16.1
Introduction
Age-related fractures represent an immense and increasing public health issue. In the United States in 2005, there were an estimated 2 million age-related fractures, with associated medical expenditures of $17 billion, and fracture incidence and costs are expected to increase 50% by 2025 . Fractures of the hip, wrist, and vertebrae account for the majority of age-related fractures, with vertebral fractures being the most prevalent, and hip fractures having the most severe consequences. The consequences of age-related fractures are enormous, as those who suffer fractures experience increased mortality rates, chronic pain and disability, and a decreased quality of life .
Strategies designed to prevent fractures must be based on a sound understanding of their etiology. From an engineering viewpoint, fractures of any type are due to a structural failure of the bone. This failure occurs when the forces applied to the bone exceed its load-bearing capacity. The load-bearing capacity of a bone depends on the interaction of several factors, including the bone tissue (material behavior), geometric characteristics (size, shape, and distribution of bone mass), and the specific loading conditions experienced (loading direction, loading rate) ( Fig. 16.1 ). This suggests that in addition to material and structure (see Chapter 13 : The mechanical behavior of bone, for a more detailed discussion of these), loading is also an important determinant of fracture risk. In support of this concept, clinical studies have repeatedly shown that factors related to the loads applied to the skeleton are important determinants of fracture risk .
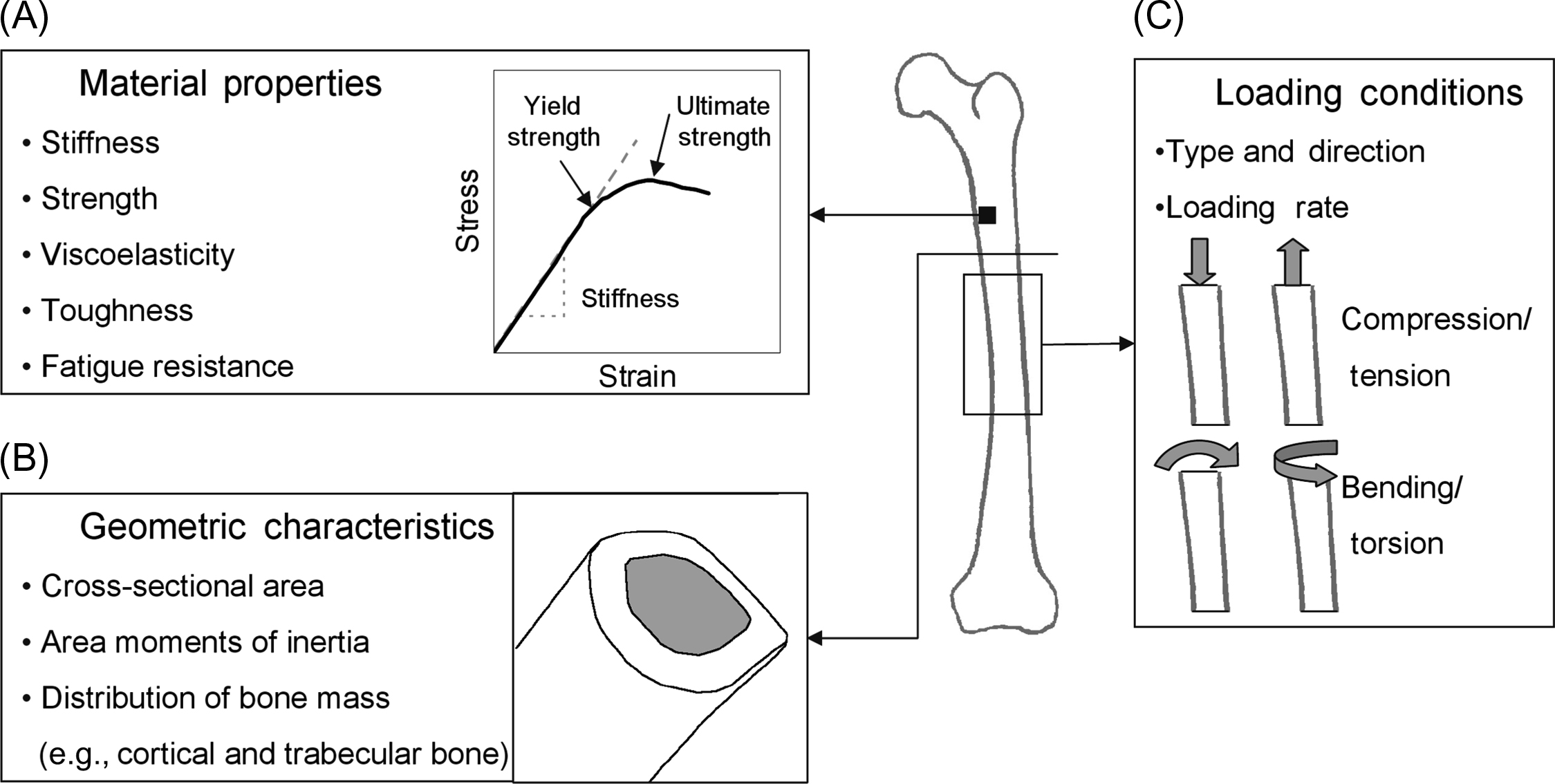
Insight into the relative contributions of skeletal fragility versus skeletal loading may be gained by using a standard engineering approach for evaluating the risk of structural failure. To design a structure, engineers consider the size and geometry of the structure, the materials from which it is to be made, and the types of loads to which it will be subjected. Using this information, the loads applied to the structure during its normal usage can be compared to the loads known to cause failure. This comparison of applied load versus failure load gives an estimate of how safely the structure is designed. If a structure’s design appears unsafe, it is necessary to change the geometry of the structure (e.g., increase its size), use stronger materials, or reduce the applied loads. In practice, it is often difficult to precisely estimate the strength of a structure and the loads applied to it. Therefore, to reduce the likelihood of unexpected failure, structures are often designed with high safety factors so that strength far exceeds the maximum expected loads.
To apply these concepts in the study of the etiology of fractures, one can derive the load-to-strength ratio, namely, the ratio of the load delivered to a bone (or applied load ) to the load-bearing capacity of that bone (failure load) for a given activity ( Fig. 16.2 ). Thus, when the load-to-strength ratio is much less than one, the forces applied to the bone are much lower than those required to fracture it, and the bone is at low risk for fracture. However, when the load-to-strength ratio is high (≫1), fracture of the bone is predicted to occur. A high load-to-strength ratio can occur either when the bone is very weak and its load-bearing capacity is compromised, or when very high loads, such as those resulting from trauma, are applied to the bone. In elderly individuals, it is likely that the coupling of a weak bone with an increased incidence of traumatic loading leads to the dramatic rise in fracture incidence with age.
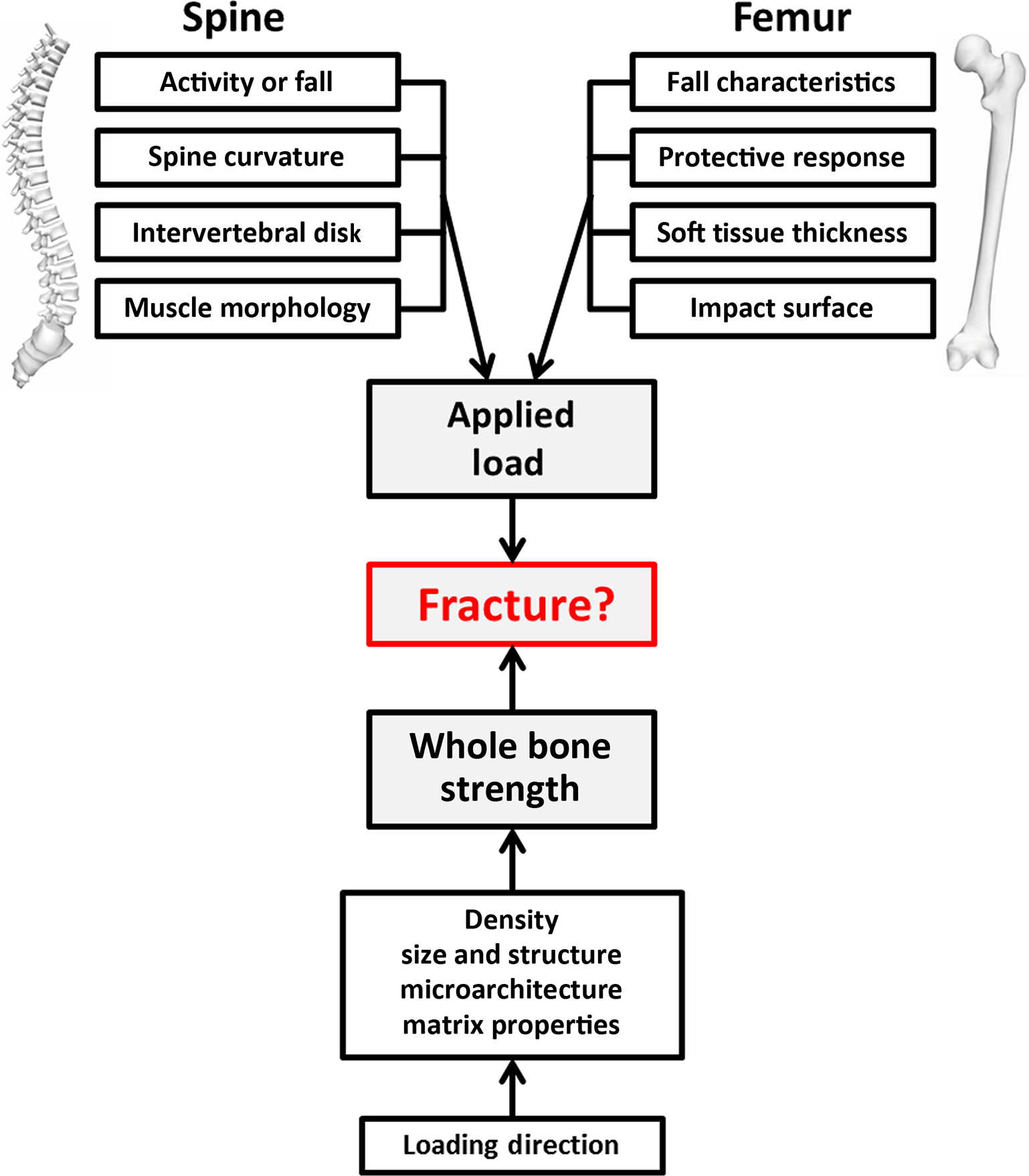
To apply the concept of load-to-strength ratio in studies of hip and vertebral fracture, the everyday loads applied to the bone of interest and the corresponding load required to fracture the bone must be identified. For example, the majority of hip fractures are associated with a fall. Therefore information about the loads applied to the femur during a fall and about the load-bearing capacity of the femur in a fall configuration are required. While this approach is relatively easy to conceive, in practice it is difficult to apply. There are surprisingly few data detailing the magnitude and direction of loads applied to the skeleton during activities of daily living or engendered during traumatic events, such as a trip, slip, or fall. Moreover, due to the complex morphology of the skeleton and associated muscle and tendon attachments, it is difficult to design a laboratory study that mimics the loading environment encountered by the bone in vivo. Therefore it is challenging to determine the load-bearing capacity of skeletal elements under realistic loading conditions. Moreover, both the applied loads and whole bone strength change with aging, pharmacologic intervention and disease. Nevertheless, despite these uncertainties and limitations, recent advances in fall surveillance, multibody musculoskeletal modeling (to derive in vivo loading conditions) and rough estimates of the load-to-strength ratio have now been derived for hip and vertebral fractures, providing insights into the complex roles of loading severity and skeletal fragility in the etiology of age-related fractures.
In this chapter, clinical and laboratory studies related to the biomechanics of hip and vertebral fractures are reviewed, including the factors that are related to the loads applied to the skeleton, either through traumatic events or everyday activities; the factors that are related to the structural capacity of skeletal elements; and how these factors interact to influence fracture risk.
16.2
Biomechanics of hip fractures
Hip fractures are common and costly injuries in older adults with devastating consequences for health and well-being . The age-adjusted risk of mortality in the year following a hip fracture is more than double in women and is nearly three times greater in men, relative to controls without fracture . Furthermore, the majority of hip fracture survivors do not regain their prefracture level of functionality in activities of daily living, with many losing their ability to walk unassisted . The incidence of hip fractures increases dramatically with age , and with the growth of the elderly population, the total number of hip fractures worldwide is likely to rise two- to fourfold in the next several decades, with particularly high increases in Asia .
To study the etiology of hip fractures from a biomechanics perspective, one must first identify what event(s) are associated with hip fractures and then determine the loads that are applied to the bone and the load-bearing capacity of the femur during that event. In the case of hip fractures the event of primary interest is relatively clear, as it is estimated that over 90% of hip fractures in the elderly are associated with a fall . Thus studies of the etiology of hip fractures are complicated by the need to examine risk factors for falls, as well as risk factors for fracture. In addition, given that fewer than 2% of falls in the elderly result in a hip fracture , investigations of hip fracture etiology must also distinguish factors related to “high-risk” falls that result in fracture. Therefore in this section, we will review clinical and laboratory studies related to the factors influencing the loads applied to the femur during a fall and the load-bearing capacity of the femur in a fall configuration.
16.2.1
Falls and hip fractures
A fall can be defined as a sudden, unexpected event that results in a person coming to rest on a surface lower than their starting position. A fall can be further characterized by several phases: (1) an instability phase resulting in fall initiation, (2) a descent phase, (3) an impact phase, and (4) a postimpact phase during which the faller comes to rest. The definition of “fall severity” is more difficult. From a biomechanical perspective, fall severity can be described by the magnitude and direction of the load applied to the hip, and the impact site . From a clinical perspective, Cummings and Nevitt suggested that a high-risk fall includes (1) impact on or near the hip, (2) lack of active protective mechanisms such as an outstretched arm to break the fall, and (3) insufficient energy absorption by local soft tissues. Thus by these criteria a high-risk fall could transmit a force to the proximal femur that exceeds the force required to fracture the hip.
16.2.1.1
Epidemiologic studies of falls and hip fracture
A number of epidemiologic studies have been conducted to examine the circumstances and the characteristics of falls that result in hip fractures . The individual’s environment, activity, as well as sociodemographic and health factors all contribute to falls and hip fracture . For example, a community-based study of older adults recorded 1172 falls and reported that most falls occur within the home, with injurious falls more likely to occur in those older than 85 years, those who fell in the bathroom, and those who fell sideways or backward .
Not surprisingly, fall impact at or near the hip is a very strong risk factor for hip fracture . Likely because of this, the direction of a fall strongly influences the risk of hip fracture. A study of 612 elderly fallers found that in both men and women, falling backward, straight down, or sideways increased the risk of hip fracture about 10- to 15-fold relative to falling forward . Multiple case–control studies of fallers with hip fractures and without hip fractures have found that falling to the side is a strong risk factor for hip fracture independent of hip bone mineral density (BMD), increasing fracture risk about 2.5- to 5.7-fold . These studies show that in elderly fallers, independent risk factors for hip fracture include characteristics related to fall severity, low BMD at the hip, body habitus [e.g., low body mass index (BMI)], and factors related to mobility and balance ( Table 16.1 ). Importantly, all these studies indicate that specific types of falls are more “severe” in terms of hip fracture risk, regardless of bone strength, and that falling to the side and impacting near the hip represents a particularly risky event.
Factor | Greenspan et al. , N =149 | Greenspan et al. , N =132 | Wei et al. , N =197 |
---|---|---|---|
Fall to the side | 5.7 (2.3–14) | 4.7 (1.7–13) | 2.5 (1.6–3.9) |
Impact at the hip | − | − | 4.9 (2.7–8.8) |
Potential energy of fall (J) a | 2.8 (1.5–5.2) | − | − |
Femoral neck BMD (g/cm 2 ) b | 2.7 (1.6–4.6) | 1.9 (1.1–3.2) | 1.7 (1.0–2.8) |
Body mass index (kg/m 2 ) b | 2.2 (1.2–3.8) | − | 1.8 (1.1–2.8) |
Mobility c | − | 3.5 (1.1–11) | 2.0 (1.1–3.5) |
Previous stroke | − | − | 2.9 (1.3–6.3) |
a Calculated for an increase of 1 SD.
b Calculated for a decrease of 1 SD.
c Substantial assistance or dependent ; calculated for a decrease of 1 SD .
16.2.1.2
Real-world fall surveillance studies
As noted previously, fall characteristics play a critical role in hip fractures. Yet, until recently the majority of information on fall-related risk factors for hip fracture were derived from controlled laboratory studies of young adults or from subjective self-reports in community-dwelling older adults. To address this limitation, investigators have begun to report fall circumstances and characteristics based on video-captured “real-world” falls in older adults . In the first study of this kind, Robinovitch et al. described the circumstances of 227 falls in 130 individuals in a long-term care facility, noting that the most frequent causes of falls were incorrect weight shifting (41% of falls) and a trip or stumble (21% of falls), with few falls associated with slipping (3%) . They extended this initial work to identify the risk factors for hip impact during real-world falls, analyzing >500 falls in 160 adults . Notably, hip impact occurred in 40% of falls, with increased risk of hip impact for forward and sideways falls. Many of those who fell sideways were able to rotate their trunk to land on their buttocks; however, individuals exhibiting difficulty with activities of daily living were less able to utilize this protective response. Analyses of real-world falls have also identified discrepancies with fall incident reports , variations in fall circumstances by sex , characteristics of frequent versus infrequent fallers , and differences in fall kinematics when compared to laboratory-based studies . In sum, this relatively new line of research in the analysis of real-life falls in older adults brings novel insights for interventions designed to reduce the risk of falls, and in particular, injurious falls, among older adults.
16.2.2
Factors influencing the loads applied to the femur during a fall
16.2.2.1
Active protective responses
Due to the clear importance of sideways falls in hip fracture etiology, several laboratory investigations have been conducted to further study the characteristics of sideways falls in relation to hip impact forces, including protective measures that might be taken to reduce impact forces. In a study of the descent phase of sideways falls from standing height in young adults, van den Kroonenberg et al. found that approximately 70% of the total energy available is dissipated during the descent phase of a sideways fall from standing height. This energy dissipation is likely due to muscle activity and the stiffness and damping characteristics of the hip and knee joints. “Active responses,” such as using the arm to break a fall, reduce the impact forces experienced at the hip during falls to the side . In a study that elicited sideways falls in 42 young adults ( Fig. 16.3 ), more than 90% of falling subjects impacted the hand and the hip region . Impact on the hand preceded impact on the hip by an average of 50 ms, which decreased hip impact velocity by about 18%. Thus, while young adults may utilize protective responses to reduce hip impact, it does not appear that they prevent impact on the hip during sideways falls. However, use of the arm alone to break a fall is probably not the ideal “active response,” as it may provide insufficient protection as well as place the faller at risk for wrist fractures. A study of younger and older women estimated that the energy of a fall is about 2.7 times greater than the energy that young women could absorb with both arms, and about 5 times greater than the energy that older women could absorb . In a sideways fall the energy absorption potential is likely much less, as only one arm could be realistically used to break the fall. Furthermore, the average time required for an older woman to position her hand to catch herself is longer than for a young woman, such that an older woman may not be able to use her hand to help break a sideways fall . Indeed, in simulated falls onto outstretched hands, older women have lower capacity to absorb energy and to control their descent compared to younger women . Thus, despite the potential for reducing fall severity via active responses, it is likely that with age, the ability to dissipate energy during a fall or to activate protective responses will decrease, and therefore it is likely that elderly individuals “fall harder” than young adults.

With the difficulties inherent in conducting experimental studies of falls and fall impact that are simultaneously safe and realistic, additional insights may be gained through the use of biomechanical modeling. In such a study, Lo and Ashton-Miller used a forward dynamic biomechanical model to simulate sideways falls and examine the effects of different falling strategies on impact forces and risks of injury. A fall to the side from standing height with the hip as the primary impact site estimated hip impact force as 5574 N, which would likely cause a hip fracture in older adults as this well exceeds the average femoral strength of older adult cadaveric specimens tested in vitro . However, by using knee flexion, waist flexion, and axial trunk rotation during the fall to minimize injury risk, the hip impact force was reduced to 1585 N, a 72% reduction. This is consistent with the experimental finding that about 70% of fall energy is dissipated in the descent phase . Using the hand to help break the fall decreased the hip impact force to 1225 N, a further reduction of 23%, also comparable to experimental results . It was also found that impact forces increase with reductions in available joint torques (simulating reduced muscle strength in older adults) and longer delays before initiating the falling strategy (simulating slower reaction times). Thus hip impact forces varied in magnitude by as much as fivefold depending on the falling strategy adopted, and this study shows that adopting the “right” falling strategy can greatly reduce impact forces.
If there is a “right” way to fall, perhaps learning or practicing it could be a useful strategy in reducing hip impact forces and preventing hip fractures. This concept has been explored in a series of small experimental studies of martial arts falling techniques. By using proper falling techniques, experienced martial artists can reduce impact forces in sideways falls from a kneeling position by about 27%–30% versus simply falling on the hip and using the hand to arrest the fall . With brief periods of training, inexperienced young adults were able to use these techniques to reduce hip impact forces by 17% , whereas inexperienced older adults were able to reduce hip impact forces by 8% . A limitation of these studies is the use of falls from kneeling height for safety, which alters the direction and location of hip impact force in experienced martial artists , with uncertain effects on fall “severity.” Further research is needed to better understand the potential of training people in falling techniques to reduce femoral loading in “real-world” falls and to prevent hip fractures in older adults.
16.2.2.2
Passive approaches to reduce the loads applied to the femur: trochanteric soft tissue
The forces applied to the proximal femur during a sideways fall depend not only on the dynamics of the descent phase but also on characteristics of the impact phase of the fall. During a sideways fall with impact to the greater trochanter, about 15% of the total impact force is distributed to structures peripheral to the hip, with the remainder of the force delivered along a load path directly in line with the hip . However, the peak force experienced by the femur is attenuated by the soft tissues overlying the greater trochanter. In a study using trochanteric soft tissue samples obtained from nine cadavers, positioned over a surrogate proximal femur and pelvis, and subjected to a typical impact load associated with a sideways fall , increased soft tissue thickness was strongly correlated with reduced peak femoral impact force and increased soft tissue energy absorption. Importantly, however, the energy-absorbing properties of trochanteric soft tissue decline substantially with age and likely contribute to the increased risk of hip fracture among older adults.
16.2.2.3
Passive approaches to reduce the loads applied to the femur: hip protectors
If trochanteric soft tissue can attenuate impact forces applied to the femur, artificial padding may provide an intervention to reduce hip impact forces and prevent hip fractures. Thus a variety of studies have examined various types of hip-protecting pads under laboratory conditions. An in vitro study found that force attenuation provided by hip protectors depends strongly on impact velocity, pelvic size, and pelvic stiffness . Generally, protectors with lower stiffness reduced impact force better than stiffer pads, the ability to absorb energy is important at higher impact velocities, and designs that bridge the greater trochanter rather than contacting it were better at lower impact velocities. In a laboratory comparison of 26 types of commercially available hip protectors, the impact force in a simulated sideways fall was reduced by 2.5%–40% . Recent studies have also tested novel approaches to hip protector design, including improved pad materials, three-dimensional (3D) printed hip pads, the concept of a “wearable airbag,” and development of a “stick on” hip pad . Established guidance for laboratory testing of hip protectors should be followed for any newly developed padding systems prior to testing in clinical trials . In addition to in vitro studies, two in vivo studies found that in low magnitude in vivo impacts on the greater trochanter hip protectors reduced peak pressure at the greater trochanter by about 70% compared to no pads , with the effect of hip protectors being twice as large in women with low versus high body mass . Thus there is significant evidence that hip protectors can reduce femoral loading during a fall. However, proper usage is important, as the protective effects will be largely negated if a hip protector is positioned as little as 5 cm from its intended position .
Despite the strong experimental rationale behind the use of hip protectors, their effectiveness as a clinical intervention has been mixed . Some studies have reported that hip pads reduce the risk of hip fracture in older adults, while others have not found a significant protective effect of hip pads. A review and metaanalysis of clinical trials found that hip pads are not effective in preventing hip fractures in community-dwelling older adults, and there is only marginal evidence that hip pads are effective in preventing hip fractures in older adults in nursing home residents.
Unfortunately, acceptance and proper use of the hip protectors is relatively poor in most trials, indicating that steps to improve pad design for comfort and ease of use must be taken if hip pads are to be a useful tool in preventing hip fractures . For example, Korall et al. reviewed the use of hip protectors in a study conducted in several long-term care facilities . They noted that hip protectors were only worn during 60% of the reported falls. Importantly, however, the risk of hip fracture was reduced by nearly threefold by wearing a hip protector at the time of falling. These findings support the need for research and/or educational efforts to maximize adherence with hip protectors in long-term care facilities.
16.2.2.4
Passive approaches to reduce the loads applied to the femur: energy-absorbing floors
A novel approach to reducing loads applied to the hip during a fall is via the use of compliant flooring, a passive intervention approach that acts as a shock absorber (i.e., reduces the ground stiffness) and, consequently, attenuates the applied forces during the impact phase of the fall. Developing an effective compliant flooring system is challenging since reducing the floor stiffness may negatively impact a person’s balance and increase fall risk. Epidemiologic and laboratory studies have shown the ability of compliant flooring to lower the impact forces during the fall. For example, Maki and Fernie tested 13 different floor coverings in a simulated fall study and reported that compliant flooring could decrease the peak impact forces by up to 23%.
In comparison with hip protectors, compliant flooring has couple of advantages. First, the hip protectors can only protect one specific impact location, however, the proper compliant flooring has the potential to reduce the risk of a range of fall-related injuries. Second, unlike the hip protectors, adherence would not be a concern for compliant flooring . Moreover, some studies have reported that the simultaneous use of compliant flooring systems and hip protectors provides higher attenuation in peak impact force during the fall .
Although, there is a lack of synthesized evidence regarding the key elements that affect the performance of compliant flooring systems, a scoping review by Lachance et al. examined biomechanical efficacy, clinical effectiveness, cost-effectiveness, and safety of compliant flooring with respect to prevention of fall-related injuries in older adults. Laboratory studies indicated that compliant flooring could reduce impact forces during a fall with minimal effects on balance during walking and standing. Clinical studies indicated that that although the compliant flooring can reduce fall-related injuries, the risk of falling is higher. Another study noted that the energy absorption in sideways falls was threefold greater for subjects with high BMI than for those with low BMI . In summary, while compliant flooring appears a promising approach for preventing fall-related injuries (including hip fractures), additional research is needed to confirm efficacy in reducing injuries, cost-effectiveness, and workplace safety.
16.2.3
Factors that influence the femoral strength
As discussed elsewhere in this volume (see Chapter 13 : The mechanical behavior of bone), multiple factors contribute to the load-bearing capacity, or fracture strength, of a bone, including its intrinsic material properties and the total amount (size) and spatial distribution (shape) of the bone tissue. Since the mechanical properties of both cortical and trabecular bone are strongly related to bone density, many have hypothesized that age-related bone loss is a primary contributor to the steep increase in hip fracture incidence with age. In support of this hypothesis, there is strong evidence from prospective clinical studies that low BMD, measured both at the hip and at other sites, is a risk factor for hip fracture . Furthermore, case–control studies of elderly fallers have reported that low BMD of the hip is a risk factor for hip fracture that is independent of fall characteristics .
16.2.3.1
Age, loading rate, and loading direction
Laboratory studies have evaluated the load-bearing capacity of the proximal femur and confirmed that areal BMD (aBMD) assessed using dual-energy X-ray absorptiometry (DXA) and volumetric BMD (vBMD) from quantitative computed tomography (QCT) are strongly correlated to the load-bearing capacity of human cadaveric femurs. Femoral bone mineral content and density explain around 40%–80% of the variation in load-bearing capacity of the proximal femur , and this relationship is fairly linear. Some studies have used a loading configuration designed to simulate the single-leg stance phase of gait . The loads required to fracture the femur in stance conditions ranged from approximately 1000 to 13,000 N (225–3000 lb). Other studies have evaluated the load-bearing capacity of the proximal femur in a configuration designed to simulate a sideways fall with impact to the greater trochanter . The loads required to fracture the femur in sideways fall impact conditions ranged from approximately 500 to 10,000 N (112–2250 lb). Importantly, a study in which 18 pairs of cadaveric femurs were tested, one side in stance configuration and the other in sideways fall configuration indicates that the loads required to fracture femurs in a sideways fall configuration are more than three times less than loads in a stance configuration ( Fig. 16.4 ). This highlights the importance of loading configuration for the load-bearing capacity of the femur, and the increased risk of hip fracture imposed by a sideways fall. Moreover, subtle differences in the direction of a sideways fall loading can greatly influence femoral strength, with as large an effect as 25 years of age-related bone loss . In addition to loading configuration, loading rate can also affect the load-bearing capacity of the femur due to the viscoelastic properties of bone. Courtney et al. studied the effect of age and loading rate on the failure load of the proximal femur in a fall configuration ( Fig. 16.4 ). When tested at a slow loading rate (2 mm/s), femurs from young individuals (age 17–51) were more than twice as strong as femurs from older individuals (age 59–83). At a high loading rate (100 mm/s), such as might be expected during a fall, femurs from both young and older individuals were approximately 20% stronger than at the slow loading rate. However, femurs from the younger group were still approximately twice as strong as those from the older group.
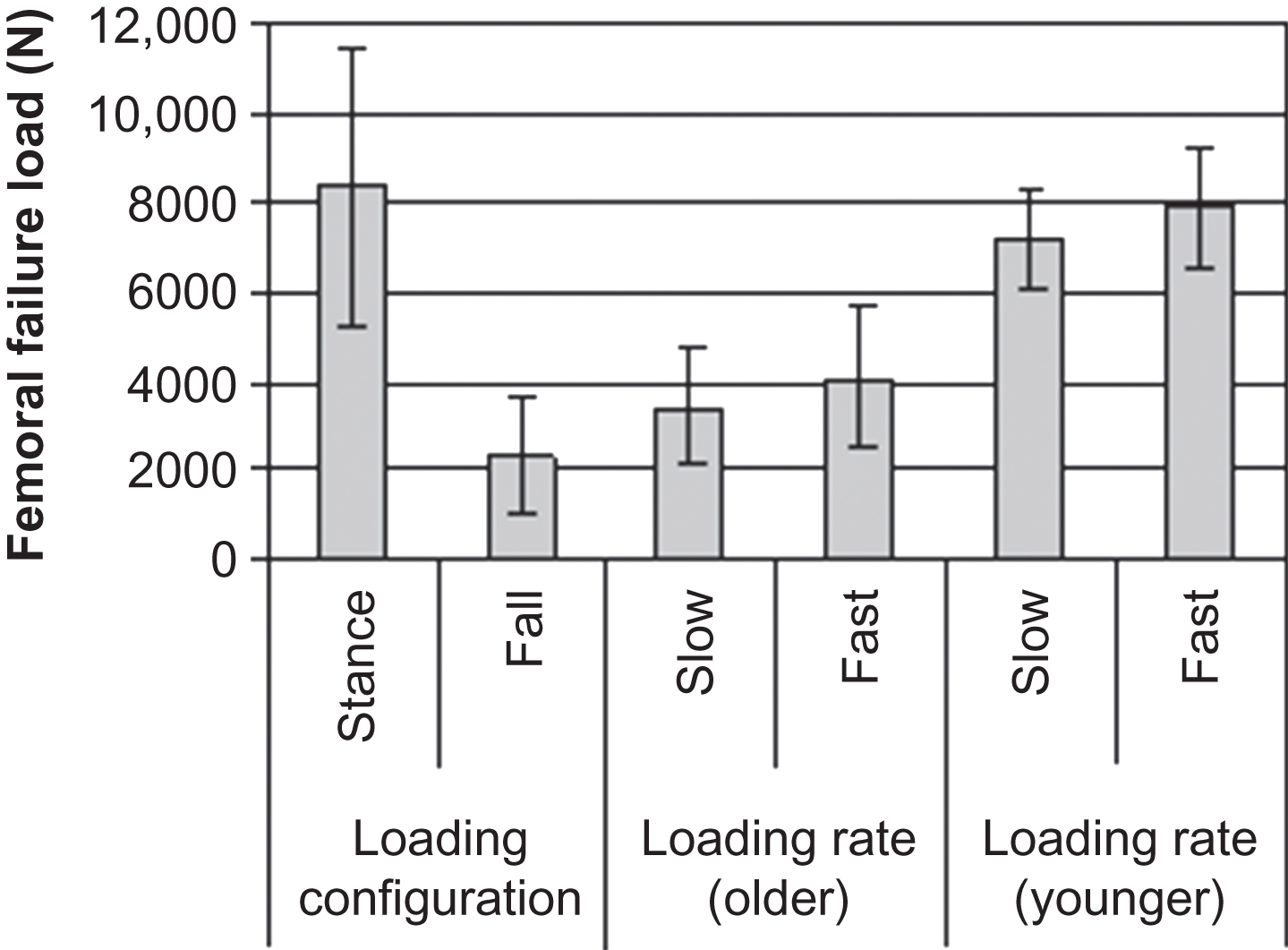
16.2.3.2
Bone density, size, and structure
In addition to BMD, loading direction, and loading rate, femoral geometry also influences the load-bearing capacity of the proximal femur. Since the load-bearing capacity is a structural property, it is influenced by the size of the specimen. Therefore larger femurs have a greater load-bearing capacity; and femoral neck area, neck width, and neck axis length are all positively correlated ( r 2 =0.21–0.79) with femoral failure loads . It is interesting to note that the positive correlation between femoral neck length and femoral strength appears to contradict findings from clinical studies, where a longer femoral neck length or hip axis length (HAL) is associated with a greater risk of hip fracture . Some evidence has suggested that the association between HAL and fracture risk comes from the inclusion of “acetabular thickness” in addition to femoral neck length in the HAL measurement . However, biomechanically, a longer femoral neck could result in a longer moment arm of force on the proximal femur during a fall impact. A recent case–control study found that moment arm in a sideways fall was about 8%–9% longer in cases than controls, which results in about a 20% larger moment applied to the hip during a fall . Thus the geometry of the proximal femur may play an important role in femoral loading, and additional studies are needed to better understand the complex relationship between hip geometry and fracture risk.
16.2.3.3
Noninvasive assessment of femoral strength
While in vitro laboratory studies are useful for understanding the factors that influence the load-bearing capacity of the femur, the ability to predict femoral strength in vivo is critical to clinical evaluation of fracture risk. Fortunately, DXA and QCT provide noninvasive methods by which information on BMD and geometry may be obtained. In a simplistic approach, femoral strength may be estimated from DXA-based BMD using published regressions . A more thorough, although more technically difficult, approach is 3D finite element analysis (FEA) based on QCT data, which can incorporate geometry and material distribution information within a single model. Multiple studies have found that measured fracture strength of cadaveric femurs is highly correlated with FEA predictions for stance loading configurations ( r 2 =0.75–0.96) and sideways fall loading configurations ( r 2 =0.85–0.90) . In general, FEA explains more of the variance in measured strength than densitometric measurements , although this increase has not always been statistically significant, likely due to small sample sizes. However, FEA offers other advantages, including the prediction of fracture site as well as strength and estimates of strength under a variety of loading configurations . Thus FEA estimates of bone strength appear useful as a technique for improved clinical evaluation of femoral strength and fracture risk .
In summary, the load-bearing capacity of the proximal femur is influenced, at least in part, by femoral BMD, femoral geometry, loading direction, and loading rate. At a given moment an individual’s bone density and geometry are constant, although they can readily change with age or therapeutic intervention. However, loading direction and loading rate are highly variable, and as such the characteristics of a fall significantly influence fracture risk. Overall, studies have demonstrated strong relationships between the load required to fracture the femur and noninvasive measurements of BMD or content and bone geometry from DXA or QCT. The use of techniques such as FEA that integrates geometry and material factors into a structural model of the femur may further improve estimates of femoral strength and fracture risk.
16.2.4
Interactions between fall severity and femoral strength: the load-to-strength ratio for hip fracture
The concept of a load-to-strength ratio for fracture risk assessment implies that low BMD is not the only indicator of risk, but rather, that the loads applied to the bone must also be considered. In order to apply the load-to-strength ratio to evaluate risk of hip fracture in patients, one must be able to estimate both the strength and loading of the proximal femur under relevant conditions. Based on the epidemiology, the most relevant condition for hip fracture in older adults appears to be a sideways fall with impact near the hip. Based on biomechanical experiments and models , an estimate of femoral impact forces associated with a sideways fall may be derived from an individual’s height and body mass. Specifically, this equation takes the form:
Peakimpactforce(N)=2ghmk
Peakimpactforce(N)=2ghmk−71×T
Femoralstrength(N)=7527×trochantericaBMD(g/cm2)–443
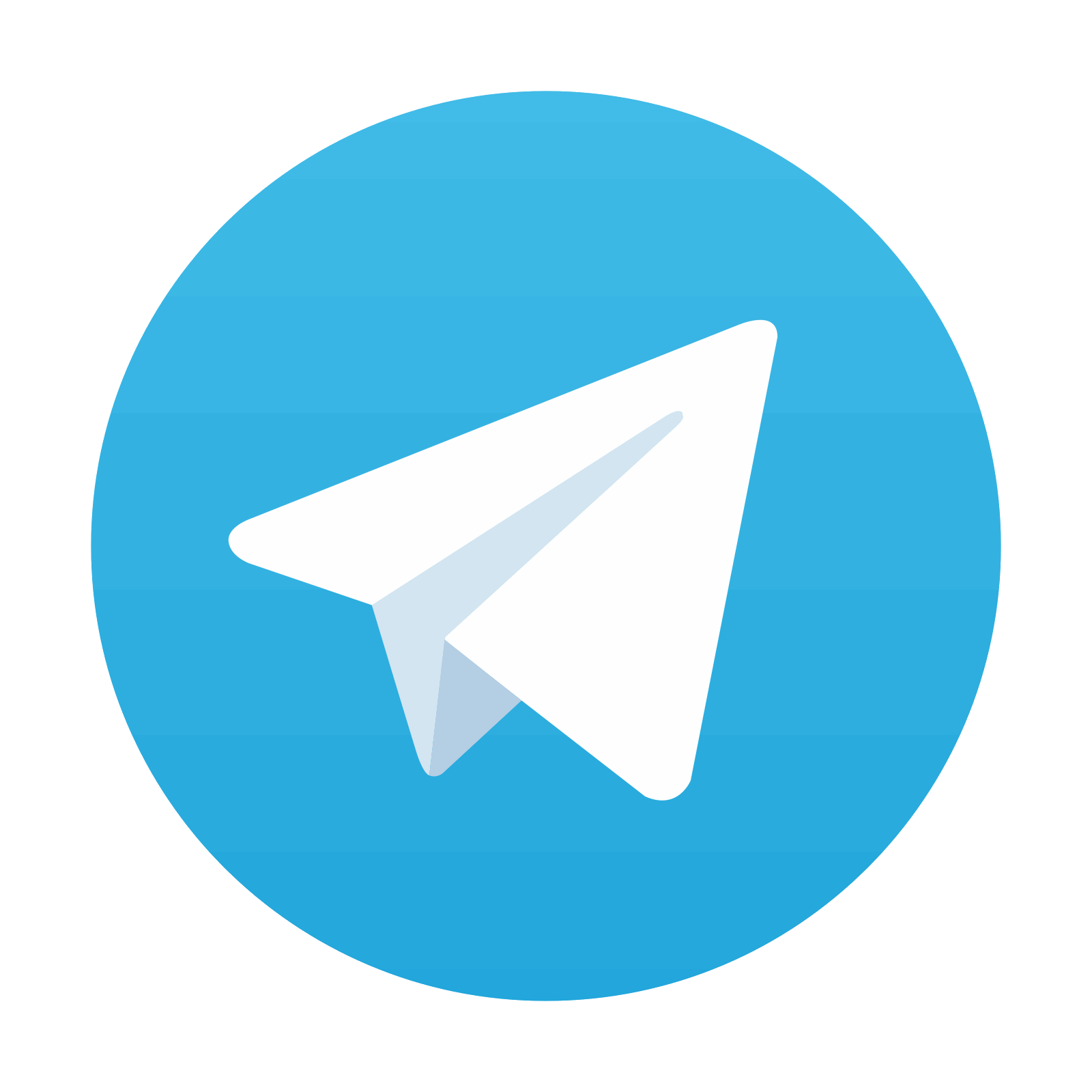
Stay updated, free articles. Join our Telegram channel

Full access? Get Clinical Tree
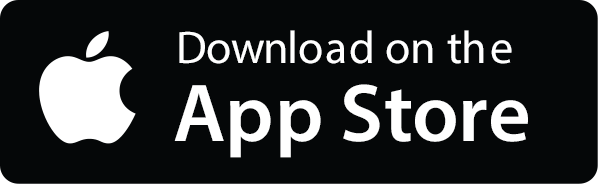
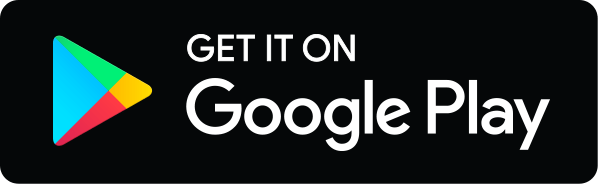
