Fig. 10.1
Image of the Reference Point Indentation (RPI) Instrument, the first microindentation device to be utilized in human subjects for the measurement of indices of bone material properties in vivo. Reproduced from Hansma et al. (Rev Sci Instrum 2008;79(6):064303) with permission
To address these issues, these same investigators developed a new microindentation device that allows for the safe quantification of an index of bone material properties (without the need for a reference probe or displacement of the periosteum) in humans with minimal discomfort. This measure of bone material properties, later coined the “bone material strength index” or BMSI, can now be obtained using the OsteoProbe® (Active Life Scientific Inc., Santa Barbara, CA, USA), a small handheld microindentation instrument designed for in vivo bone material property measurements [33, 36]. Figure 10.2 shows the device positioned over the midshaft of the anterior tibia, the optimal testing site (determined by calculating the midpoint from the proximal end of the medial border of the tibial plateau to the distal edge of the medial malleolus). Indeed, this site provides a viable flat surface, away from tendons, ligaments, blood vessels, and nerves, for performing cortical bone material property measurements.
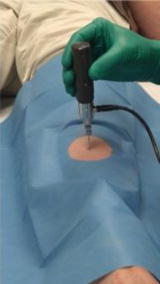
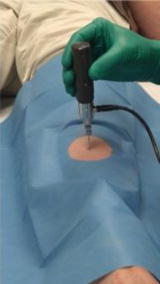
Fig. 10.2
Image of the OsteoProbe® (Active Life Scientific Inc., Santa Barbara, CA), a handheld microindentation instrument designed for in vivo measurements of the material properties of bone (i.e., bone material strength index [BMSI]) in humans at the midshaft of the non-dominant anterior tibia. Reproduced from Farr et al. (J Bone Miner Res 2014;29:787–95) with permission
Primary components of the OsteoProbe® include an impact mechanism, a displacement transducer, and a sterilized stainless steel disposable probe with a 90° conical tip (375 μm diameter; <10 μm tip sharpness radius). After administration of local anesthesia (1 % lidocaine), the probe is inserted through the skin, soft tissue, and periosteum until resting on the bone surface. While keeping the device perpendicular to the bone surface (within 10°), the measurement is started by slowly depressing the outer housing unit of the instrument, which in turn compresses the internal primary spring until the trigger mechanism initiates an impact. The impact mechanism creates a force (peak of 40 N) to drive the probe a minimal distance into the bone cortex, while the displacement transducer measures the indentation distance increase (μm) from impact. Thus, the BMSI is a direct in vivo measure of how well bone resists deformation in response to microindentation.
Accurate and precise in vivo measurements of BMSI are due to four basic operations: (1) pre-load, (2) trigger, (3) impact, and (4) unloading, as described previously [33]. Each operation occurs at different rates as indicated in the time (s; seconds) vs. force (N; newtons) graph shown in Fig. 10.3, which displays the forces imposed on the bone during each measurement cycle. Pre-load (operation 1) occurs over ~1 s when the operator slowly depresses the device’s outer housing unit to compress the internal primary spring. This ensures that the test probe is securely anchored to the bone before the primary indentation occurs. Once the pre-load force reaches 10 N, the trigger (operation 2) mechanism is automatically initiated, generating an impact (operation 3) lasting ~0.25 ms (with a peak force of 40 N and a constant impulse rate at 0.01 N s). After impact, unloading (operation 4) results in return of the force to 0 N and completion of the measurement cycle. A displacement transducer measures the indentation distance increase (μm) from impact, which is converted by computer to BMSI, defined as 100 times the ratio of the harmonic mean indentation distance increase from impact from five separate impacts into a polymethyl-methacrylate phantom relative to the indentation distance increase from impact into bone [36]. For each subject, BMSI is calculated as the average of 5–10 measurements, generally performed in a circular, rather than linear, fashion at different midshaft tibial sites (separated by >2 mm). It should be noted that the procedure causes minimal discomfort (only during the local anesthesia injection) and no complications have been observed to date. In fact, subjects report feeling only light pressure on the bone, but no pain, during the procedure, which typically takes less than 5 min to perform. Although minimally invasive, it should be noted that this procedure is not intended for patients who have a significant skin disorder, bruising, local edema, or infection, as well as those undergoing treatment for blood clots or severe coagulation defects.
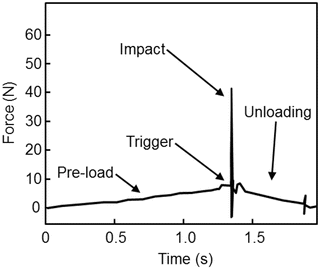
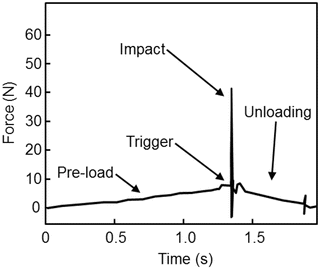
Fig. 10.3
Time (s; seconds) vs. force (N; newtons) plot showing a single OsteoProbe® measurement cycle including the four basic operations of the device: (1) pre-load, (2) trigger, (3) impact, (4) unloading. Adapted and reproduced from Bridges et al. (Rev Sci Instrum 2012;83:044301) with permission
The indentations are very small (on average, <200 μm), but do create minimal microcracks in the bone surface that are clinically insignificant and only detectable by scanning electron microscopy [33, 36]. Thus, BMSI is a direct measure of fracture resistance because the farther the probe indents the bone (higher the indentation distance from impact), the more easily bone is fractured (lower the BMSI). This technology has now been used in a number of relatively small clinical studies [25, 33, 36–39], and similar microindentation approaches have been utilized in previous studies involving animals [40–42] and humans [34, 35].
There is also considerable interest in how bone material properties relate to other measures of skeletal properties such as whole-bone mechanical properties and bone microarchitecture (see Chap. 9). For example, studies utilizing an animal model of T2DM found strong correlations between impaired bone material properties derived from an in vitro microindentation testing instrument, called the BioDent® (Active Life Scientific Inc., Santa Barbara, CA, USA), and reduced bone strength assessed by traditional ex vivo bone mechanical testing techniques (three-point bending and axial compression) at both appendicular and axial skeletal sites [42]. However, given the different loading patterns utilized by the OsteoProbe® (single-impact) and BioDent® (cyclic loading), these instruments likely provide different measures of bone material properties. Nonetheless, understanding how the outputs of these instruments relate to bone quality and to one another is of great importance toward their application in predicting fracture risk in both preclinical and clinical settings.
Although additional work is needed to answer these questions, some data are starting to emerge. For example, Granke et al. [43] recently tested the extent to which bone parameters derived from the BioDent® and OsteoProbe® were related to other measures of cortical bone quality in human cadaveric bone specimens. Based on measurements performed by both instruments at the same site, BMSI (derived from the OsteoProbe®) was inversely correlated with local cortical porosity (r = −0.69, P < 0.001), whereas none of the BioDent® parameters were related to any bone microarchitectural parameters. The authors concluded from these findings that the OsteoProbe® may be more sensitive than the BioDent® for measurement of aspects of cortical bone microarchitecture, in addition to its ability to assess bone tissue material properties [43]. This may reflect the greater force (~40 N) and depth (on average, ~200 μm) of the single-impact loading mechanism used by the OsteoProbe® vs. the BioDent® (force of ~20 N, depth of ~70 μm). Further, because fractures typically result from a single insult, it is possible that a one-time impact load more accurately reflects how bone responds to trauma as compared to a cyclic loading pattern of modeling. Future studies are needed to test these hypotheses experimentally.
There is also interest in how in vivo measures of bone material properties (e.g., obtained using the OsteoProbe®) relate to bone imaging parameters in humans, although this issue has received little attention to date. Interestingly, in T2DM patients [25], BMSI was not significantly (all P values >0.05) correlated with any radial or tibial cortical bone parameters (derived from HRpQCT) or any regional aBMD parameters (derived from DXA), suggesting that in patients with T2DM, bone material properties may be a major predictor of skeletal fragility independent of cortical bone microarchitecture and aBMD. Notwithstanding these preliminary findings, additional studies will be required to test this possibility in larger cohorts of both diabetic and nondiabetic women and men.
Bone Material Properties in Patients with T2DM
Whether patients with T2DM have compromised bone material properties as compared to nondiabetic persons has been a question of long-standing interest [16, 44]. To address this, we recently performed in vivo microindentation testing (using the OsteoProbe®) of the tibia to directly measure BMSI in 60 postmenopausal women (age range, 50–80 years): 30 patients diagnosed with T2DM for >10 years and 30 age-matched, nondiabetic women [25]. We also assessed bone microarchitecture of the distal radius and tibia by HRpQCT and regional aBMD by DXA. In addition, we examined the associations of BMSI with circulating glycated hemoglobin A1c levels. Compared to controls, T2DM patients had significantly lower BMSI: unadjusted (−11.7 %; P < 0.001; Fig. 10.4a) and following adjustment for BMI (−10.5 %; P < 0.001; Fig. 10.4b). These differences remained significant following additional adjustments for potential confounders, including hypertension and diabetic complications (data not shown). Interestingly, the mean glycated hemoglobin level over the previous 10 years was negatively correlated with BMSI (r = −0.41; P = 0.026) in patients with T2DM. By contrast, BMSI was not associated with a cross-sectional glycated hemoglobin level in nondiabetic control subjects (r = −0.09, P = 0.630). These findings thus represent the first demonstration of compromised bone material properties in patients with T2DM. In addition, our results highlight the potential detrimental effects of prolonged hyperglycemia on bone quality. We infer from these findings that the skeleton warrants recognition as another important target tissue subject to diabetic complications [25].
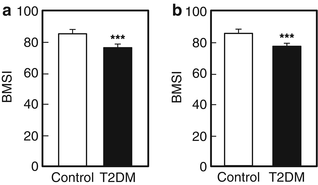
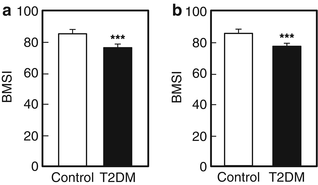
Fig. 10.4
Unadjusted (a) and body mass index (BMI)-adjusted (b) comparisons of bone material strength index (BMSI) between patients with type 2 diabetes mellitus (T2DM) and age-matched, nondiabetic controls. Values are shown as mean ± SE. ***P < 0.001. Adapted and reproduced from Farr et al. (J Bone Miner Res 2014;29:787–95) with permission
A valid concern of the OsteoProbe® instrument is safety. Notably, our group [25] and others have extensive experience using the device [25, 33, 36–39] and a closely related prototype [34, 35] in humans. Importantly, no complications have been reported at either the time of measurement or in follow-up. Nevertheless, as noted previously, out of an abundance of caution, patients with a significant skin disorder, bruising, local edema, or infection, as well as those undergoing treatment for blood clots or severe coagulation defects should not undergo this procedure. In addition, although the indentations are very small (on average <200 μm) and separated by at least 2 mm, it is likely preferable to perform the indentation in a circle rather than linearly down the tibia in order to minimize the extremely improbable, but theoretical [44], risk of stress fracture.
Pathogenesis of Reduced Bone Quality in T2DM
Because patients with T2DM have a higher fracture risk than nondiabetic persons despite having normal or even increased aBMD [4, 13, 14], they likely have impairments in bone quality. Our finding of reduced bone material properties in patients with T2DM [25] is consistent with this hypothesis, and points to important unanswered questions regarding the mechanism(s) of human diabetic bone disease. Studies in T2DM rodent models have identified advanced glycation end products (AGEs) among the primary proximal culprits [45]. Unfortunately, however, there are currently no T2DM animal models that sufficiently recapitulate the skeletal phenotype observed in human patients with adult-onset T2DM [46]. Thus, humans currently serve as the best model system for studying the pathogenesis of diabetic bone disease.
Although additional studies in living human subjects with T2DM are direly needed, as noted previously, most of our knowledge to date of diabetic bone disease in humans has been derived from cadaveric bone specimens. Nevertheless, findings from such studies have been critical in demonstrating that the increased risk of fragility fractures in patients with T2DM may stem from non-enzymatic glycation and collagen cross-links in diabetic bone, partially resulting from the increased production of AGEs. Because AGEs are recognized for their central role in mediating diabetic complications [45], these “bad” intermediate protein products have emerged as potentially viable therapeutic targets for preventing or perhaps reversing diabetic bone disease in humans [47].
AGEs are intermediate protein products that undergo undesirable chemical modifications after excessive glucose exposure [48]. Thus, prolonged high circulating glucose concentrations in T2DM may lead not only to higher glycated hemoglobin levels, but also to the accumulation of AGEs both in the circulation and in bone tissue itself [49]. Increases in AGEs negatively impact type I collagen as well as the generation and survival of osteoblasts in bone [16, 49, 50]. While enzymatic processes normally produce pyridinium crosslinks necessary for the mechanical integrity of collagen, non-enzymatic glycation creates undesired crosslinks that modify the extracellular matrix in bone and decrease bone formation [51–53]. Further, in vitro experiments have demonstrated that AGEs impair bone formation by interfering with osteoblast differentiation, attachment to bone surfaces, function, and survival [54–56]. Thus, the increased production of AGEs in T2DM likely leads to defects in bone formation and ultimately, due to coupling between bone formation and resorption, to low bone turnover.
While many different AGEs have been identified in human tissues [48], the most studied is pentosidine, circulating levels of which have been associated with clinical fractures in T2DM patients [57, 58]. N ε-carboxy-methyl-lysine (CML) is another dominant component of total AGEs [59], and its level in circulation has been related to osteoporosis [60] and hip fracture [61]. While commercial immunoassays have been developed to measure pentosidine in human serum, concerns exist regarding assay validity due to numerous factors in blood and urine that interfere with immunoassay standardization [62]. Thus, reverse phase high performance liquid chromatography is currently perhaps the most reliable method for the quantification of pentosidine in the circulation [63, 64].
To date, few studies have measured AGEs in human bone tissue, with most limited to cadaveric specimens obtained from nondiabetic human subjects. Although bone pentosidine is increased in nondiabetic fracture patients relative to non-fracture controls [65, 66] and has been shown to predict vertebral biomechanical properties (independent of BMD) in nondiabetic subjects [67], bone pentosidine levels have not been directly measured in vivo in T2DM patients. Furthermore, while pentosidine has conventionally been used as a surrogate biomarker of total AGE accumulation, it is only a single component of the total AGE content in bone [49]. Indeed, recent evidence suggests that pentosidine may not fully account for the overall influence of glycation on bone tissue [53].
To address this issue, the Vashishth laboratory has developed techniques to directly quantify total AGEs (ng quinine/mg collagen) via a fluorometric assay [68] and pentosidine (mmol/mol collagen) using ultra-high performance liquid chromatography (UPLC) methods [69] in human bone tissue specimens. Using these techniques, they showed that, in nondiabetic human cadaveric bone specimens, total bone AGEs are associated with bone mechanical properties, including yield strain (r = 0.54; P < 0.01), ultimate strain (r = 0.038; P = 0.09), and toughness (r =−0.39; P = 0.08). In addition, pentosidine is also associated with yield strain (r = 0.46; P < 0.05) and ultimate strain (r = 0.44; P < 0.05), but not toughness (r = −0.25; P > 0.05) [52]. While these findings provide potential insights into human diabetic bone disease, to our knowledge, no study has examined AGEs in bone tissue specimens obtained from living subjects with T2DM. Such studies may be pivotal toward unveiling the pathogenesis of skeletal fragility in patients with T2DM.
Future Directions
For many years, the lack of appropriate tools for noninvasively assessing critical aspects of bone quality significantly limited our understanding of skeletal deterioration in patients with T2DM. However, recent technological advances in high-resolution image acquisition and analysis, as well as assessment of bone material properties in vivo offer great potential for improving prediction of fragility fractures in this population. Despite the success and increasingly widespread use of novel tools such as HRpQCT and bone microindentation testing, additional work is needed to understand how the outputs of these instruments improve fracture prediction in patients. Furthermore, although our group previously demonstrated in patients with T2DM that cortical porosity is increased and that BMSI is reduced and correlates inversely with chronic glycemic control as assessed cross-sectionally [25], whether cortical porosity and BMSI deteriorate over time to a greater extent in diabetic vs. nondiabetic subjects remains unknown and are important issues that need to be resolved. This will require concurrently evaluating biomechanically relevant components of bone structure and quality in both cross-sectional and longitudinal studies of large numbers of women and men with and without T2DM using identical methodology. In addition, while it is plausible that glycemic control (as assessed by glycated hemoglobin) or AGEs (as measured in the circulation or in bone tissue) are associated with changes in bone material properties over time in patients with T2DM, this remains untested experimentally. Finally, further work is needed to establish the potential causal role of AGEs in mediating diabetic bone disease.
Conclusions and Working Model
In conclusion, considerable evidence indicates that the skeleton needs to be recognized as another important target tissue subject to diabetic complications. Our current understanding of the pathogenesis of skeletal fragility in diabetes suggests a working model (Fig. 10.5), whereby poor glucose control in patients with T2DM leads to increases in AGEs that have negative effects on osteoblasts, which in turn causes a reduction in bone formation. This defect in bone formation subsequently results in low bone turnover in T2DM patients, which prolongs the lifespan of type I collagen in bone, thereby leaving it particularly vulnerable to damage from increased AGEs. Ultimately, this creates a “vicious cycle” that may contribute to reduced bone quality and increased fracture risk in patients with T2DM.