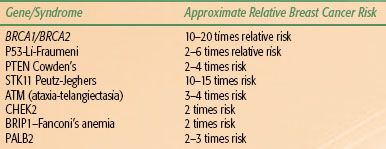
TABLE 56.3 PROBABILITY OF BRCA1 GERMLINE MUTATIONS IN VARIOUS CLINICAL SCENARIOS
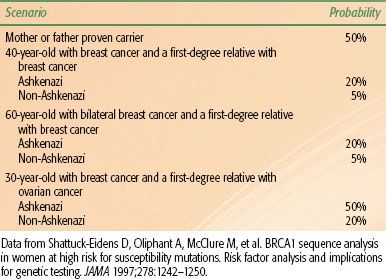
PREVENTION AND GENETIC SCREENING
Approximately 10% of breast cancer patients have familial breast cancer, typically defined as breast cancer showing an autosomal dominant inheritance pattern.20 During the 1990s, germline mutations in three important tumor suppressor genes—p53, BRCA1, and BRCA2—were discovered in family members of individuals with familial breast cancer.43–45 All three genes have been shown unequivocally to predispose to breast cancer. A number of genetic conditions associated with increased risk of breast cancer are summarized in Table 56.2.
Mutations in p53
Germline mutations in the p53 gene are very rare and result in Li-Fraumeni syndrome, named after two investigators who made significant contributions to the understanding of this condition.45 The p53 gene is one of the most important tumor suppressor genes and has been called the “guardian of the genome” because of its critical role in cellular pathways that recognize and direct a response to DNA injury. One consequence of a germline mutation in p53 is an increased risk for a variety of cancers, including childhood sarcomas, gynecologic tumors, and breast cancer. Breast cancer is the most common malignancy in patients with Li-Fraumeni syndrome; the lifetime risk is estimated to be 90%.45
Mutations in BRCA1 and BRCA2
Studies of patients with familial breast cancer led to the discovery of BRCA1 in 1995 and BRCA2 in 1996. Similar to p53, both BRCA1 and BRCA2 are tumor suppressor genes that contribute to the stability of the genome by mediating the effects of the cellular response to DNA injury. Individuals with a germline mutation in BRCA1 have a lifetime risk of breast cancer of 65% to 85%. In addition, these individuals have an elevated lifetime risk of ovarian cancer, which may approach 50%. Other types of cancer that develop more frequently in BRCA1 carriers include colon and prostate cancers. The lifetime risk of breast cancer for women with germline BRCA2 mutations mirrors that for women with BRCA1 mutations. BRCA2 mutation carriers are also at increased risk for ovarian cancer compared with the general population, but their risk is much less than the risk in women with BRCA1 mutations. BRCA2 is also associated with male breast cancer and pancreatic cancer. Genetic screening for germline mutations in BRCA1 and BRCA2 is now possible. Testing should be performed in centers equipped with genetic counseling programs designed to properly inform individuals of the social, economic, and legal consequences associated with genetic testing. Germline mutations in BRCA1 and BRCA2 are rare, occurring in fewer than 7% of patients with breast cancer. Thus, only a minority of breast cancer patients with a family history of the disease would be predicted to carry a mutation in one of these genes. Table 56.3 contains data concerning the probability of carrying a BRCA1 mutation based on an individual’s age at cancer diagnosis, personal cancer history, and family cancer history and whether the individual is of Ashkenazi Jewish descent.46
No definitive data exist on which to base screening recommendations for individuals with a proven germline mutation in a gene predisposing to the development of breast cancer. The NCCN has published a guideline recommending that individuals with a genetic predisposition undergo annual clinical and self-breast examination prior to age 25 and annual mammography or magnetic resonance imaging (MRI) and semiannual clinical and self-breast examination after age 25.47 In addition, annual pelvic examinations with transvaginal sonography, color Doppler examinations of the ovaries, and measurement of serum cancer antigen (CA-125) levels are recommended beginning at age 25 to 35 years. For those women over the age of 35, a risk-reducing bilateral salpingo-oophorectomy is recommended, with possible short-term hormone replacement therapy.
Breast Cancer Prevention Strategies
Tamoxifen
Understanding of the role of estrogens and progesterones in breast cancer development has led to the development of pharmacologic strategies that could significantly decrease the incidence of breast cancer over the next two decades.
Several pharmaceuticals that affect the estrogenic pathways have been studied as chemopreventive agents, but the only agent for which mature data from clinical trials are available is tamoxifen.48,49–50,51–52,53 Interest in tamoxifen as a chemopreventive agent arose after a number of randomized trials designed to test the efficacy of hormonal therapy for invasive breast cancer reported that tamoxifen reduced the incidence of contralateral breast cancer. On the basis of these data, in 1992 the National Surgical Adjuvant Breast and Bowel Project (NSABP) began a randomized, placebo-controlled study (the P-1 trial) to test the efficacy of 5 years of tamoxifen in the prevention of breast cancer.53 Between 1992 and 1997, 13,388 women with a 1.67% or greater predicted risk of developing breast cancer within 5 years were enrolled in this trial. Risk was assessed using a modification of the Gail model, which permitted enrollment of any woman older than 60 years of age and selected women younger than 60 years with additional risk factors that increased their annual risk to at least that of a 60-year-old. In addition, women with a history of lobular carcinoma in situ (LCIS) were included. Women were not allowed to use estrogen replacement therapy during their participation in the trial. The results of the NSABP P-1 trial indicated that tamoxifen reduced the rates of invasive and noninvasive breast cancer by 49% and 50%, respectively. The benefit of tamoxifen was seen in all age groups (≤49 years, 50 to 59 years, ≥60 years). In addition, women with a history of atypical ductal hyperplasia had an 86% risk reduction, and women with a history of LCIS had a 56% risk reduction. Finally, the benefit was seen across all subgroups specified according to family history of breast cancer. Tamoxifen selectively reduced the incidence of estrogen receptor–positive tumors; estrogen receptor–negative tumors developed at an equal rate in the tamoxifen and placebo groups. No evidence was shown of a cardioprotective effect of tamoxifen in this trial, but the number of osteoporosis-related fractures was reduced in the tamoxifen-treated cohort. Tamoxifen increased the risk of developing stage I endometrial cancer (risk ratio of 2.53).
More recently, a landmark study was published that compared tamoxifen to raloxifene as a preventative agent in postmenopausal women with breast cancer. Raloxifene, a drug that is primarily used in prevention of osteoporosis, had been shown in prior studies to decrease the incidence of breast cancers. The NSABP study of tamoxifen and raloxifene was a prospective, double-blind, randomized clinical trial.54 There were 19,747 postmenopausal women of mean age 58.5 years with increased 5-year breast cancer risk. Patients were randomized to oral tamoxifen (20 mg per day) or raloxifene (60 mg per day) for 5 years. There were 163 cases of invasive breast cancer in women assigned to tamoxifen and 168 in those assigned to raloxifene, which was not significantly different between the two arms. The main benefit of raloxifene was in toxicity. There were 36 cases of uterine cancer with tamoxifen and 23 with raloxifene. No differences were found for other invasive cancer sites, for ischemic heart disease events, or for stroke. Thromboembolic events also occurred less often in the raloxifene group. The number of osteoporotic fractures in the groups was similar and there were fewer cataract surgeries with raloxifene. There was no difference in the total number of deaths (101 vs. 96 for tamoxifen vs. raloxifene) or in causes of death. It appears from this study that raloxifene is as effective as tamoxifen in reducing the risk of invasive breast cancer and has a lower risk of thromboembolic events. Of note there were slightly more noninvasive cancers in the raloxifene group, but that was not statistically significant
A comparison of the tamoxifen P-1, P-2, and other tamoxifen prevention trials is outlined in Table 56.4.48,49–50,51–52,55,56
Prophylactic Surgery
An alternative strategy used to prevent breast cancer development is prophylactic surgical intervention. Hartmann et al.57 analyzed outcomes in women with a family history of breast cancer who underwent bilateral prophylactic mastectomy at the Mayo Clinic between 1960 and 1993. With a median follow-up time of 14 years, only 4 of the 639 treated patients developed breast cancer. According to the Gail model, 37.4 cases of breast cancer would have been expected to develop in this population, so the prophylactic surgery resulted in an 89.5% risk reduction (P <.001).57
Breast cancer prevention strategies will continue to be a dynamic area of preclinical and clinical research.
TABLE 56.4 RESULTS OF CHEMO-PREVENTION TRIALS
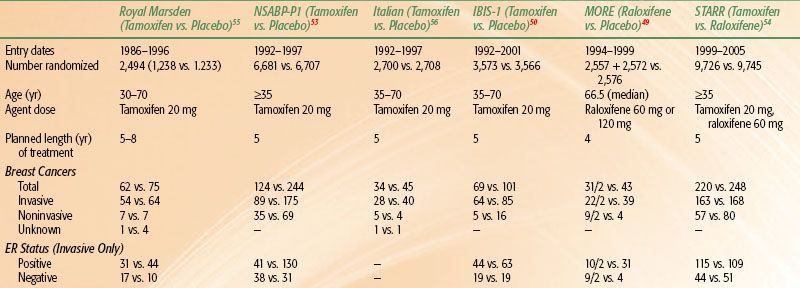
NATURAL HISTORY AND ORIGINS
All forms of breast cancer are believed to develop as a consequence of unregulated cell growth and the development of phenotypic changes such as the ability to invade, recruit a new blood supply, and metastasize. These changes in phenotypes are secondary to the development of aberrations in genetic pathways. Some of these aberrations are inherited (germline mutations), whereas others develop during the life of a breast cell (somatic mutations). It is currently believed that most breast cancer is a consequence of a series of somatic mutations. As previously noted, only 20% to 25% of breast cancer patients have a history of breast cancer in a first-degree relative. However, it is possible that some women without a first-degree relative with breast cancer still inherit a genetic background that predisposes to breast cancer. These mutations may be insufficient to cause breast cancer unless accompanied by other mutations and, therefore, would be predicted to have a low penetrance. Historically, it has been much more difficult to discover low-penetrance mutations than to discover germline mutations that result in an autosomal dominant pattern of breast cancer development. However, with newer molecular techniques, such as DNA-array assays, the identification of low-penetrance predisposing mutations may be more feasible.
Left untreated, breast cancer can have a variable clinical course. A classic article by Bloom et al.58 outlined the natural history of breast cancer patients, seen between 1805 and 1933, not treated by surgery or irradiation, 250 of whom had a pathologic diagnosis of cancer. There were no patients with stage I disease, 2.4% with stage II, 23% with stage III, and 74% with stage IV. Survival in the untreated group was 3.6% compared with an overall survival of 34% in patients treated with radical or modified radical mastectomy with or without radiation.
Concepts regarding the natural history of breast cancer have undergone great evolution over the past 100 years, with a profound impact on the management of these patients. The Halsted59 model was based on an orderly progression to the regional lymph nodes and from there to distant metastatic sites. Later, Keynes60 and Crile et al.61 suggested that breast cancer is a systemic disease and that extensive surgery to achieve local tumor control was not as important as originally believed. This alternative hypothesis was fully demonstrated in both laboratory and clinical studies by Fisher,62 who advanced the concept that breast cancer, as a systemic process involving host–tumor interactions, would not show substantial effects on survival with variations in locoregional treatment. A third hypothesis put forward by Hellman63 considers breast cancer as a heterogeneous disease with a spectrum extending from a tumor that remains localized throughout its course to one that disseminates systemically, even when detected as a small lesion, suggesting that metastases are a function of tumor growth and progression factors.
The growth rate of a tumor in the breast is thought to be constant from the date of origin. Using estimates of doubling time, it would take an average of approximately 5 years for a tumor to reach palpable size, and those lesions with slower doubling time would have an even longer latent period.64
The most common site of origin of breast cancer is the upper outer quadrant (38.5%), followed by the central area (29%), the upper inner quadrant (14.2%), the lower outer quadrant (8.8%), and the lower inner quadrant (5%).64 These rates correlate with the amount of breast tissue in the various quadrants. Cancer is somewhat more common in the left than in the right breast and may appear in both breasts simultaneously (1% to 2%). As noted above, women with a history of breast cancer have a 10% to 15% risk of developing a new primary in the contralateral breast.
As the cancer grows, it travels along the ducts, eventually breaking through the basement membrane of the duct, invading adjacent lobules, ducts, fascial strands, and the mammary fat, spreading through the breast lymphatics and into the peripheral lymphatics. The tumor can grow through the wall of blood vessels, spread into the deep lymphatics of the dermis, and eventually produce edema of the skin (peau d’orange), which usually indicates that the superficial as well as the deep lymphatics are involved. Skin dimpling can be caused by involvement of Cooper’s ligament. Ulceration and infiltration of overlying skin, which may develop late in the course of the disease, are usually preceded by fixation and localized redness of the skin over the tumor and are less frequently seen because of the current emphasis on screening and early diagnosis.64
TABLE 56.5 INTERNAL MAMMARY NODE INVOLVEMENT RELATED TO LOCATION OF PRIMARY TUMOR AND TO AXILLARY NODE INVOLVEMENTA
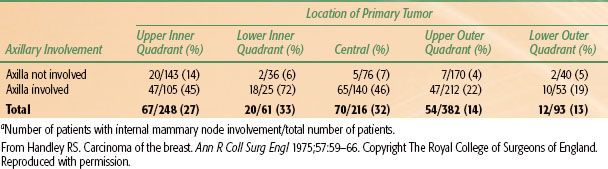
Axillary Spread
A common route of spread of breast carcinoma is first through the axillary lymph nodes, with the incidence increasing with larger tumors. Depending on mode of detection, tumor size, histology, and other clinical or pathological factors, between 10% and 40% of newly diagnosed stage T1 and T2 breast cancers have pathologic evidence of axillary nodal metastases. Voogd et al.65 assessed 7,680 patients with documented invasive breast cancer; of 5,125 patients known to have clinically negative lymph nodes who underwent axillary dissection, 1,748 (34%) had positive lymph nodes at pathologic examination. Univariate analysis showed that lymph node metastases were associated with tumors larger than 1 cm (P = .001), moderate or poorly differentiated nuclear grade (P = .005), high fraction of cells in the growth phase (S phase) of the cell cycle (P = .041), presence of lymphatic vascular invasion (P <.001), and age younger than 60 years (P = .01).
Multiple studies have shown a strong relation between primary tumor size and axillary nodal involvement.66–70 Even patients with T1a and T1b disease have significant nodal involvement. Mustafa et al.71 noted an overall frequency of axillary lymph node metastases in T1a and T1b lesions of 16%; integrating age, tumor size, and grade predicted the frequency of nodal metastases. Overall, patients with all three poor prognostic indicators had a 34% incidence of nodal involvement, and those with no poor prognostic factors had a ≤7% probability of nodal metastases. Gann et al.72 reviewed 18,025 patients with a diagnosis of breast carcinoma from the American College of Surgeons database. On multivariate analysis, the following factors were independently associated with a greater likelihood of one or more positive lymph nodes: larger tumor size, young age, African American or Hispanic race, outer-half tumor location, poor or moderate differentiation, aneuploidy, and infiltrating ductal histologic type.
Although up to 30% to 40% of T1 or T2 clinically node-negative breast cancers may have pathologically involved lymph nodes, data from NSABP-04 suggest that less than half of clinically negative but pathologically positive axilla will experience a clinical relapse in the axilla.73 In this study, operable breast cancer patients, who were primarily diagnosed with palpable breast tumors in the premammography era, were randomized to one of three arms: simple mastectomy without axillary dissection, simple mastectomy with axillary dissection, or simple mastectomy with comprehensive chest wall and regional nodal irradiation. In the arm undergoing axillary dissection, nodal positivity was approximately 40%. Nodal control was excellent (>97%) in this arm as well as in the arm treated with radiation. In the simple mastectomy arm, where no nodal treatment by radiation or dissection was administered, the axillary failure rate was approximately 20%. Assuming equal distribution among the arms, it is presumed the pathological involvement was approximately 40%, indicating that less than half of those with pathological involvement eventually failed clinically.
Internal Mammary Spread
Metastases to the internal mammary nodes (IMNs) are correlated with tumor size, are more frequent from medial half and central lesions, and occur more frequently when there is axillary node involvement (Table 56.5).74 A table with more detailed analysis of internal mammary involvement by tumor size, location and number of positive nodes is found in Chapter 57. Veronesi et al.75 found that, among women with tumors larger than 2 cm who were younger than 40 years of age and had positive axillary nodes, there was a 41% risk of having positive IMNs on IMN dissection; the corresponding risk for patients of that age with negative nodes was 16%. Sugg et al.76 reviewed 286 patients with breast cancer who underwent IMN dissection. Positive IMNs were associated with primary tumor size (P <.0001) and the number of positive axillary nodes (P <.0001), but not with age or primary tumor location. Patients who had positive IMNs (25% of all patients) had a significantly worse overall 20-year disease-free survival rate than did patients with negative IMNs (P <.0001). Clinical failure of the internal mammary nodes is extremely rare, despite the evidence of pathological involvement from these studies. Most studies looking at nodal failure patterns report failure in the internal mammary region of <1%.77–81
Supraclavicular Spread
Spread to supraclavicular lymph nodes usually follows involvement in the high axillary lymph nodes or IMNs depending on the location of the primary lesion. Chen et al.82 reviewed 2,658 patients with invasive breast cancer who underwent surgery and adjuvant therapy. With a median follow-up period of 39 months, supraclavicular lymph node metastasis developed in 113 (4.3%). Young age (≤40 years), tumor size >3 cm, angiolymphatic invasion, negative estrogen-receptor status, and DNA synthetic phase fraction >4% were significant for predicting supraclavicular metastasis on univariate analysis. Three predictive factors were significant after multivariate analysis: high histologic grade, more than four positive nodes, and axillary level II or III involved nodes. In patients with axillary level I involved nodes and four or fewer positive nodes, the incidence of supraclavicular lymph node metastasis was 4.4%, but if axillary level III was involved, it increased to 15.1%.
Clinical failure in the supraclavicular fossa is relatively rare in patients with early-stage breast cancer and is dependent on the degree of axillary involvement. For patients with no or minimal nodal involvement (less than three involved axillary nodes), supraclavicular failure is extremely rare. In an analysis of 691 patients with zero to three nodes involved undergoing breast-conserving surgery and radiation therapy to tangential fields only without regional nodal irradiation, Galper et al.78 reported failure in the supraclavicular fossa in 1.3% of patients.
Several studies have demonstrated that the failure rate in supraclavicular nodes, left untreated, may be as high as 20% in patients with advanced disease or more than four lymph nodes involved.83–86,87 In a cohort of 1,031 patients with operable breast cancer treated with mastectomy and level I or II node dissection plus adriamycin-based chemotherapy, but no radiation, Strom et al.87 reported failure in the supraclavicular fossa was 8% at 10 years. Predictors of supraclavicular failure included four or more involved nodes and gross extranodal extension. In these subgroups, supraclavicular failure ranged from 14% to 19%. Radiation to the supraclavicular fossa in these higher risk patients results in high local control rates, with isolated supraclavicular failures occurring in less that 1% of prophylactically treated nodes.
Systemic Spread
Using monoclonal antibodies to epithelial cytokeratins or tumor-associated cell membrane glycoproteins, carcinoma cells can be detected on cytologic bone marrow (or lymph node) preparations. Braun et al.88 combined patient data from nine studies involving 4,703 patients with stage I, II, or III breast cancer. Micrometastasis was detected in 30.6% of the patients. With a median follow-up of 5.2 years, patients with bone marrow micrometastasis had larger tumors and tumors with a higher histologic grade and more often had lymph node metastases and hormone receptor–negative tumors, compared with those without bone marrow micrometastasis. The presence of micrometastasis was a significant prognostic factor for poorer overall survival (RR 2.15; P <.001), breast cancer–specific survival (RR 2.44; P <.001), disease-free survival (RR 2.13), and distant disease-free survival (RR 2.33; P <.001 for all outcomes measures). In multivariable analysis, micrometastasis was an independent predictor of a poor outcome.
Local Control and Systemic Metastasis
Patients treated for breast cancer are at risk for local-regional failure, as well as systemic metastasis. It is evident from the available literature that optimizing local control can impact systemic metastasis and survival, and similarly, systemic therapy can impact local control.89–91,92 Integration of systemic therapy with radiation will be discussed in detail later, but numerous studies have clearly demonstrated a significant improvement in local control with the use of radiation therapy and systemic therapy (both cytotoxic and hormonal) compared with radiation therapy without the use of systemic therapy.92–97 Appropriate integration of both local and systemic treatments through a multidisciplinary approach is thus essential to optimize outcome. Although there is some overlap, prognostic factors for local-regional control and systemic metastasis often differ.
For patients with early-stage invasive breast cancer, even with appropriate systemic therapy, development of metastasis can vary from <5% in women with T1a disease and favorable histology, to >40% for women with T2 tumors and pathologically involved lymph nodes. Similarly, local-regional failure rates can vary from <5% to over 40% depending on local treatment and prognostic factors for local failure.93–95,96,98–100,101–102
The impact of local control on systemic metastasis in breast cancer as well as other malignancies has been the subject of considerable debate and controversy. Although the benefits of local control with respect to cosmesis and quality of life are apparent, the independent effect of local control on systemic disease and survival has been questioned. Several studies have identified local control as an independent predictor of disease-free or overall survival.99,102,103–106 Fisher et al.,103 in an analysis of patients treated in NSABP protocol B-06, concluded that ipsilateral breast tumor recurrence was a harbinger, but not a cause, of distant metastases. Although mastectomy or breast irradiation after lumpectomy prevented expression of the marker (breast relapse), neither lowered the risk of distant metastases, which was determined by a host of prognostic factors.
More recent meta-analyses, however, have demonstrated a small but significant impact of local control on systemic metastasis and overall survival.89,90 A meta-analysis of randomized trials by Vinh-Hung and Verschraegen90 comparing breast-conserving surgery without radiation to breast-conserving surgery with radiation confirms an approximate threefold reduction in local relapse with radiation therapy and an 8.6% improvement in mortality in the radiated cohorts.
One of the most convincing and authoritative studies related to this subject is the recent analysis of the Early Breast Cancer Trialists Collaborative Group (EBCTCG).89 In this analysis, over 42,000 women were enrolled in 78 randomized trials that compared 24 types of local treatment (radiotherapy vs. no radiotherapy, more vs. less surgery, or more surgery vs. radiotherapy). The EBCTCG attempted to relate the effect on local control to breast cancer mortality by grouping studies into whether the 5-year local relapse risk difference between the two comparisons of local therapy exceeded 10%. In those comparisons in which the difference in 5-year local recurrence risk was <10%, there was no impact on 15-year breast cancer mortality. However, there were 25,000 women enrolled in trials in whom the comparisons involved >10% differences in local control. In those studies, the difference in local recurrence risks at 5 years were 7% versus 26%, and the 15-year mortality risks were 44.6% versus 49.5% (P <.00001). Figure 56.6 summarizes the results of this meta-analysis with respect to the impact of radiation on breast cancer mortality in both breast conservation and following mastectomy. An update from the EBCTCG focused on 10,801 women enrolled in 17 randomized trials of radiotherapy versus no radiotherapy after breast-conserving surgery and relates the absolute reduction in 15-year risk of breast cancer death to the absolute reduction in 10-year recurrence risk.107 Overall, radiotherapy reduced the 10-year risk of any first recurrence from 35.0% to 19.3% (P <00001) and reduced the 15-year risk of breast cancer death from 25.2% to 21.4% (P <0001). In women with pN+ disease (n = 1,050), radiotherapy reduced the 10-year recurrence risk from 63.7% to 42.5% (P <00001) and the 15-year risk of breast cancer death from 51.3% to 42.8% (P = 01). Overall, about one breast cancer death was avoided by year 15 for every four recurrences avoided by year 10.
FIGURE 56.6. Effect of radiotherapy on breast cancer mortality and on all-cause mortality after breast-conservation surgery or after mastectomy with axillary clearance: 15-year or 20-year probabilities. (From Clarke M, Collins R, Darby S, et al. Effects of radiotherapy and of differences in the extent of surgery for early breast cancer on local recurrence and 15-year survival: an overview of the randomised trials. Lancet 2005;366:2087–2106, with permission from Elsevier.)
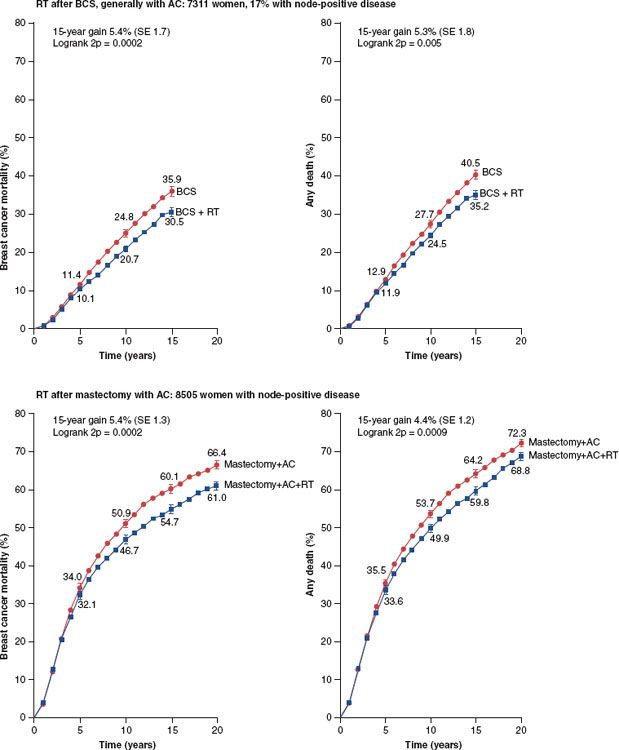
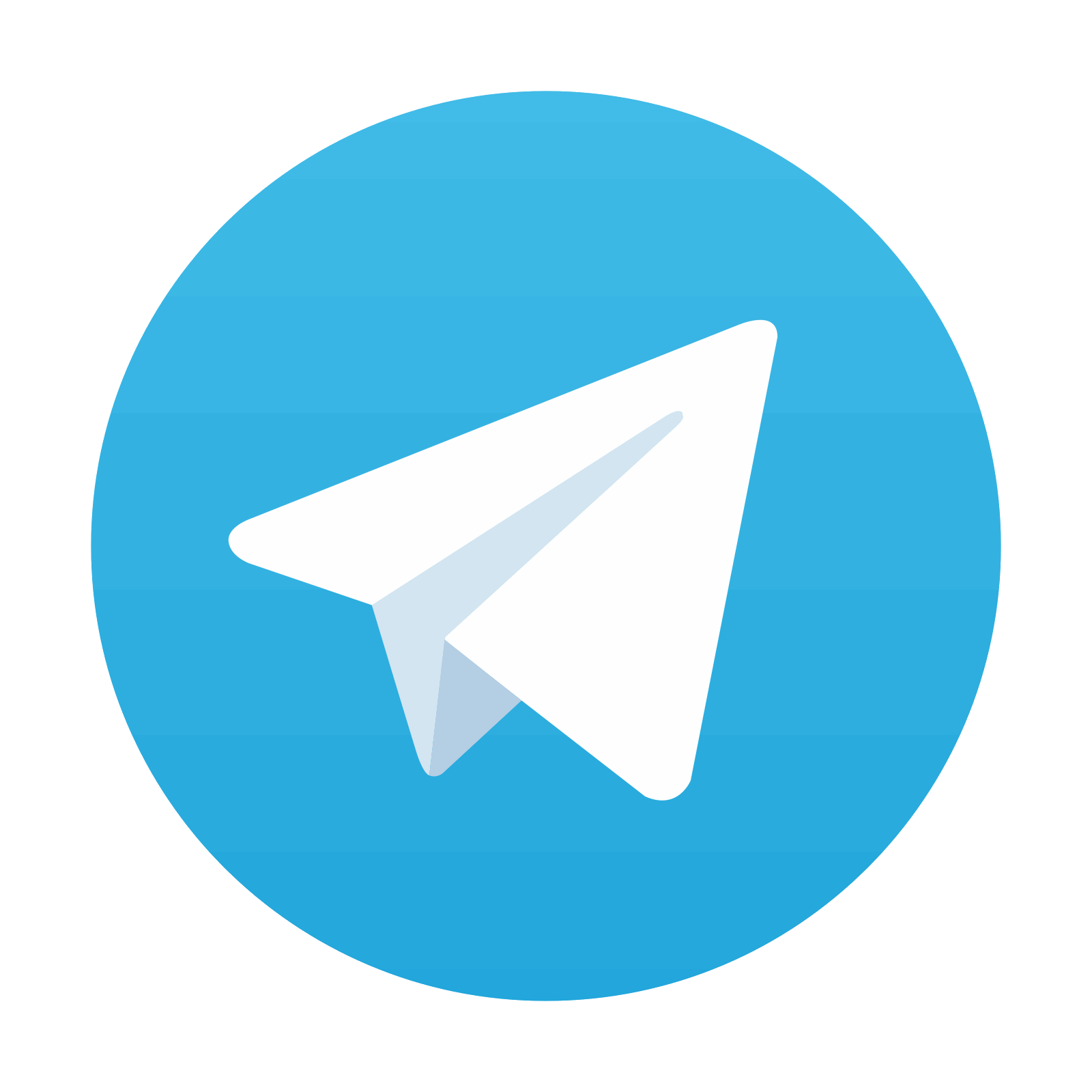