Fig. 2.1
Dissemination tumor process. Metastasis process involves the relapse of tumor cells from primary tumor to target organ. This metastasis process is dependent to survival capacity of different subpopulation of CTCs into blood system
However this is a simplification of metastasis process, because these entire basic step have helped by multi epigenetic and genetic events which are in turn depends of different functional changes consequence of microenvironment (Cock-Rada and Weitzman 2013; Brabletz et al. 2013).
In addition, one fundamental step into of this metastasis cascade is the dissemination tumor cell phase. Through which, tumor cells acquire the capacity to circulate through bloodstream from primary tumor and finally colonize different organs to develop a metastasis (Pantel and Brakenhoff 2004).
Metastasis process presents inherent difficulties due to technological limitations in analyzing a complex in vivo process rich with heterotypic interactions. Invasion, survival in the circulation, and growth in distant organs are not amenable to methods that primarily use in vitro models. Despite technical challenges, elegant experiments that started in the 1950s were done with mouse xenograft models and resulted in an important descriptive understanding of the biology of metastasis. With the accumulation of knowledge from studying cancer cells in isolation, subsequent advances in metastasis built on the classic studies.
Unfortunately, metastasis remains responsible for the vast majority of cancer-related morbidity and mortality. Therefore, the understanding of this step into of metastasis process is a high priority.
In this chapter we will review our knowledge about the dissemination process, describing some important steps of this process in the metastasis of solid tumors.
2.2 Metastasis Process in Solid Tumor
The major cause of cancer-associated mortality is tumor metastasis. The metastasis process is known as the spread of cancer from the organ of origin (primary site) to distant tissues. Despite, our understanding about cell proliferation, cell death, genomic instability, and signal transduction pathways has rapidly progressed; our understanding of tumor metastasis is far from complete (Kovács et al. 2013; Lorusso and Rüegg 2012).
Cancer occurs after a cell is progressively genetically damaged and turns into a cell bearing a malignant phenotype. These cells are able to undergo uncontrolled abnormal mitosis, which leads to an increase of these cancerous cells at that location. In absence of regular control mechanisms a heterogeneous population of cells is created and these cancerous cells together form the primary tumor. A tumor is considered benign if it lacks the ability to invade other tissue. When cells acquire the ability to penetrate and infiltrate surrounding normal tissues, the cancer is considered malignant and has the potential to metastasize (Yokota 2000; Chaffer and Weinberg 2011).
Before tumor cells can start to metastasize, they need to succeed in stimulating angiogenesis. In this way tumor cells gain direct access to the blood circulation. This leads to improved access to the nutrients and oxygen carried by the blood, but also an opportunity for the tumor cells to enter the blood stream. This process is shown in Fig. 2.1 (Dissemination tumor process. Metastasis process involves the relapse of tumor cells from primary tumor to target organ. This metastasis process is dependent to survival capacity of different subpopulation of CTCs into blood system). An alternative route for tumor cells to end up in the blood circulation is through the lymphatic system. The release of cancer cells from the primary tumor to the peripheral blood appears even in patients with small cancer primary tumors (Cho 2010; Nadal et al. 2013; Ross and Slodkowska 2009).
Circulating tumor cells (CTCs) are probably the origin of incurable metastatic disease and an active area of cancer research (Massard and Fizazi 2011). The first observation of tumor cells in blood was made by Thomas Ashworth in 1869 (Ashworth 1869). In subsequent reports CTCs where only observed in blood when present in high number. As technology advanced it became possible to detect the presence of CTCs in a much lower concentration (Panteleakou et al. 2009; Sleijfer et al. 2007).
Tumor cells circulating in the blood can reach in principle most sites of the body. However, despite apparent similarities in clinical and/or histological features, different cancer types do not exhibit the same proclivity to metastasize to the same organs, and the same cancer type can preferentially metastasize to different organs. For example breast cancer generally creates metastases in liver, lung and bone; while prostate cancer is often metastasizes to bone.
This tissue tropism has long been recognized and has intrigued clinicians and pathologists to seek an explanation. James Ewing and others argued that tissue tropism could be accounted for based on mechanical factors and circulatory patterns of the primary tumor. In contrast, Stephen Paget proposed his “seed and soil” hypothesis (Fidler 2003; Paget 1889). This stated that the propensity of different cancers to form metastases in specific organs was due to the dependence of the seed (the cancer) on the soil (the distant organ). The first is mechanical of nature, a large amount of CTC arrests in the first capillary bed they encounter. The second is more biological, the CTCs will form a metastasis in tissue only if they are able to extravasate out of the blood stream and the local environment is suitable for them to grow. Tumor cells thus have a preference for a certain site, and this opens an interesting research field to identify the cell surface molecules on the tumor cells and the endothelial cells align in the capillaries at the specific sites. Current understanding would suggest that both seed and soil factors and anatomic considerations contribute to metastatic tropism. A modern interpretation of the seed and soil hypothesis is an active area of investigation, with molecular definitions accumulating for both the cancer and the microenvironment (Langley and Fidler 2011).
Numerous sequential steps are needed for metastasis, multiple genetic changes are envisioned. A failure in any step would prevent metastasis altogether. Accordingly, tumor cells that can accumulate a full complement of needed alterations to endow them with metastatic ability should be rare. These ideas are supported by early experiments. Work by Fidler and colleagues showed that subpopulations of tumor cells that display significant variation in their metastatic ability and metastatic lesions likely arose from single progenitor cells. Recent studies confirmed that metastasis is an inefficient process. These studies revealed that less than 0.01 % of tumor cells gave rise to metastases (Zhe et al. 2011).
2.3 Epithelial Mesenchymal Transition Process: Contribution to Tumor Aggressiveness
One of the principal events related with initiation metastasis function is epithelial mesenchymal transition (EMT) (Yao et al. 2011). During development, the generation of many adult tissues and organs results from a series of EMT events and the reverse process, a mesenchymal-to-epithelial transition (MET) (Thiery 2002; Aokage et al. 2011). EMT is a multi-step morphogenetic process during which epithelial cells down-regulate their epithelial properties and up-regulate mesenchymal characteristic (Fig. 2.2). EMT is a process by which epithelial cell undergo remarkable morphological changes characterized by a transition from an epithelial phenotype to an elongated fibroblastic phenotype. This event is characterized by epithelial cells loosening their cell-cell adhesion, losing cell polarity, and gaining the ability to invade and migrate under controlled cues. Important regulators include Notch and Wnt/β-catenin pathways, TGF-β family members, and FGF proteins that serve to set up regulatory networks involving EMT transcription factors such as Snail and Twist (Wu and Zhou 2008). These networks do not necessarily regulate cell fate, but rather drive morphogenetic movements by repression of the cell-cell adhesion protein E-cadherin, promoting cytoskeletal rearrangement, and increasing MMP activity. After cells complete EMT-mediated morphogenetic migration, they can then transiently differentiate into epithelial structures.
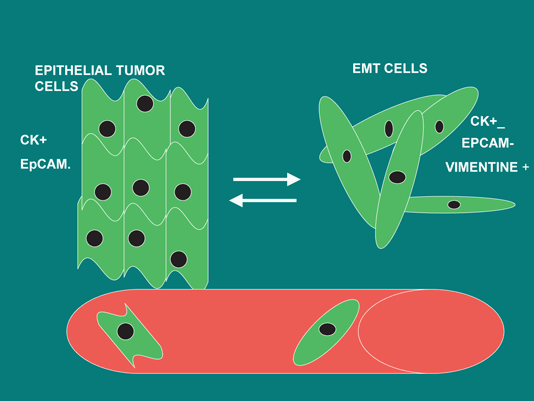
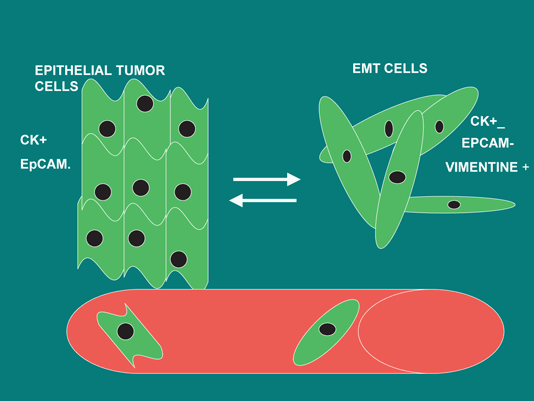
Fig. 2.2
EMT process. EMT process involves loss of epithelial marker and upregulation of mesenchymal markers. This event is necessary to initiation of metastasis
Among different factors that involve EMT process, several transcription factors have been said to drive this process, including members of the Snail and basic Helix Loop Helix (bHLH) families, and two double zinc finger and homeodomain (ZEB) factors. Between most characterized are ZEB 1 and ZBE 2, snail, slug and Twist. Interesting all these factors are regulated by selective pressures as hypoxia, inflammation, apoptosis, senescence and need for proliferative, metabolic and self-renewal sufficiency (Peinado et al. 2007; Tan et al. 2012).
Interesting, hypoxia can induce Snail and Twist, a direct target of HIF-1α.34. Low oxygen enhances β-catenin activity by inhibiting the activity of glycogen synthase kinase-3β, which normally induces the destruction of β-catenin. Accordingly, the presence of enhanced β-catenin signaling promotes Snail expression and subsequent EMT. Interestingly, the ability of hypoxia to liberate active β-catenin may set in place a feed-forward loop to help maintain EMT. Activation of Snail represses E-cadherin, which can then further enhance β-catenin and reinforce Snail expression (Tsai and Wu 2012; Lu and Kang 2010).
Similar to hypoxia, the inflammatory microenvironment can also promote EMT (Leibovich-Rivkin et al. 2013). It has recently been demonstrated that TNF-α, which is an inflammatory mediator secreted by TAMs, sets into motion a signaling cascade that funnels through NF-κB and glycogen synthase kinase-3β to stabilize Snail and β-catenin, and thus, enhances cancer cell migration. This ability of cancer cells to awaken an EMT program by co-opting an inflammatory microenvironment may be further reinforced by EMT itself. Snail-induced EMT is able to generate an environment of immunosuppressive T-regulatory cells and impaired dendritic cells partly through TGF-β and thrombospondin-1, helping to further perpetuate the inflammatory surroundings (Colotta et al. 2009).
Further hypoxia and inflammation, the need of CTCs to resist apoptosis and overcome senescence may be additional reasons to shoot EMT switch. Cells that have undergone EMT are associated with increased resistance to apoptosis (Savagner 2010). As mesenchymal cells do not necessarily have a direct contact to the basal lamina, they are not subject to anoikis and the EMT would therefore aid survival of the loosened cancer cells, possibly through prosurvival activity conferred by Snail and Twist. EMT can also help cancer overcome oncogene-induced senescence. Both Twist transcription factors and ZEB1 have been shown to suppress p21cip1 and/or p16ink4a, two p53-regulated cell cycle proteins that are critical in restraining oncogene-transformed cells via senescence (Smit and Peeper 2008). These findings suggest that the pressure to resist apoptosis and avoid senescence can result in activation of EMT transcriptional regulators. On the other hand, obtaining the ability to sustain long-term self-renewal would provide an advantage to cells in a growing tumor mass. Recent data suggest that EMT process is consistent with the acquisition of a “cancer stem–like” (CSC) phenotype (Pardal et al. 2003).
2.4 Circulating Tumor Cells and Cancer Stem Cells
The principal problems for the identification of CSC have result in problems to define CSCs in terms of origin and function. However it is well known that CSCs could be described like more or less differentiated cells; as well as, they are characterized by a peculiar phenotypic and genotypic plasticity (Tang 2012). Interestingly, we can detect these same characteristics into CTCs. In fact, similarly to CSCs, the molecular definition of CTCs presents the most significant difficulty to their clinical application. CTCs are a highly heterogeneous population of cancer cells, and their genotypic and phenotypic characterization not only to confirm their malignant origin but also to follow their immune-phenotypic changes with tumor progression and to identify diagnostically and therapeutically relevant targets for individual cancer therapies (Mavroudis 2010).
From the original observations of Bonnet and Dick on leukaemic stem cells (Bonnet and Dick 1997), many markers have been proposed for different CSCs (including CD133, CD44, CD24, and CD138; ABCG2, ABCB5, EpCAM, and ALDH1; CXCR4; various signaling pathways Hedgehog, Notch, Wnt/β-catenin, BM1, and PTEN; and different microRNAs) (Medema 2013).
On the other hand, recent studies have demonstrated the association among EMT process, metastasis development, recurrence with biology of CSCs. Recently it has been described the existence of stem cell-enriched subpopulations with a CD44 high/CD24 low cell surface marker profile, as well as having significantly higher levels of many EMT-related transcription factors, such as Snail and Twist, when compared with their CD44 low/CD24 high counterparts. Furthermore, these EMT transcription factors are able to directly increase the number of cells with stem-like characteristics. Although it remains to be determined whether EMT is occurring in cancer stem cells or whether EMT occurs in non–self-renewing cells that then give them stem-like properties, these data argue for a another compelling reason for cancer cells to acquire EMT properties (Tirino et al. 2013).
The connection between CSCs and CTC has been explained through the expression of EMT markers on CTCs suggesting that they are cancer stem cells. In fact, many CTCs do not express epithelial markers (EPCAM, Cytokeratin, etc) but express other markers like mesenchymal markers and stem markers (like Vimentine, Snail, N-cadherin, CD44, ALDH1).
The acquisition of these phenotypes involve cell-cell connection loss, while elasticity is gained by getting rid the rigid of cytoskeleton, facilitating passage of CTCs into the blood. More interesting, these EMT process is specially observed on single CTCs, while epithelial characteristics, like expression of EPCAM, are often observed in cell cluster (van de Stolpe et al. 2011). Thus, the gain of these capabilities to the extravasation and seeding in an organ tissue or evade the action of immune system are similar to normal mesenchymal stem cells. Finally, only the characterization of these CTCs like cancer stem cell could provide the assets to growth, like can be the reversion from EMT process to MET process (Mesenchymal Epithelial Transition) (Yao et al. 2011). Assuming this information is understandable that CTCs and CSC are the same population. Thus, CTSCs (circulating tumor stem cells) may hold to improve our known of metastasis process.
Recent results have shown that the tumor cells can adopt different characteristics, depending of type of microenvironment or niche (van de Stolpe 2013). Such that, some studies have demonstrated the expression of melanoma tumor marker in cells with endothelial mesenchymal, a phenomenon called “vascular mimicry” (Fan et al. 2013). Another example of this cell plasticity of CTCs, is their capacity to coexpression of specific tumor maker with the leucocyte marker CD45, symptomatic of the possibility the adaptation present in CTCs and suggesting the option that they represent a progeny of tumor stem cell (Conejo-Garcia et al. 2005).
In conclusion, the adaption capacity of CTCs, through the phenotypic and genetic plasticity present in these cells only can be explain because at least some CTCs presents feature of progenitor cancer cells. The presence of these specific characteristic involve the capacity of CTCs for adapting in the different microenvironments and opt for proliferation or enter in dormancy status.
2.5 Epigenetic Regulation of miRNA and Their Role in EMT Process
Cancer is a disease involving the failure of function of regulatory genes that control normal cellular homeostasis. Multiple mutations, almost all human cancers contain important epigenetic abnormalities that cooperate with genetic lesions to generate the cancer phenotype. Epigenetic aberrations arise early in carcinogenesis preceding gene mutations and therefore provide targets for early detection (Loeb et al. 2003).
MicroRNAs (miRNAs or miR) are small non-coding regulatory RNAs with sizes of 17–25 nucleotides (miRBase; http://microrna.sanger.ac.uk/). The first miRNA lin-4 and let-7 were identified by Ambros and Ruvkun in 1993 as a result of developmental studies on the worm Caenorhabditis elegans that identified important roles for noncoding RNAs (ncRNAs). In fact, in animal cells, post transcriptional regulation by miRNA requires an mRNA that is complementary to target sequence (position 2–7 of the mature miRNA). So, it is now appreciated that ncRNAs potentially influence cellular phenotypes by regulating of hundreds of target mRNAs (Gregory and Shiekhattar 2005).
The pattern of miRNA expression can be correlated with cancer type, stage, and other clinical variables. Thus, the analysis of miRNA’s expression, suggests the main role in different aspects of cancer biology such as proliferation, apoptosis, invasion/metastasis, and angiogenesis. In fact, several microRNAs, such as miR-10b, miR-9, and members of the miR-200 family, play critical roles in EMT or EMT-related events. For example, miR-10b influences cell migration, invasion, and metastasis by repressing HOXD10, a known inhibitor of a RHOC-mediated promotility program (Zadran et al. 2013).
The EMT is a complex series of morphological changes that conclude in the loss of epithelial characteristics and the acquisition of a mesenchymal motile phenotype. In the context of cancer, this process facilitates the dissemination of cancer cells and survival of CTCs until to seeding a secondary site where this process is reversible, the reversal of EMT is known like Mesenchymal Epithelial Transition (MET) (Kalluri 2009). Due to the dynamic nature of this process, epigenetic alterations are involved in dissemination, EMT, MET and finally metastasis. Interesting, this epigenetic regulation impacts miRNA that regulate gene pathway involve EMT process (Taube et al. 2013). In fact, in the context of the epigenetic regulation of EMT, it was found that the CpG island near the miRNA-200c and miRNA-141 transcription start is unmethylated in miRNA-expressing tumor-normal cells and is closely methylated in miRNA-negative and invasive tumor cells (Bullock et al. 2012). miRNA expression is further facilitated by the enrichment of chromatin-permissive histone modifications (H3 acetylation and H3K4 trimethylation). Davalos et al. demonstrated that in epithelial cancer cell lines, the 5ʹ-CpG islands of miRNA-200 family members are unmethylated, whereas the hypermethylation-mediated silencing of these miRNAs was found in transformed mesenchymal cells (Davalos et al. 2011). The reversibility of this methylation state mediates the shift between EMT and MET. Similar results were obtained in bladder cancer and breast cancer cell lines (Davalos et al. 2011). This results in increased histone acetylation and E-cadherin expression. Interestingly, the chemo- and radiosensitivity of these breast cancer cells was increased by enhanced p53-mediated apoptotic pathways (Mirzayans et al. 2012).
In this way, several miRNA has been involved in epigenetic EMT regulation. For example, miR-10b, miR-9, and members of the miR-200 family, play critical roles in EMT or EMT-related events (Samantarrai et al. 2013). The expression of miR-10b is under the control of the EMT transcription factor Twist. miR-10b influences cell migration, invasion, and metastasis by repressing HOXD10, a known inhibitor of a RHOC-mediated promotility program. The expression of miR-10b is under the control of the EMT transcription factor Twist. In addition, miR-10b is essential for Twist-induced EMT and for metastasis (Ma 2010). In contrast to miR-10b, which is specifically up-regulated in metastatic cancer cells, miR-9 expression is up-regulated in both metastatic and non-metastatic tumor cells relative to non-transformed cells. These observations, together with the fact that miR-9 expression can be increased by the actions of MYC and MYCN oncoproteins, cause us to propose that miR-9 expression is often induced at earlier stages of multi-step tumor progression (Nesbit et al. 1999). Thus miR-9 promotes metastasis by targeting E-cadherin, which results in β-catenin signaling and VEGF expression. Accordingly, motility, invasion, angiogenesis, and metastasis ensue. Consequently, miR-9 can be considered as a pro-metastatic miRNA and a negative regulator of the key metastasis suppressor, E-cadherin. Special importance has MiR-200 family members which also regulate EMT by inhibiting ZEB1 and ZEB2, through the suppression of E-cadherin (Gregory et al. 2008).
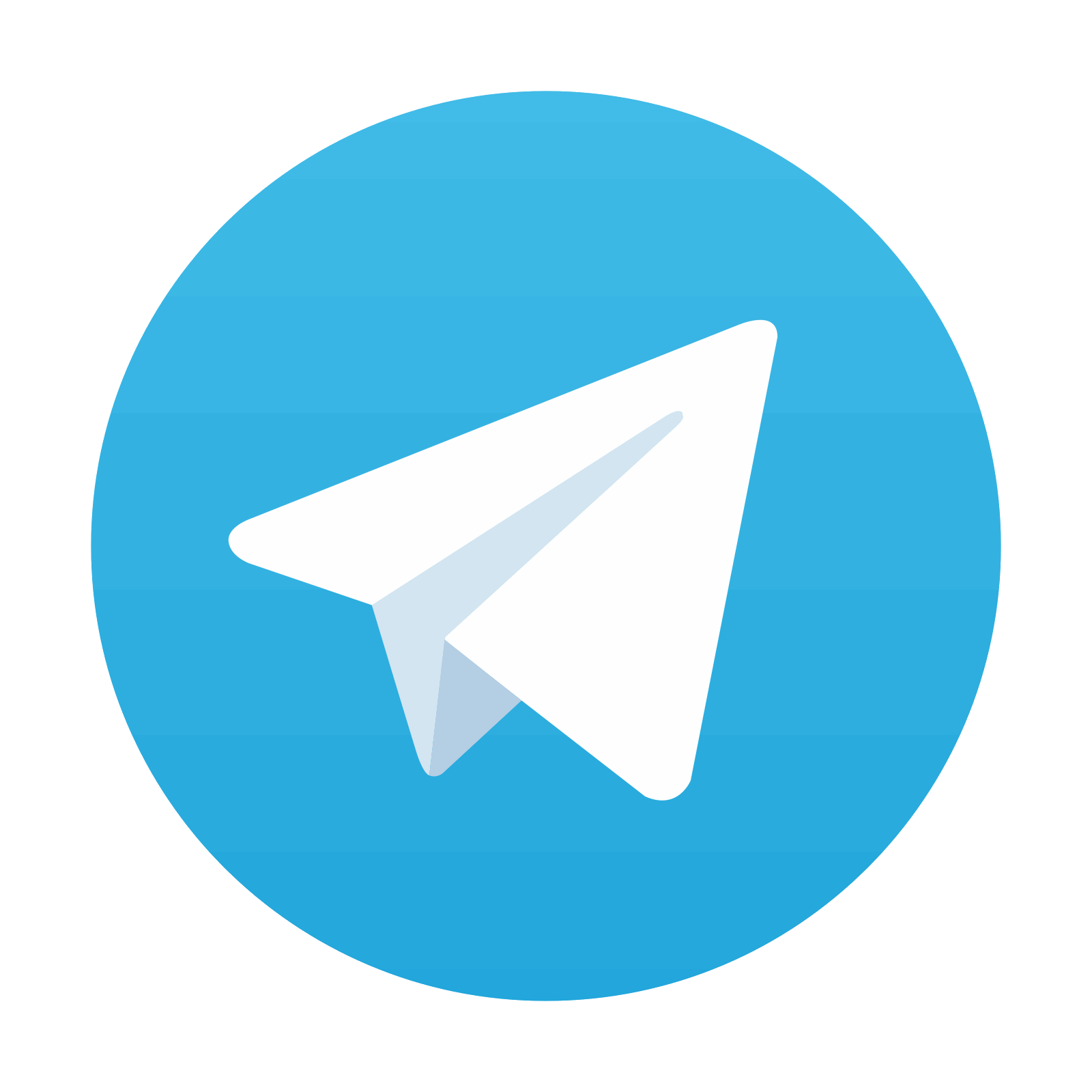
Stay updated, free articles. Join our Telegram channel

Full access? Get Clinical Tree
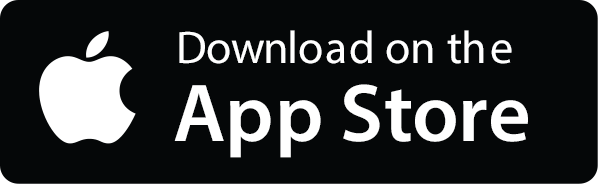
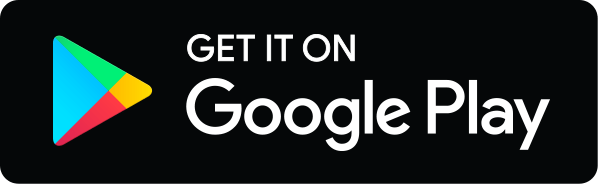