Bone Form, Function, Injury, Regeneration, and Repair
Alexia Hernandez-Soria
Mathias Bostrom
Bone form consists of its cellular morphology, matrix composition, and circulation. Bone function, regeneration, and repair integrate cellular and macroscopic remodeling, biomechanics, material properties, metabolism, and age-related changes. Injury to bone can occur by way of osteonecrosis and fracture. These topics are addressed individually in this chapter.
Bone Cellular Morphology
Bone Structure
Structurally, bone is categorized as trabecular or cortical bone (Table 19-2). Porosity and architectural characteristics differentiate the two types of bone. These differences account for their respective material properties. Each structural bone type may exist as either or both types of microscopic bone, depending upon age, location, and setting (normal vs. pathologic bone).
Trabecular bone
Woven
Lamellar
Cortical bone
Compact bone: layers of lamellar bone without osteons; small animals
Plexiform bone: layers of lamellar and woven bone; large animals experiencing rapid growth
Haversian bone: vascular channels surrounded by lamellar bone (osteons); most complex type of cortical bone
Haversian Bone
Osteon/bone structural unit (BSU): the major structural unit of cortical bone; contains a central neurovascular canal surrounded by concentric lamellae
Table 19-1 Properties of Microscopic Bone
Property
Woven Bone
Lamellar Bone
Definition
Primitive; “immature” bone
Remodeled woven bone; “mature” bone
Found in
Embryo, infant, metaphyseal region, tumors, osteogenesis imperfecta, pagetic bone
Cortical bone, trabecular bone throughout the mature skeleton (most bone after the age of 4)
Composition
Dense collagen fibers, varied mineral content
Formed by intramembranous or endochondral ossification; contains collagen fibers
Organization
Randomly arranged collagen fibers
Highly ordered; stress-oriented collagen fibers
Response to stress
Isotropic: independent of direction of applied forces
Anisotropic: mechanical behavior differs according to direction of forces; bone’s greatest strength is parallel to longitudinal axis
Table 19-2 Bone Structure
Property
Trabecular
Cortical
Description
Spongy and cancellous bone
Dense or compact bone; <30% porosity
Location
Metaphysis or epiphysis (long bone); cuboid bone (vertebrae)
Diaphysis (long bone); outer layer, “envelope,” of cuboid bone (vertebrae)
Architecture
Individual trabeculae organized into 3-D lattice of rods and rods, rods and plates, or plates and plates
Rods = thin trabeculae
Plates = thick trabeculae
Lattices orient in response to stress.
Plexiform bone: layers of lamellar and woven bone. Contains vascular channels and allows for rapid growth and accumulation of bone.
Haversian bone: arrangement of osteons surrounded by lamellae
Mechanical stress
Predominantly subjected to compressive forces
Subjected to bending, torsional, and compression forces
Porosity
50% to 90% (large spaces between trabeculae)
∼10% (dependent on density of voids in architecture)
Apparent densitya
0.30 g/cm3 (std 0.10 g/cm3 ± 30%)
1.85 g/cm3 (std 0.06 g/cm3 ± 3%)
aApparent density = mass of bone tissue/bulk volume of tissue (bulk volume = bone + bone marrow cavities); std = standard deviation
Haversian canal: central canal of the osteon; contains cells, haversian vessels, and nerves
Volkmann’s canals: connect haversian canals of neighboring osteons
Howship’s lacunae: resorptive cavities within which reside osteoclasts
Canaliculi: channels within and between osteons; allow communication and waste removal between developing cells of lamellae and haversian vessels
Haversian Vessels
Most are structurally similar to capillaries. They contain a base membrane that functions as a selective-ion transport barrier important for Ca2+ and P2- transport and response of bone to mechanical loads.
The small vessels are similar to lymphatic vessels, which do not have a base membrane. These vessels contain only precipitated protein.
Bone Cells
Osteoblastic Lineage
Three types of cells of osteoblastic lineage are each derived from pleuripotential mesenchymal stem cells (Table 19-3).
Osteoclastic Lineage
Osteoclasts differentiate from pleuripotential hematopoietic stem cells (Table 19-4).
Circulating monocytes develop into osteoclasts at resorption sites.
Cellular Mechanisms of Remodeling
Throughout life old bone is continually replaced by new bone. The process of remodeling and bone formation is regulated by cellular mechanisms of osteoblasts and osteoclasts.
Table 19-3 Properties of Osteoblastic Lineage Cells | ||||||||||||
---|---|---|---|---|---|---|---|---|---|---|---|---|
|
Table 19-4 Properties of Osteoclasts | ||||||||
---|---|---|---|---|---|---|---|---|
|
Remodeling
Remodeling is the process of resorption of immature (woven) and old bone followed by the formation of new lamellar bone.
There are two forms of remodeling:
Endochondral remodeling: converts primary bone to secondary bone (cartilage to trabecular bone); believed to decrease density of trabecular bone
Haversian remodeling: repairs fatigue damage to skeleton, maintains bone mineral homeostasis, and thought to be necessary for maintaining viability of cells far from bone surfaces. Increases porosity of cortical bone, decreases width, and believed to decrease strength.
Both endochondral and haversian remodeling follow the same six-stage sequence of events: resting, activation, resorption, reversal (coupling), formation, and mineralization. The process is regulated by mechanical and systemic factors.
Stages of Remodeling
Resting
At any time, about 80% of human bone surface (perios-teal and endosteal) is resting.
Bone surface is lined with an endosteal membrane and resting bone lining cells believed to be involved in bone mineral homeostatic mechanisms.
Activation
The exact factor of activation is unknown.
In response to regulators, osteoclasts are recruited and given access to a section of bone surface. It is believed that a capillary extends to the surface, delivering osteoclast precursors (monocytes).
Bone lining cells digest the endosteal membrane before retracting to allow the osteoclasts access to the mineralized bone (osteoclast regulation factors are summarized in Box 19-1).
Resorption
Resorption leads to formation of Howship’s lacunae in cancellous bone and cutting cones (resorptive cavities or Haversian canals) in cortical bone.
The process lasts about 14 days. The osteoclast precursors are thought to coalesce at the bone’s surface to form large multinucleated osteoclasts.
The osteoclasts attach to the surface, forming a “clear zone” under their ruffled border.
In a concerted mechanism, the bone surface is solubi-lized.
Concerted mechanism: Cathepsin B and acid phosphatase are released in “clear zone.” Carbonic acid is reduced by carbonic anhydrase intracellularly. H + is released into the “clear zone” until pH reaches ∼3.5 and environment is acidic enough to degrade mineralized bone.
Pyridinolines cannot be degraded by resorption and are released into extracellular fluid. Serum and urine levels can be measured for monitoring resorption.
Reversal/Coupling
A 28-to 35-day interval between resorption and formation of bone, which can be noted histologically by an absence of osteoclasts in Howship’s lacunae and cutting cones
Surfaces are lined with mononuclear cells to prepare the surfaces for new bone formation. A glycoprotein layer (cement line), placed over the surface, is thought to facilitate attachment by new osteoblasts. The mechanisms by which this occurs are not known.
This time interval is considered the time when bone formation is “coupled” with resorption due to the perceived hormonal and cellular mechanisms of communication among the bone resorbing and forming cells.
Box 19-1 Osteoclast Regulation Factors
Receptor activator of NF-K β(RANK)/receptor activator of NF-k β-ligand (RANKL)
Osteoblasts produce protein RANKL.
RANKL is received by cell surface receptor, RANK, on precursor osteoclasts.
RANK + RANKL stimulates osteoclasts’ development and bone resorption.
Osteoprotegerin (OPG)
Decoy RANKL receptor
OPG inhibits osteoclast development and increases bone mass.
Parathyroid hormone (PTH)
Stimulates number of osteoclasts found in bone
PTH is correlated with increased RANKL and decreased OPG expression.
PTH receptors are found on osteoblasts.
PTH stimulates osteoblast production of interleukin-6 (IL-6) and IL-6 stimulates osteoblast production of IGF-1.
Calcitonin
Directly inhibits bone resorption
Calcitonin receptors found on osteoclasts
Thyroid hormone
Hyperthyroidism increases bone resorption.
Vitamin A
Has been shown to stimulate resorption
Growth Factors
Resorption-stimulating growth factors:
EGF/TGFα, PDGF, IGF-1, and FGF
Osteoclasts have receptors for IGF-1.
TGFβ has been shown to inhibit osteoclast formation.
Cytokines
IL-1, IL-6, and tumor necrosis factor are known to be involved in mediating osteoclast differentiation and bone resorption.
Formation and Mineralization
The two aspects of bone formation occur in this stage: matrix synthesis followed by mineralization.
The new osteoblasts deposit a new layer of unmineralized bone matrix, the osteoid seam.
The osteoid seam will reach ∼70% mineralization in 5 to 10 days and complete mineralization in about 4 months (in cortical and trabecular bone).
Bone modeling-dependent bone loss: An adult BSU will mineralize 5% less bone than it resorbed.
Matrix Synthesis
Osteoblasts secrete many types of collagenous and non-collagenous macromolecules, which are all proteins.
The secretions include all critical structural elements for osteoid in both trabecular and cortical bone.
The unique architecture differentiating bone types is due to the particular organization of the secreted proteins.
All proteins, and thus matrix organization, are regulated on a genetic level.
Most matrix proteins undergo some form of post-transla-tional processing, which allows matrix regulation without interfering with gene expression.
Collagen cross-linking
Glycosylation to produce proteoglycans and glycoproteins
Phosphorylation: produces osteopontin, bone sialoprotein
Vitamin K dependant -y-carboxylation: produces osteocalcin
Many proteins have specific functions related to their surface receptors or adhesion and anti-adhesion properties.
Osteoblasts secrete proteins according to matrix formation.
Fibronectin and osteonectin are secreted early in formation.
Osteocalcin (calcium binding protein) is secreted only after matrix is formed.
Mineralization
Mineralization is the process by which bone crystals grow and proliferate within the holes and pores of the matrix collagen fibers.
The bone crystals are composed of an analog of hydroxy-apatite (HA). The HA in matrix leads to the rigidity of bone.
Mineralization is regulated by the spaces and orientation of matrix collagen fibers (pores and holes) and by the noncollagenous matrix proteins.
The noncollagenous proteins can function both as nucleators and inhibitors of crystal formation.
There is a lag time of —14 days between matrix formation and mineralization. The time is thought to be regulated by noncollagenous proteins and allows matrix to “mature;” strengthened by collagen cross-linking, resulting pyridinolines (pyridinoline, deoxypyridinoline, hydroxylysylpyridinoline, lysylpyridinoline).
Due to lag time, there is always an osteoid seam between the osteoblasts and newly mineralized bone.
Regulation of Remodeling
The bone remodeling sequence is intricately controlled by endocrine hormones as well as local paracrine and auto-crine factors. The systemic and local factors that mediate bone formation are listed in Box 19-2 (also see Chapter 10, Metabolic Bone Diseases).
Box 19-2 Regulators of Bone Formation
Systemic factors
Growth hormone/insulin-like growth factor
Thyroid hormone
Vitamin D
Estrogens
Glucorticoids
Parathyroid hormone
Local factors
IGF-1
Transforming growth factor beta
Bone morphogenetic protein
Fibroblast growth factors
Parathyroid hormone-related peptide
Prostaglandins
Platelet-derived growth factor (VEGF)
Transcription factors
Core binding factor (Cbfa1/Runx2)
Osterix (transcription factor for osteoblast differentiation)
Resorption Regulation
Regulating bone resorption necessarily focuses on mediating osteoclast proliferation and activity. The exact mechanisms of interaction remain under investigation. The roles of systemic and local factors in osteoclast regulation are summarized in Chapter 10.
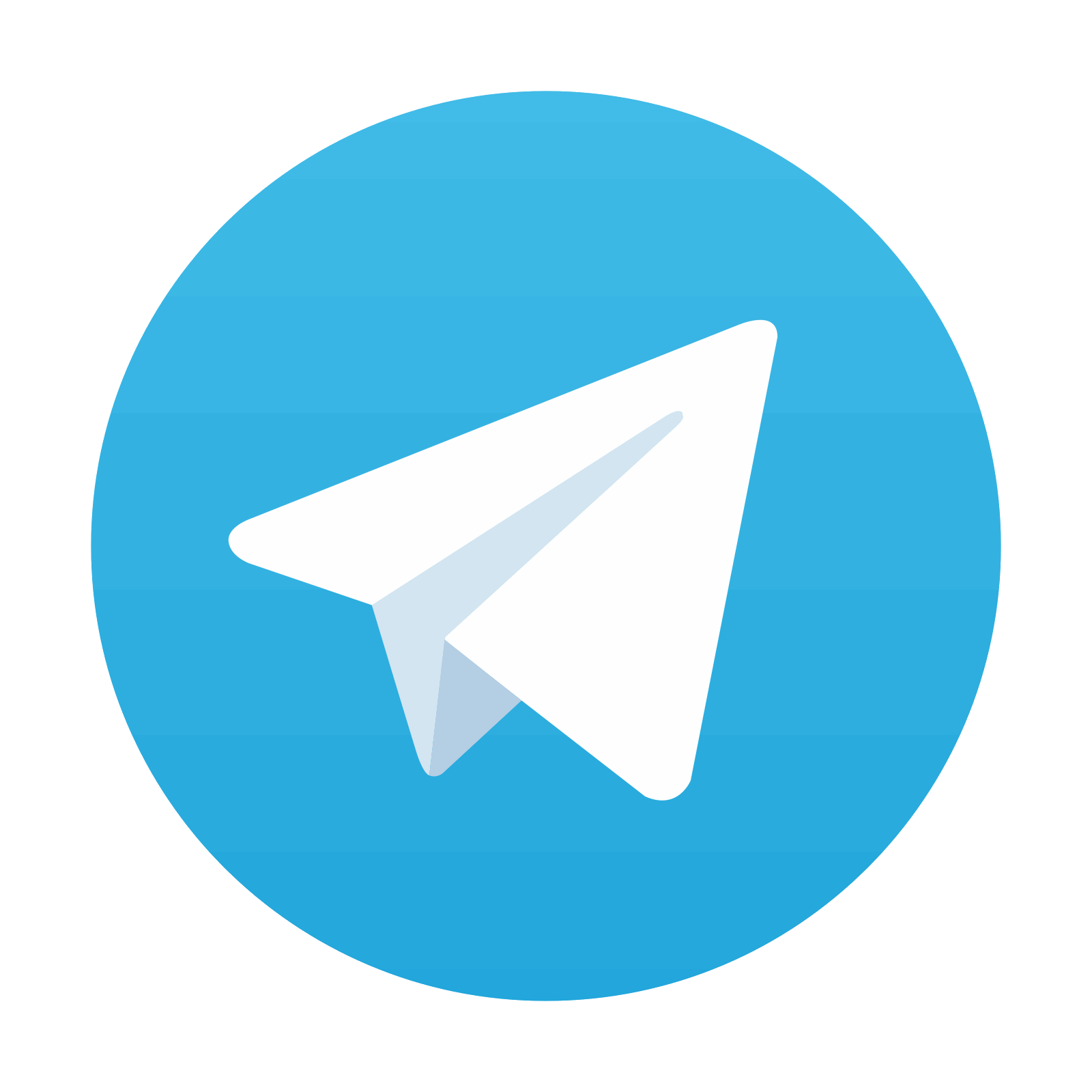
Stay updated, free articles. Join our Telegram channel

Full access? Get Clinical Tree
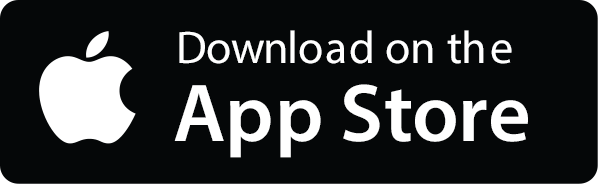
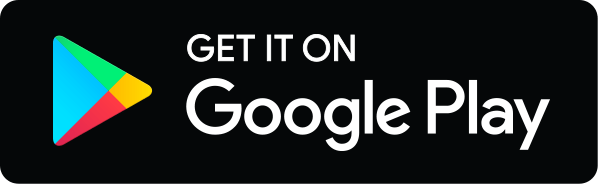
