Abstract
The discipline of Transfusion Medicine (also known as “Blood Banking”) includes: (i) the collection, testing, processing, and preparation of blood and blood components; (ii) the selection of the most appropriate products and transfusion practice based on laboratory findings and patient need; and (iii) the monitoring of the effectiveness of transfusion as modified by disease, physiological status, or the procedure(s) performed. Certain patient groups (e.g., neonates, oncology patients, hematopoietic progenitor cell transplant recipients, those with sickle cell disease, and others); often require complex pretransfusion processing and specialized product selection and modification.
Keywords
Transfusion medicine, Blood Banking, red blood cells, fresh frozen plasma, sickle cell disease, leukocyte reduction
Introduction
The discipline of Transfusion Medicine (also known as “Blood Banking”) includes the:
- 1.
Collection, testing, processing, and preparation of blood and blood components.
- 2.
Selection of the most appropriate products and transfusion practice based on laboratory findings and patient need.
- 3.
Monitoring of the effectiveness of transfusion as modified by disease, physiological status, or the procedure(s) performed.
Certain patient groups (e.g., neonates, oncology patients, hematopoietic progenitor cell transplant recipients, those with sickle cell disease, and others); often require complex pretransfusion processing and specialized product selection and modification.
Donor Selection and Collection
The US Food and Drug Administration (FDA) regulates donor selection, collection, testing, component preparation, storage, and distribution of blood and blood components through the Code of Federal Regulations. The American Association of Blood Banks regularly publishes standards for blood banks and transfusion services, technical bulletins, and a technical manual, which direct donor and patient transfusion practices and procedures. Outside of the United States, similar regulatory agencies oversee production, transfusion, and posttransfusion complications through biovigilance networks.
Infectious disease testing should include serological testing for human immunodeficiency virus (HIV), hepatitis B and C, human T lymphotrophic virus I/II, and syphilis and nucleic amplification testing for HIV, hepatitis C, and West Nile virus. Although not currently required by the FDA, testing for Chagas disease ( Trypanosoma cruzi ) is also performed. Cytomegalovirus (CMV) testing is not routinely performed. Because of the possibility of bacterial contamination of platelet products, different methods of bacterial testing for platelets are also performed. Donor deferrals or other testing may be indicated, depending on national or international infectious disease prevalence. An example includes variable and changing donor travel deferrals for malaria and Chikungunya virus. Testing for Dengue virus and babesia may be performed if these disorders are endemic to a specific geographical location.
Component Preparation
Whole-Blood-Derived Products
In the United States, the majority of red blood cells (RBCs) and plasma products (including fresh frozen plasma (FFP), thawed plasma, and other plasma products) are derived from the donation of whole blood. In Canada and Europe, whole-blood-derived platelets are prepared using an alternative “buffy coat” method. Figure 36.1 outlines different whole-blood-derived blood components. Depending on the type of processing, one whole-blood unit can generate one unit each of RBCs, platelet concentrate, and plasma. In addition, subsequent thawing and precipitation of FFP at 1–6°C generates a unit of cryoprecipitate.
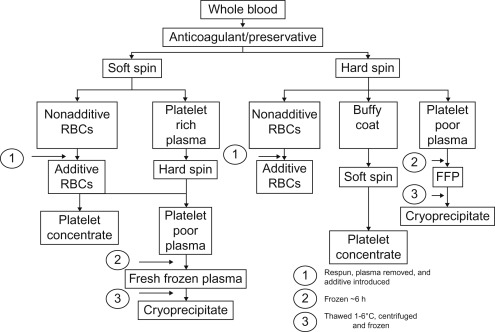
Apheresis-Derived Products
The same products obtained from whole-blood processing may also be obtained using apheresis techniques. Apheresis techniques provide the ability to obtain up to 12 equivalent donor whole-blood platelet concentrates, a double unit of RBCs, or up to 500 or 600 ml of plasma depending on donor weight. Granulocytes are also obtained using apheresis methods. The use of sterile connecting devices and specialized bag configurations permit these apheresis products to be divided into standard or even smaller units, especially valuable for infants and children.
RBC and Whole-Blood Products
Whole blood is collected into bags containing one of several anticoagulant preservative solutions; these include acid citrate dextrose solution, citrate-phosphate-dextrose solution, citrate-phosphate-double-dextrose solution, and citrate-phosphate-dextrose-adenine solution. Additive solutions are often added to the anticoagulated RBC product to increase shelf life. These solutions are each slightly different in their additive constituents and include AS-1, AS-3, AS-5, and AS-7 ( Table 36.1 ). In Europe, Canada, and other countries, an alternative additive solution, SAG-M, may be used. Toxic levels of these anticoagulant preservative and additive solutions are only reached in patients undergoing massive transfusions or extracorporeal membrane oxygenation (ECMO) ( Table 36.2 ). Different methods to reduce the anticoagulant additive solutions are utilized by transfusion services.
Constituent | CPDA-1 a | CPD | AS-1 b | AS-3 b | AS-5 b | AS-7 b | SAG-M b |
---|---|---|---|---|---|---|---|
Volume (ml) | 100 | 63 | 100 | 100 | 100 | 100 | 100 |
Sodium chloride (mg) | 0 | 0 | 900 | 410 | 877 | 0 | 877 |
Dextrose (mg) | 2000 | 1610 | 2200 | 1100 | 900 | 1585 | 820 |
Adenine | 17.3 | 0 | 27 | 30 | 30 | 27 | 17 |
Mannitol | 0 | 0 | 750 | 0 | 525 | 1000 | 0 |
Trisodium citrate (mg) | 1660 | 1660 | 0 | 588 | 0 | 0 | 0 |
Citric acid | 206 | 206 | 0 | 42 | 0 | 0 | 0 |
SODIUM PHOSPHATE | |||||||
Monobasic (mg) | 140 | 140 | 0 | 276 | 0 | 0 | 0 |
Dibasic (mg) | 0 | 0 | 0 | 0 | 0 | 170 | 0 |
Shelf life of WB units | 35 | ||||||
Shelf life of RBC units c | 35 | 28 | 35–42 | 35–42 | 35–42 | 35–42 | 35–42 |
a Approximately 450 ml of donor blood is drawn into 63 ml CPDA-1. A unit of RBCs (Hct ~70%) is prepared after centrifugation and removal of most plasma.
b When AS-1, AS-5, AS-7 or SAG-M is used, ~450 ml of donor blood is drawn into CPD. When AS is used, ~450 ml of donor blood is drawn into citrate-phosphate-double-dextrose solution which differs from CPD in containing, double the amount of dextrose. After centrifugation and removal of plasma, the RBCs are resuspended in 100 ml of the additive solution with final Hct 55–60%.
c Approved shelf life varies depending on country’s regulatory agency.
Additive | CPDA-1 | AS-1 | AS-3 | SAG-M | ECMO | Toxic dose b |
---|---|---|---|---|---|---|
mg/kg | mg/kg | mg/kg | mg/kg | 2 units | mg/kg/h | |
NaCl | 0 | 28 | 5 | 35.6 | 641 | 137 |
Dextrose | 13 | 86 | 15 | 20.4 | 1982 | 240 |
Adenine | 0.2 | 0.4 | 0.4 | 0.2 | 18 | 15 |
Citrate | 12 | 6.5 | 8.4 | 6.5 | 148 | 180 |
Phosphate | 9 | 1.3 | 3.7 | 1.3 | 31 | >60 |
Mannitol | 0 | 22 | 0 | 0 | 500 | 360 |
a Assuming 60% Hct for CPDA-1, AS-1, AS-3, and SAG-M units.
b Toxic dose actually difficult to predict since adenine, dextrose, and phosphate enter RBCs for metabolism.
In some situations whole-blood units (reconstituted from RBCs and compatible plasma) may be necessary. Reconstituted whole blood may be needed as a prime for apheresis procedures in very small patients or for whole-blood exchange for hemolytic disease of the fetus and newborn (HDFN) in order to avoid iatrogenic dilution-induced coagulopathy. There are no platelets in reconstituted whole-blood units. To monitor bleeding risk associated with thrombocytopenia, a platelet count should be obtained.
Plasma Products
The following types of plasma are available from transfusion services.
- 1.
FFP, prepared after separation of whole blood or apheresis and frozen at ≤−18°C within 6 h of preparation.
- 2.
PF24 (plasma frozen within 24 h after phlebotomy), prepared from a whole blood or apheresis collection and stored at 1–6°C within 8 h of collection and frozen at ≤−18°C.
- 3.
PF24RT24 (plasma frozen within 24 h after phlebotomy held at room temperature up to 24 h after phlebotomy), prepared from apheresis collection.
Each of these plasma products is considered to be equivalent in terms of clinical efficacy, with similar concentrations of coagulation and anticoagulation proteins. Delayed freezing reduces the concentration of factors V and VIII, protein C (PF24 only), and protein S (PF24RT24 only) making their use in clinical circumstances where these proteins would be beneficial contraindicated.
All plasma products thawed in an “open system” must be discarded after 24 h to minimize bacterial contamination. If the plasma product has been thawed in a closed system, storage for up to 5 days at 1–6°C is allowed, but the product must be relabeled as thawed plasma. There are no well-designed clinical trials in neonates or critically ill children demonstrating risk–benefit of one plasma product over another.
Platelet Products
Platelets are derived from whole blood or by apheresis. In the United States, whole-blood-derived platelets are prepared from a second “hard spin” of platelet-rich plasma. Platelets have a shelf life of 5 days (in some countries up to 7 days) and must be stored at 20–24°C. In Europe, Canada, and some other countries platelets may be prepared from whole blood using a “buffy coat” method ( Figure 36.1 ; Table 36.3 ). Clinical studies comparing different platelet preparations have evaluated posttransfusion platelet increments, adjusting for patient blood volume and platelet product content. Few pediatric studies have been performed to assess efficiency, especially in the bleeding patient.
Apheresis platelets | Whole-blood-derived (platelet-rich plasma) | Whole-blood-derived (buffy coat method) a | |
---|---|---|---|
Donor exposure | Lower | Higher | Higher |
Percentage of repeat donors | Higher | Lower | Lower |
Compatibility with cost-effective, sensitive bacterial detection methods | Yes | No (unless prestorage pooling) | No (unless prestorage pooling) |
Compatibility with pathogen reduction technologies | Yes | No (unless prestorage pooling) | No (unless prestorage pooling) |
Hospital preparation (pooling, point-of-care bacterial testing) | Less | More | More |
Transfusion service paperwork | Less | More | More |
Wastage | Less | More | More |
Febrile, nonhemolytic transfusion reactions | Fewer | More | More |
Platelet activation | Lower | Highest | Lowest |
Single donor plasma exposure | Higher than whole blood derived | Reduced | Reduced |
Cost of production | Higher | Medium | Low |
Availability | Low | High | High |
In a large multicenter trial to evaluate platelet dosing, it has been shown that there are differences in bleeding risk between adults and children receiving platelets for hypoproliferative thrombocytopenia. Children, particularly those undergoing hematopoietic stem cell transplantation (HSCT), were at a higher risk of bleeding than adults over a wide range of platelet count. Grade 3 or greater bleeding occurred most commonly in children 6–12 and 13–18 years of age regardless of HSCT.
The FDA recently approved the use of a platelet additive solution (PAS) for apheresis platelets. PAS solutions contain acetate or glucose as a substrate for platelet metabolism, phosphate to buffer lactate production, citrate to prevent coagulation and lactate production and potassium and magnesium to improve platelet function during storage. Theoretical advantages include improved in vivo and in vitro platelet quality, increased duration of storage, reduction of allergic reactions, and ability to use out-of-group platelets.
Cryoprecipitate
Cryoprecipitate is prepared by thawing whole-blood-derived FFP between 1 and 6°C and recovering the precipitate through centrifugation. The cold-insoluble precipitate is placed in the freezer within 1 h after removal from the refrigerated centrifuge then thawed for use. Cryoprecipitate contains high levels of fibrinogen (>150 mg), factor VIII (>80 IU), factor XIII, vWF, and fibronectin. Current indications include congenital and acquired hypofibrinogenemia, and factor XIII deficiency when viral-inactivated, monoclonal or otherwise viral-inactivated manufactured factor concentrates are not available or are contraindicated.
Granulocytes
Granulocytes are collected using apheresis technology and are not an FDA-licensed product. Donors may be stimulated with oral dexamethasone or subcutaneous granulocyte colony-stimulating factor (G-CSF) and undergo apheresis to collect at least 1×10 10 white blood cells (WBCs) per unit. Indications include a febrile, neutropenic patient with severe bacterial or fungal infection unresponsive to antifungals or to antibiotics and whose anticipated course will result in neutrophil recovery. Granulocytes may also be indicated in patients with neutrophil function defects (e.g., chronic granulomatous disease, leukocyte adhesion deficiency), who are unresponsive to antibiotics or antifungal agents for sepsis or acute infectious complications. Rigorous clinical trials for dosing are not available. Specialized consent and approval by the institutional review board may be required for use of this product as a clinical trial due to unresolved issues of risk–benefit for this product. The Resolving infection in Neutropenia with Granulocytes Study is a phase IIII randomized study of adjunctive granulocyte transfusion in septic patients undergoing standard antimicrobial therapy, which was published in 2015, was under powered and did not answer whether transfusion of dexamethasone or G-CSF-stimulated granulocytes has added benefit in septic children and adults. The decision to use granulocyte transfusion should be taken on a case by case basis, weighing the potential risks and benefits.
Complications of granulocyte transfusion include acute and chronic CMV and other viral infections for which testing is not performed or is unavailable, as well as human leukocyte antigen (HLA) alloimmunization since the product cannot be leukoreduced. Exposure to a large burden of foreign HLA antigens through WBC transfusion may contribute to the development of subsequent platelet refractoriness and a reduced likelihood of hematopoietic stem cell engraftment. Granulocytes contain a significant number of RBCs (>30 ml with a hematocrit generally between 5% and 15%), and therefore must be crossmatch compatible against a patient’s specimen and can, if administered frequently, result in iatrogenic polycythemia.
Granulocyte concentrates have historically been irradiated to obviate the risk of graft-versus-host disease (GVHD). A study of adult acute myeloid leukemia (AML) patients has proposed that nonirradiated granulocytes are superior to irradiated granulocytes for the control of bacterial infections. The shelf life of the product is limited to only 24 h. This increases the risk of transfusion-transmitted infections as time-consuming donor infectious disease screening is often not available when the product is transfused.
Blood Component Modifications
Leukoreduction
In Europe, Canada, and several other countries, universal leukocyte reduction (LR) has been adopted as a standard for all cellular blood components except granulocytes. RBCs typically contain up to 10×10 9 WBC and can be leukoreduced either by filtering whole blood at the beginning of processing (prestorage LR) or by attaching a filter with a sterile connecting device at the bedside to a unit of RBCs pretransfusion ( Table 36.4 ). There are different approaches to the timing of filtration; at the bedside ensures that the filtration process has been observed while filtration in the blood bank allows for the monitoring and to control the WBC content postfiltration. Current LR filters reduce the number of WBCs a thousandfold so that the residual WBC count does not exceed 5×10 6 per packed RBC unit (United States) or 1×10 6 (Europe) while retaining 85% of the original red cell content. Transfusion services have developed quality control methods to ensure meeting these standards. LR should never be used as a strategy for the prevention of transfusion-associated graft-versus-host disease (TA-GVHD).
Component/product (volume) | Composition | Storage length | Storage conditions | Usual indications |
---|---|---|---|---|
Red blood cells (RBCs) (in citrate-phosphate-dextrose solution, citrate-phosphate-double-dextrose solution, citrate-phosphate-dextrose-adenine anticoagulant (CPDA-1), ~250 ml) | RBCs (~75% Hct); 50–70 ml plasma, 10 9 –10 10 white blood cells (WBCs) and platelets (nonfunctioning) | 28 days | 1–6°C | Increase RBC mass in symptomatic anemia |
RBCs (additive, ~300 ml) | RBCs (~60% Hct); 40 ml plasma, 10 9 –10 10 WBCs and platelets (nonfunctioning); 100 ml additive solution | 35–42 days | 1–6°C | Increase RBC mass in symptomatic anemia |
RBCs, leukocytes reduced (~300 ml with additive; ~225 ml without additive | RBCs with Hcts as above; <5×10 6 WBCs (US) <1×10 6 WBCs (Europe), few dysfunctional platelets, 40 ml plasma; should contain ≥85% of original red cell content | 35–42 days | 1–6°C | Increase RBC mass in symptomatic anemia, decrease likelihood of febrile reaction, alloimmunization to leukocyte antigens, reduce cytomegalovirus (CMV) transmission |
Washed RBCs (~180 ml) | RBCs with ~Hct 75%; <5×10 6 WBCs, no plasma | 24 h | 1–6°C | Increased RBC mass in symptomatic anemia; reduced potassium, additives and pathologic antibodies |
Whole blood (~500 ml) | RBCs (~Hct 40%); 200 ml plasma, (deficient in labile clotting factors V and VIII, platelets (dysfunctional at 72 h); 10 9 –10 10 WBCs | 28 days | 1–6°C | Pump/other mechanical prime; hemolytic disease of the fetus and newborn, assists in hemostasis, if given 48 h or less after collection |
Whole-blood-derived platelets (~50 ml) | Platelets >5.5×10 10 /unit, ~50 ml plasma; RBCs; WBCs | 5 days | Agitated, 20–24°C | Bleeding caused by thrombocytopenia or thrombocytopathy |
Apheresis platelets (~300 ml) | Platelets (>3×10 11 /unit); ~300 ml plasma; RBCs; WBCs | 5 days | Agitated, 20–24°C | As above for whole-blood-derived platelets |
Platelets, leukocytes reduced (~300 ml) | Platelets as above; <5×10 6 WBCs | 5 days | Agitated, 20–24°C | As above for whole-blood-derived platelets; decrease in febrile reaction, alloimmunization to leukocyte antigens and reduce CMV transmission |
Fresh frozen plasma (FFP) (~250 ml) | Plasma, all coagulation factors and complement (no platelets) | 1 year | ≤−18°C | Treatment of coagulation factor deficiency when a suitable recombinant or derivative product is neither available or suitable; treatment for thrombotic thrombocytopenic purpura (TTP) |
PF24 (plasma frozen within 24 h after phlebotomy, ~250 ml) | Plasma, all stable coagulation factors and complement (no platelets), decreased level of labile coagulation factors | 1 year | ≤−18°C | Clinically significant coagulation factor deficiencies; treatment for TTP. Note: not to be used for deficiencies of labile clotting factors such as factor V and VIII; TTP |
PF24RT24 (plasma frozen within 24 h after phlebotomy, held at room temperature up to 24 h after phlebotomy, ~250 ml) | Plasma, all stable coagulation factors and complement (no platelets), decreased level of labile coagulation factors | 1 year | ≤−18°C | Clinically significant coagulation factor deficiencies; note: not to be used for deficiencies of labile clotting factors such as factors V and VIII and protein S; TTP |
Thawed plasma (derived from FFP, PF24, or PF24RT24, ~250 ml) | Plasma, all stable coagulation factors and complement (no platelets), variable level of other coagulation factors | See next entry | Thawed at 30–37°C and maintained at 1–6°C for 4 days postinitial 24-h period | Clinically significant coagulation factor deficiencies; note: not to be used for deficiencies of labile clotting factors such as factors V and VIII (and protein S, if prepared from PF24RT24); TTP |
Cryoprecipitate (~15 ml) | Fibrinogen ≥150 mg/unit; factor VIII ≥80 IU/unit; factor XIII, fibronectin, von Willebrand factor | 1 year | ≤−18°C | Deficiency or dysfunction of fibrinogen or factor XIII, von Willebrand disease, or hemophilia A when a recombinant product or derivative is not available |
Apheresis granulocytes (~200–300 ml) | Granulocytes (>1×10 10 PMN/unit); lymphocytes and monocytes; platelets (>2.0×10 11 /unit), some RBCs, 3–8×greater content when G-CSF and dexamethasone-stimulated donors) are used. | 24 h | Agitated 20–24°C | Provide functional granulocytes for febrile, septic patients, with anticipated WBC recovery and unresponsive to antibiotics/antifungals |
Irradiation
Irradiation prevents proliferation of donor lymphocytes which may recognize recipient tissues as foreign and initiate transfusion-acquired graft-versus-host disease ( Table 36.5 ). Irradiation practice varies among institutions. Some institutions selectively irradiate blood products on the basis of diagnosis, age (or weight) of the patient, or the relationship of the donor to the recipient, while other institutions irradiate all products. Much of the practice variability occurs because of logistical issues such as location of the irradiator, real or estimated wastage of the RBC products and, in particular, technologist availability and patient population. Irradiation of blood at the point of distribution in the blood bank is a reliable method for ensuring that inadvertently nonirradiated units are not administered. FFP and cryoprecipitate are derived from thawed frozen plasma which damages WBCs during the freezing process and do not require irradiation unless fresh unfrozen plasma is being used. Irradiation reduces RBC storage shelf life to not >28 days postirradiation depending on the time of irradiation relative to collection. Irradiation followed by refrigerator storage causes a higher concentration of potassium in the product. To avoid wastage of RBC units, irradiation prior to issue is optimal. Instrumentation used for blood irradiation includes:
- 1.
Free-standing cesium or cobalt gamma source irradiators ( 137 Cs or 60 Co).
- 2.
Linear accelerators and other devices which use X-ray sources.
FETUS/INFANT |
Intrauterine transfusion |
Premature infants |
Congenital immunodeficiency (identified or suspected) |
Those undergoing exchange transfusion for erythroblastosis |
CHILD/ADULT |
Congenital immunodeficiency (identified or suspected) |
Hematological malignancy or solid tumor (neuroblastoma, sarcoma, Hodgkin’s disease receiving ablative chemo/radiotherapy) |
Recipient of peripheral blood stem cells, marrow, cord blood, or cytotoxic T lymphocytes (CTL) |
Recipient of familial blood donation |
Recipient of HLA matched products |
Lupus or any other condition requiring fludarabine, cyclophosphamide or combination myeloablative therapy |
POTENTIAL INDICATIONS |
Term infant |
Recipients of solid organ transplants |
Recipient and donor pair from a genetically homogeneous population |
Other patients with hematological malignancy or solid tumor receiving immunosuppressive agents |
In the United States, the majority of facilities use free-standing lead shielded 137 Cs gamma irradiators which allow irradiation within minutes and have substantive controls to ensure that the bag or bags of blood products receive adequate dosing.
Washed Cellular Components
Washing of RBCs and platelets is used to reduce plasma proteins and cytokines and, in the case of RBCs, to reduce potassium, cytokines, and other by-products of RBC storage. Indications for washed products include:
- 1.
Severe or recurrent allergic reactions to plasma proteins in cellular blood products.
- 2.
Anaphylactic reactions due to IgA antibodies in IgA-deficient recipients.
- 3.
Infants with neonatal alloimmune thrombocytopenia (NAIT) who receive platelets from their mother where washing removes the circulating platelet-specific antibodies responsible for NAIT.
In recurrent, severe allergic reactions, RBC additive units (which contain almost no plasma) and premedication with one or more antihistamines may be considered in lieu of washing. Potassium should be removed from large volume (>20–25 ml/kg) RBC transfusions in at-risk infants or children. In patients with T-cell activation due to the exposure of the cryptic T-antigen by neuraminidase from bacterial infection, IgM T antibody, which is present in all adult plasma, can be removed by washing. Washing has also been used to remove the small amount of anti-A and anti-B in donor blood to be transfused to non-O patients with paroxysmal nocturnal hemoglobinuria and rarely for incompatible blood group organ and HSCT.
Washed cellular products expire in 24 h for RBCs and in 4 h for platelets. Coordination is required between the ordering physician and the blood bank when a washed product is needed. Washing causes a significant loss of platelets during processing and the platelets may become activated with decreased platelet aggregation and potentially worse in vivo survival and function.
Volume Reduction
The following are indications for volume reduction procedures designed to reduce the plasma volume in a product:
- 1.
Patients who have substantive renal or cardiorespiratory dysfunction (e.g., RBC and platelet transfusions in patients with renal failure).
- 2.
Patients who would benefit from removal of potentially pathologic antibodies in plasma (e.g., ABO-incompatible platelets).
In the case of ABO-incompatible platelets, some institutions measure isoagglutinin titers and establish specific allowable cutoffs instead of washing or volume-reducing platelet products. Volume reduction is associated with significant loss of product and increased activation postprocessing and should be used only in situations with well-defined indications. Small-volume storage of platelets in syringes for administration in neonates by syringe pumps has been recently studied.
Special Pediatric Population Needs
Neonates
Neonates requiring transfusion utilize multiple small volumes of blood products issued in syringes or transfer bags. Small-volume transfusions (≤15 ml/kg) of additive or nonadditive RBC units are the primary product used. Such RBC products may be used up to the date of expiration without the risk of hyperkalemia. However, infants requiring exchange transfusion, extracorporeal support for cardiovascular surgery, and ECMO are transfused at rates >25 ml/kg and often require multiple transfusions. In emergent circumstances of unexpected massive bleeding, when fresh (<7–10 days old), washed, or volume-reduced RBC units are not available, the infusion rate should not exceed 0.5 ml/kg/min.
Given the short shelf life of an RBC unit once accessed and the small volumes of blood used to transfuse neonates, blood banks will access the same unit to provide RBC transfusions to multiple neonates. Additionally, the blood bank will access the same unit for multiple transfusions in the same neonate if there are short intervals between the transfusions. These strategies reduce both RBC wastage as well as exposure to multiple blood donors.
Neonates with hemolytic disease who require exchange transfusion postdelivery or following intrauterine fetal transfusion may require special blood products. Blood grouping of these infants can be confounded by intrauterine transfusion, Rhogam administration to the mother and IVIG administration to the infant at delivery (see Chapter 5 , Chapter 9 ). Selection and preparation of reconstituted RBCs for exchange is provided in Table 36.6 .
Blood product | Citrate-phosphate-dextrose solution or citrate-phosphate-dextrose-adenine anticoagulant (CPDA-1) red blood cells (RBCs) |
Additive solutions can be used if they are hand packed or washed to remove additive solution | |
Hematocrit | 45–60% depending on desired end result |
Age | Should be as fresh as possible, <7 days |
Irradiation | Required to prevent transfusion-associated graft-versus-host disease (TA-GVHD) and performed as close to the transfusion as possible (<24 h) |
Sickle test | Negative |
With severe hemolytic disease of the fetus and newborn | O Rh (D) negative RBCs crossmatched against mother’s plasma and negative for any other antibody besides anti-D prior to delivery |
Donor blood prepared after infant’s birth should be antigen-negative and crossmatched-negative against infant’s specimen | |
For ABO HDN | RBCs must be type O and either Rh (D) negative or Rh (D) compatible with mother and infant. The blood should be washed free of plasma or have low titer anti-A or anti-B antibodies. Reconstitution with AB plasma may result in two donor exposures |
For Rh HDN | RBCs should be Rh (D) negative and either Group O or same group as infant. Reconstitution with AB plasma may result in two donor exposures |
For polycythemia | Dilution with saline or albumin is recommended |
VOLUME OF BLOOD REQUIRED FOR EXCHANGE TRANSFUSION | |
Single volume exchange | Exchanges 60% of infant’s blood volume |
Double volume exchange | Exchanges 85% of infant’s blood volume |
Example: 2×infant’s blood volume=2×85=170 ml | |
Equations | Partial exchange for severe anemia: Volume (ml)=Infant’s blood volume×(Hb desired − Hb initial)/(pRBC Hb − Hb initial) Single volume or partial exchange for correction of polycythemia: Volume (ml)=Infant’s blood volume×Hct change/Initial Hct Final or Target Hb <SPAN role=presentation tabIndex=0 id=MathJax-Element-1-Frame class=MathJax style="POSITION: relative" data-mathml='FinalHb=%Exchanged×Hb(donor)+%Unexchanged×Hb(recipient)100′>FinalHb=%Exchanged×Hb(donor)+%Unexchanged×Hb(recipient)100FinalHb=%Exchanged×Hb(donor)+%Unexchanged×Hb(recipient)100 Final Hb = % Exchanged × Hb ( donor ) + % Unexchanged × Hb ( recipient ) 100 Note: % Exchanged=% effectively exchanged or target % exchanged % Unexchanged=100 − % Exchanged Hb(donor)=Hemoglobin of donor blood Hb(recipient)=Hemoglobin of recipient blood Volumes of RBCs and plasma needed for reconstitution: Example: Double volume exchange in an infant (85 ml/kg), desired Hct 0.45% in product. Total volume=infant weight/kg×2=85 ml/kg×2=170 ml Absolute volume of RBCs needed (ml)=Total volume×desired Hct (0.45)=170 ml×0.45=76.5 ml Assuming Hct (unit)=0.75 Actual volume of RBCs needed=Absolute volume/Hct (unit)=76.5 ml/0.75=100.8 ml Volume of FFP needed=Total volume − Actual volume of RBCs needed=170 ml − 100.8 ml=69.2 ml This will result in a double volume (170 ml) product at Hct of 0.45 needed for exchange |
Liberal transfusion criteria in the neonatal period may provide some protection from CNS hemorrhage, periventricular leukomalacia, and other known postnatal complications of prematurity. However, In terms of platelet transfusions, a multiinstitutional observational study found that infants with platelet counts <20,000/mm 3 were not at increased risk for major hemorrhage.
Patients on Extracorporeal Life Support and Other Critically Ill Patients
There has been a controversy over whether there is an increase in morbidity and mortality associated with the use of old versus fresh RBCs. Theoretically, older RBC products suffer from the RBC storage lesion, which entails biochemical changes that occur to stored RBCs that have potential clinical consequences ( Table 36.7 ). Studies of oncology patients, patients undergoing cardiac surgery, and trauma patients have shown an increase in morbidity and mortality when “older” RBCs are transfused. Recently, the Age of Red Blood Cells in Premature Infants study, a double-blind, randomized clinical trial of transfusion of RBCs <7 days old versus standard-age RBCs in very low birth weight demonstrated no difference in clinical outcomes between the two groups.
Biochemical and biochemical changes | In vitro findings | In vivo correlations with stored RBCs/potential clinical significance |
---|---|---|
2,3-diphosphoglycerate (2,3-DPG) depletion | Left shift of oxygen–hemoglobin (Hb) curve | Restoration of RBC 2,3-DPG within 24–72 h of transfusions; delay in peripheral oxygen offloading |
adenosine triphosphate (ATP) depletion | Reversible red blood cell (RBC) spheroechinocyte formation | Poor correlation of ATP levels with 24 h posttransfusion RBC survival. Splenic trapping |
Increased intracellular calcium | RBC dehydration with echinocytosis and microvesiculation | Increased viscosity, reduced flow in capillary systems, microparticles |
Metabolic modulation | Increased glucose consumption via pentose phosphate pathway and increased O 2 saturation of Hb; progressive acidification and lactate accumulation | Metabolic abnormalities in recipient |
Decreased Na–K pump activity | Leakage of K from RBCs not returned to RBCs | Increased K levels in older RBCs, clinically significant hyperkalemia; worsened by RBC irradiation and refrigerator storage |
Depletion of SNO-Hb | Loss of SNO-Hb after 3 days of storage | No off-loading of NO under hypoxic conditions; less vasodilation and blood flow in capillary systems |
Low-level hemolysis | Free Hb scavenges nitric oxide (NO); break down to free heme and iron | Decreased NO causes vasoconstriction; free heme causes pulmonary hypertension and acute tubular injury in the kidney |
Membrane phospholipid loss | Deduction of RBC microvesicles correlates with spheroechinocyte formation and increased osmotic fragility | Less vesiculation associated with prolongative of storage time and increased survival of AS-1 preserved RBCs |
Abnormal membrane phospholipid distribution | Phosphatidyl-serine (PS) accumulates on outer membrane in acid pH or in presence of aminophopholipid translocase inhibitor. This loss is seen less with additive solutions and hypotonic solutions. However, no change during routine blood bank storage |
|
Lipid peroxidation and protein oxidation |
|
|
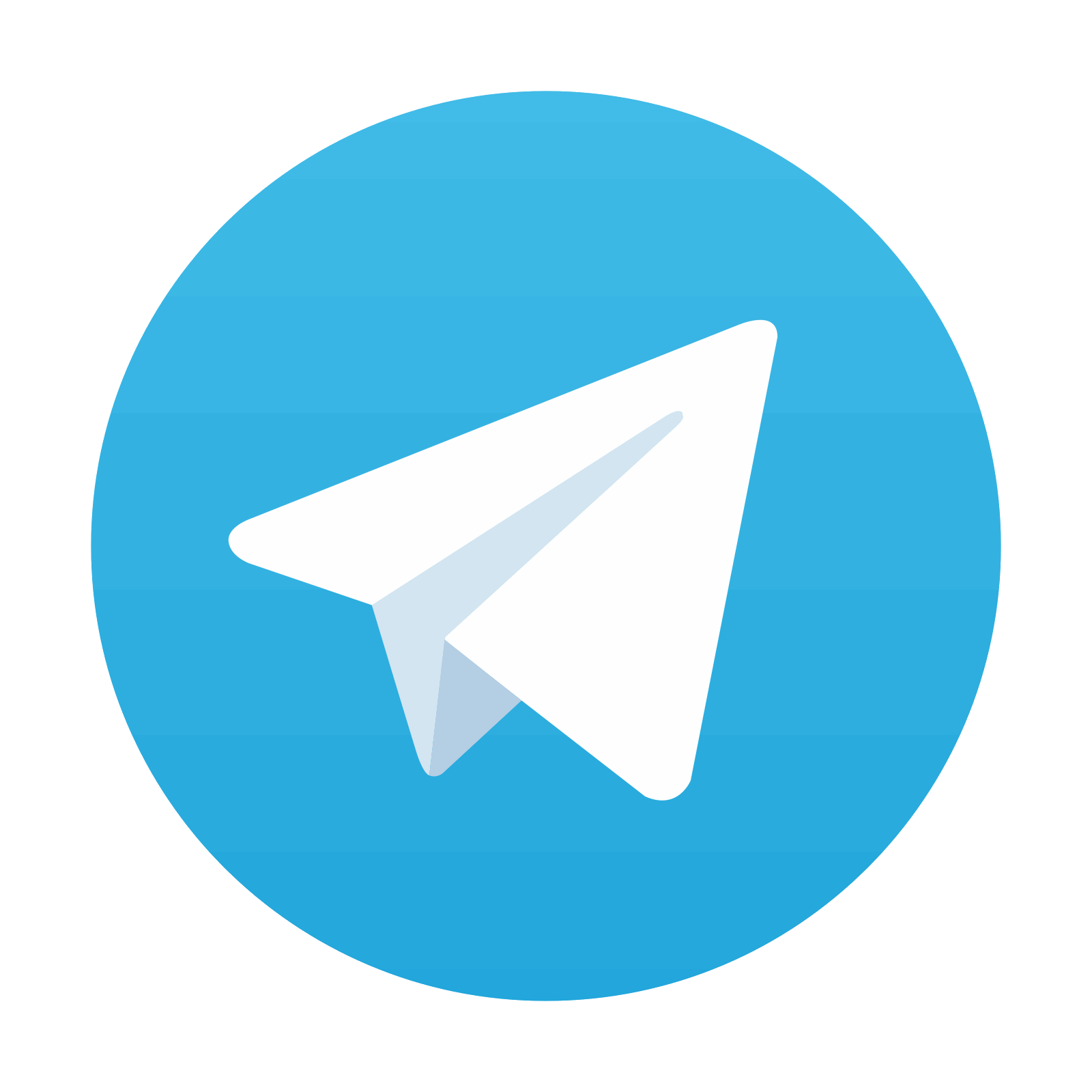
Stay updated, free articles. Join our Telegram channel

Full access? Get Clinical Tree
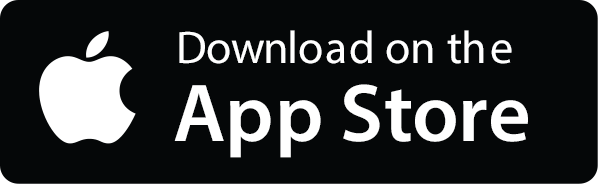
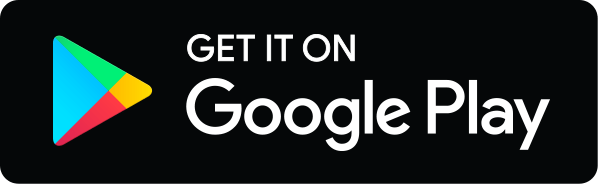
