Fig. 4.1
Hematoxylin and eosin (H&E) picture of a tumor showing neoplastic, inflammatory, and stromal cells. H&E stained section of a tumor showing neoplastic cells (arrow head), inflammatory cells (double arrow head), and stromal cells (arrow) that compromise the typical tumor environment
4.2.1 Cell Culture
Purifying nucleic acid from cells grown in tissue culture is straightforward and will provide high quality, unfragmented DNA or RNA (assuming cells are not fixed in formalin). In addition, since cells are usually grown as a pure cell line, the percentage of DNA actually coming from the cells of interest should be 100 % assuming no others cells contaminating the culture. The difficulty in using nucleic acid from cell culture revolves more around having a thorough knowledge of what cells are actually growing in the culture. As older cell lines have undergone many passages and have been shared from laboratory to laboratory, it is not always certain that the cell line being used is actually the right one. A study in 2008 reported that 23 % of the cell lines tested had discrepant TP53 mutations [1]. Therefore, it is suggested to confirm the molecular traits of cell lines before they are used in crucial experiments [2, 3]. In addition to cell mix up, every time a cell replicates there is a chance for a mutation to occur. With some cell lines having been passaged for decades, there is a high likelihood that the cell lines of today contain many more alterations than they originally did.
4.2.2 Direct Human Samples
4.2.2.1 Fluids
Body fluids can often be a good source for human nucleic acid as they are often easier to access than solid tissues and require less invasive techniques to sample. Blood, more specifically the white blood cells within blood, can serve as a high quality source for germline DNA. Drawing a small tube of blood is easily performed in the clinic or outpatient setting and requires little equipment. It should be noted, however, that certain blood tubes may contain anti-clotting agents that can inhibit molecular analysis. It is generally recommended to use tubes containing ethylenediamine tetra acetic acid (EDTA) as an anticoagulant and to avoid tubes containing heparin as it is an inhibitor of many enzymes used in molecular analysis [4]. In addition to germline DNA, in the setting of leukemias, myelodysplastic syndrome, or now even circulating tumor cells from solid tumors, blood can also serve as a good source for somatic studies. Saliva, commercially available mouth rinses, and cheek swabs can also serve as a source of germline DNA. While these are often even easier to obtain than fresh blood, they can sometimes suffer from degradation and bacterial contamination [5–7]. The acquisition of nucleic acid from tumors can often be obtained from ascites or pleural effusions, often taken for therapeutic reasons from patients with cancer. However, a disadvantage in relation to these sample types is that the cells can suffer from varying degrees of degradation. Regardless of the source of fluid, if the intent is to obtain nucleic acid from tumor cells it is important for the fluid to also undergo cytologic analysis so it is known what cells are actually being captured.
4.2.2.2 Tissue
Fresh/Frozen Tissue
Fresh tissue will usually provide high quality DNA since it is not subjected to the crosslinking formalin causes, however, nucleic acid must be isolated from it quickly, or the tissue needs to be preserved. Typically, to store fresh tissue it is frozen in either a cutting media such as optimum cutting temperature (OTC) compound or snap frozen. OTC allows for the cutting of frozen section slides, which can be used for histological analysis and for nucleic acid purification. Freezing fresh tissue allows for the long-term storage of high quality nucleic acid. Fresh tissue is typically taken at the time of surgery, requiring protocols in place to quickly select and freeze the tissue. Due to the need for clinical pathologic analysis, the amount of tissue that can be taken for molecular analysis is often limited, especially if not for clinical testing. This tissue is usually selected by gross examination only, which can lead to missing the desired tissue. Therefore, it is recommended to always confirm the type of tissue through histologic analysis.
Formalin-Fixed Paraffin-Embedded Tissue
The typical workflow for any pathology department involves receiving specimens, performing a gross examination/description, and then preparing the specimen for histologic analysis., as described in Chap. 2. As formalin fixation and paraffin embedding of tissue is a standard procedure, there is a wealth of archived material in almost all pathology departments. FFPE tissue has several advantages over fresh frozen tissue that make it so widely used. These include being able to store blocks at room temperature and proving better histology. Formalin fixes tissue by forming cross-links between proteins, DNA, and RNA. Unfortunately, the purification of nucleic acid from FFPE material causes shearing of the nucleic acid. This is a major drawback and can limit the ability to use FFPE material in molecular analysis, depending on how long of nucleic acid fragments are needed for testing. Figure 4.2a shows an agarose gel of DNA purified from both fresh frozen and FFPE tissue. The large smears in the FFPE columns show sheared DNA of varying lengths, when in comparison, the fresh frozen column has a large band of DNA at the top of the gel indicating large-sized DNA. Figure 4.2b shows an RNA electropherogram showing the size of RNA fragments from fresh and FFPE tissue. The FFPE RNA sample is predominately composed of small fragments, when in contrast, in the fresh sample, one can see large 18S and 28S ribosomal RNA (rRNA) peaks. Despite these issues, FFPE tissue can often be used for a variety of testing [8].
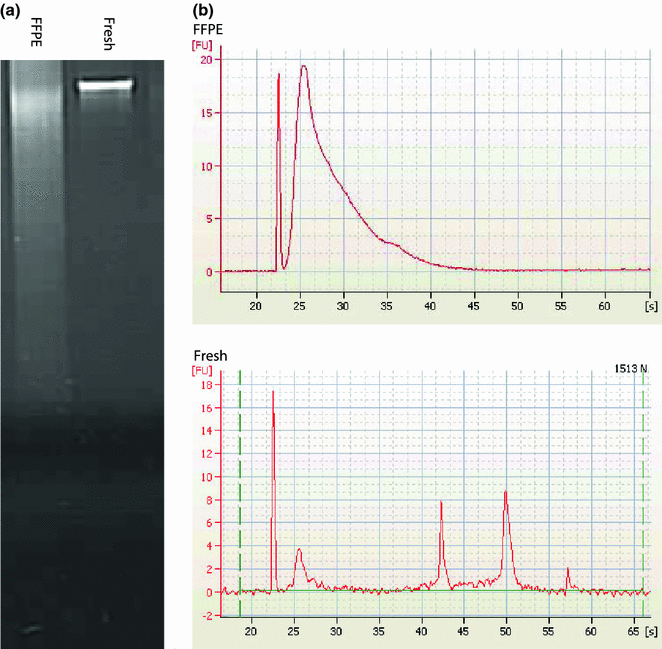
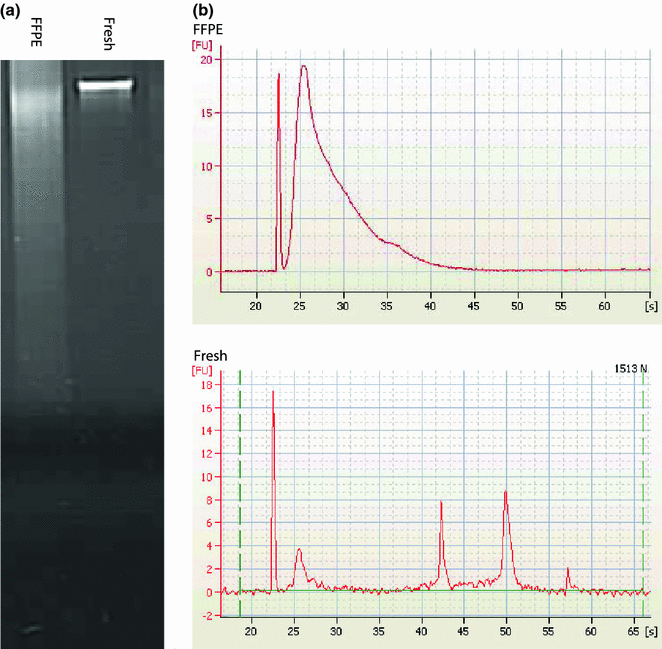
Fig. 4.2
Comparison of fresh and formalin-fixed paraffin-embedded (FFPE) nucleic acid quality. a Agorose gel of FFPE and fresh DNA showing a degraded smear for FFPE and a large band for fresh DNA. b Agilent bioanalyzer electropherograms showing the size of FFPE and fresh RNA showing degraded low-sized fragments of FFPE RNA compared to RNA from fresh tissue with large 18S and 28S rRNA peaks
Other Fixatives Used in Pathology
There are several other fixatives apart from formalin that can be sometimes used in pathology departments. Alcohol fixation (with either ethanol or methanol) will provide higher quality DNA and RNA than formalin. Bouin’s fixative or solution is a combination of formalin, picric acid, and acetic acid. While it provides excellent histology, the acid component degrades nucleic acid making most Bouin’s-fixed tissue unusable for molecular analysis. The same is true for many of the acid-based decalcification solutions used in pathology [9].
4.3 Nucleic Acid Purification
Following specimen collection and storage, whether fresh frozen or FFPE, the material to use for nucleic acid purification must be selected. Depending on how much material is available and how that material was stored, this process may need the assistance of a pathologist to review slides and select the appropriate area. Knowing that tumors can be very heterogeneous with widely varying amounts of actual tumor cells, it is important to think about proper material selection and isolation.
4.3.1 Type of Tissue Isolation
The least accurate, but usually the easiest way to get nucleic acid is to isolate it from a bulk piece of tissue. This is usually performed on frozen tissue, which can be pulverized and digested. The major drawback of using this technique is the lack of histological correlation and therefore, one does not know the amount of tumor in the sample.
Isolating nucleic acid by coring tissue blocks allows for fairly accurate selection of material when there are paired slides for histological analysis. It is also fairly fast and easy, as extra slides do not need to be made and scraped. When using a paired slide to select the areas to core, one is only visualizing the tissue in two dimensions (x and y-axis), while coring takes a lot of material vertically from the tissue block (z-axis). Therefore, it is a bit uncertain how much the tissue will change as one goes deeper into the block.
Macrodissection is possibly a more accurate method of obtaining the desired tissue. In this method, a series of sections are cut from a block and the first and last slide are mounted and stained for histological analysis. Using these two slides one can circle the area of interest to be dissected. Then, using a razor blade the region corresponding to the circled area can be scraped off the unstained slides for nucleic acid isolation. This technique allows for good localization and requires no special equipment. However, having to cut and scrape slides is time consuming and requires more resources than the methods mentioned above.
By far the most accurate method for tissue selection and isolation is laser capture microdissection. This technique is similar to macrodissection, except instead of scraping the unstained slides, the slides are stained, visualized under a microscope, and a special instrument is used to cut out the desired tissue [10]. The laser capture microdissection instrument consists of a microscope, slide scanner, and laser dissector. The operator marks the area to be dissected by direct visualization of a digital histological image. A laser then is used to cut out and grab the selected tissue. As a result, this technique allows for much finer areas to be dissected, even down to a single cell in some instances [11]. The major drawbacks to this method, however, are that it is time consuming and requires an expensive piece of specialized equipment.
No matter which technique is used to isolate nucleic acid, it is imperative to have some form of histological analysis. As new molecular techniques generate massive amounts of data, one must know what type of tissue the data is being generated on.
4.4 Analysis
There are an ever-growing number of molecular techniques used to analyze DNA and RNA. It is impossible to cover all of them in a single chapter. Therefore, this chapter will focus on the general technique of MPS or so-called “next-generation sequencing” (NGS) and a few other techniques that are often used in a molecular pathology laboratory.
4.4.1 Focused Analytical Techniques
4.4.1.1 Quantitative Real-Time Polymerase Chain Reaction
Quantitative real-time polymerase chain reaction (qPCR) utilizes a traditional PCR reaction with the addition of a reporter probe (Fig. 4.3) [12]. The reporter probe has both a fluorescent reporter and a quencher with a sequence of complimentary nucleic acid for binding. Due to the close proximity of the reporter and quencher, no fluorescence is seen. As the PCR primers get extended and approach the reporter probe, the 5′–3′ exonuclease activity of the Taq polymerase degrades the bound probe, releasing the fluorescent marker. The fluorescent marker can then be detected since it is no longer in close proximity to the quencher. Like the name of the technique suggests, qPCR can be used to quantify the amount of DNA. The more DNA is present, the more reporter probe is bound and thus more fluorescence is detected once the marker is released. A variation of this technique is reverse transcription (RT) qPCR. This method can be used to quantify the amount of an RNA transcript. For this, messenger RNA is purified and undergoes a RT step to form complementary DNA (cDNA) before the qPCR is performed. One example of a typical use of this technique is quantifying the amount of cDNA to determine titers of nonhuman RNA/DNA (e.g., viral) within a human sample [13]. The results can either be compared to a set of standards to determine the absolute amount of the pathogenic DNA or more commonly when analyzing human DNA and with RTqPCR, the DNA or mRNA amount is compared to an internal standard. In this way, the results can be reported as a ratio of the specific nucleic acid to the standard. These results can then easily be compared with other samples. For expression analysis, this internal standard is usually a common (or housekeeper) gene with a known, relatively constant expression. In addition to the above-mentioned uses, qPCR can also be used for the detection of a PCR product similar to standard PCR except the analyte is detected in “real time” so a detection method such as running the DNA out on a gel is not needed.
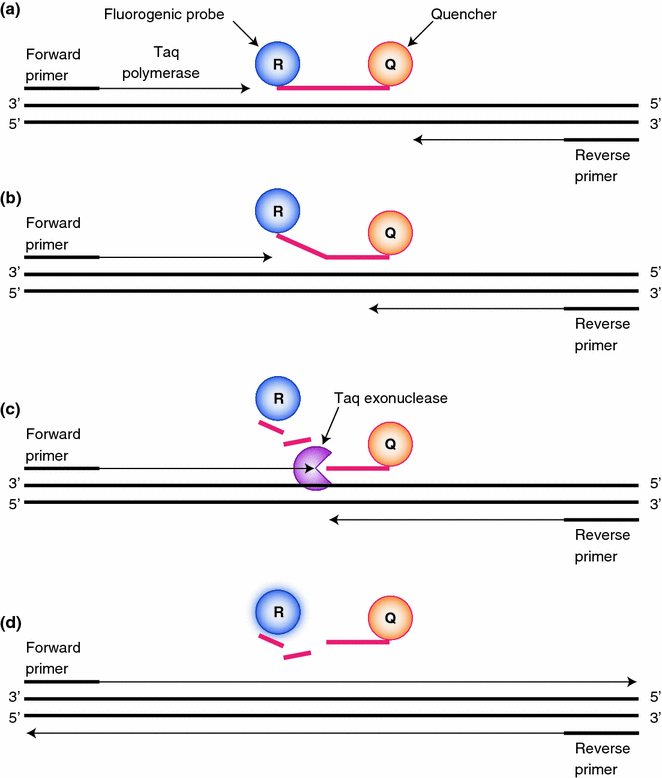
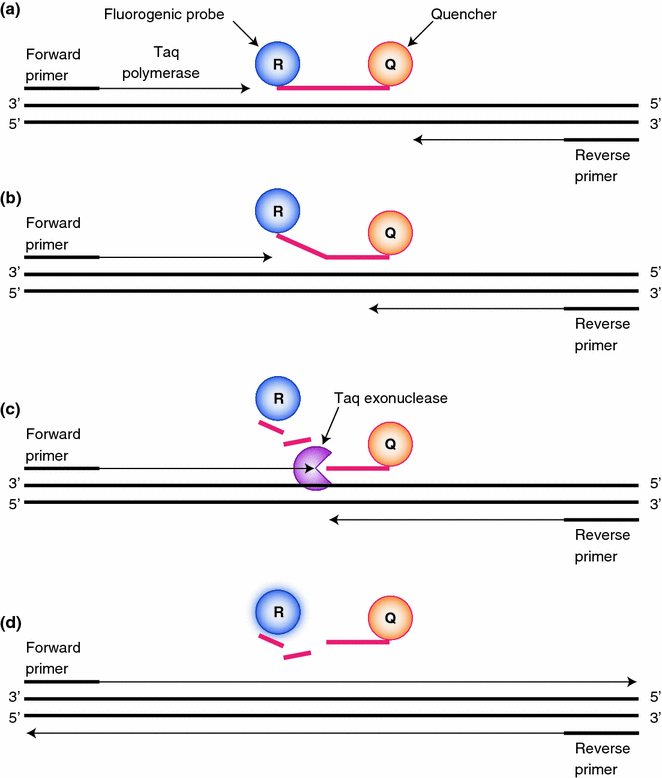
Fig. 4.3
Real-time PCR. a A nucleic acid probe that contains a fluorescent marker and quencher is bound to template DNA along with PCR primers. b Taq polymerase extends the PCR primer until it reaches the bound probe. c Taq exonuclease activity then cleaves the bound probe releasing the fluorescent marker. d Since the fluorescent marker is no longer in close proximity to the quencher, its fluorescence can be detected allowing detection and quantification
4.4.1.2 Mutation-Specific PCR
Mutation-specific PCR uses primers in the PCR reaction that are specific for a certain single nucleotide pleomorphism (SNP), or variant. In this reaction, the primer is designed so that it will only extend if the nucleotide being interrogated matches the primer (Fig. 4.4). There are multiple ways in which the primer can be designed to achieve this, however, one of the most common is to make the 3′ most nucleotide the SNP. Even if all of the other nucleotides match and the primer binds, the primer cannot get extended by the polymerase. A variant of this procedure intentionally designs a mismatch next to the nucleotide being interrogated. Therefore, if the interrogated nucleotide also does not match it will create two mismatches next to each other, the probe cannot stay bound, and amplification will not occur. In this technique, when designing the primer, the base of interrogation is placed in position-1 from the 3′ base and the intentional mismatch is placed at-2 from the 3′ base. Mutation-specific PCR is a fast, sensitive way to quickly determine if a specific mutation is present. The downside is that the alteration being interrogated must be known in advance. Due to the amplification of the target, mutation-specific PCR can be a very sensitive means of detecting rare sequence variations. However, as with any PCR reaction the utmost care needs to be taken to avoid contamination.
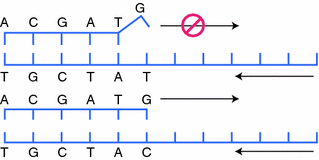
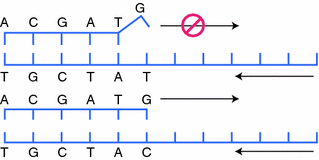
Fig. 4.4
Mutation-specific PCR. A PCR primer is designed so the 3′ most position is the nucleotide to be interrogated. a If that nucleotide does not match the template, a DNA polymerase will not extend the primer. b When the 3′ position matches, PCR will continue as normal and the product can be detected
4.4.1.3 In Situ Hybridization
In situ hybridization (ISH), introduced in Chap. 2, is a technique that can be used to detect RNA expression or DNA copy number. There are many variations of ISH depending on what is being detected, the type of probe, and the detection method. In general, it uses a nucleic acid probe to detect DNA or RNA in situ. This is often performed on touch preps, frozen section slides, or FFPE material. The probes are designed to be complimentary to the target sequence and are often labeled with a fluorescent (i.e., FISH) or chromogenic (i.e., CISH) reporter. A signal amplification step may be utilized for increased detection. DNA rearrangements and amplifications are typically detected with FISH (Fig. 4.5a) while RNA is often detected by CISH (Fig. 4.5b). For detection and quantification of the amount of a specific DNA or RNA, the probe is bound and the number of markers are counted. In the clinical setting, CISH is often used to detect Epstein-Barr virus-encoded RNA (EBER) inside human tissue [14]. One of the major advantages of ISH is the fact that it can be performed on a normal tissue slide allowing correlation with histology and confirmation that the proper cells are being interrogated. One of the drawbacks of ISH, similar to PCR, is that sequences near the target must be known. In addition, ISH is somewhat less sensitive as compared to PCR-based tests.
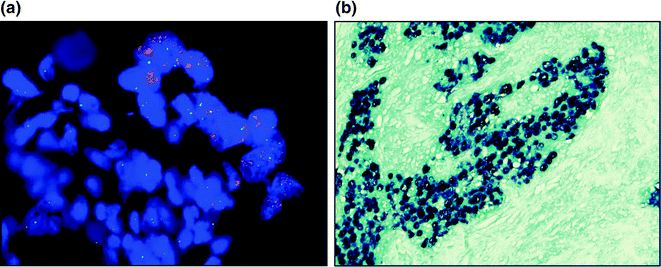
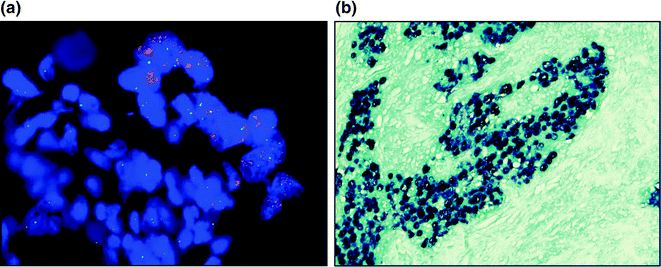
Fig. 4.5
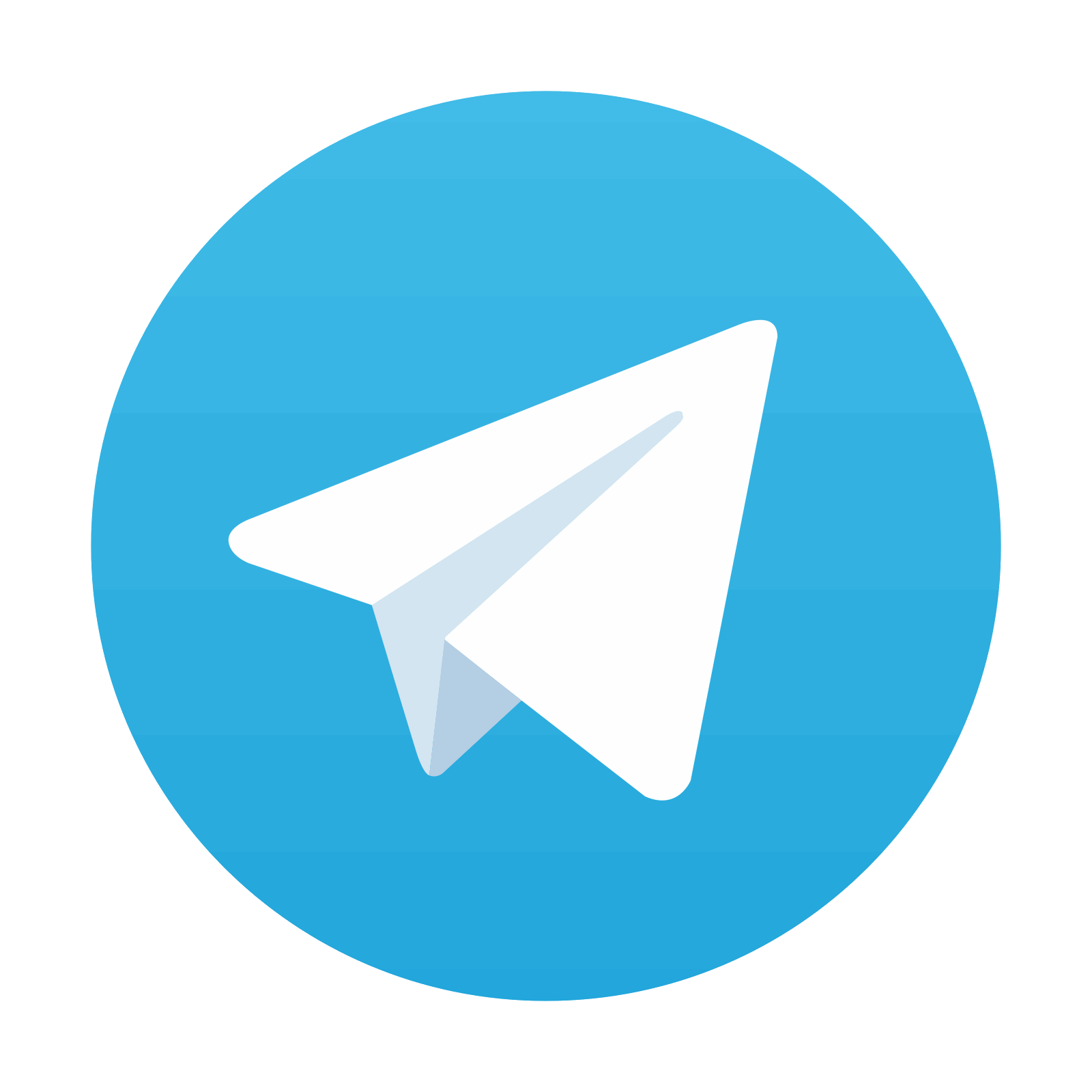
In situ hybridization. a Fluorescent in situ hybridization with ERBB2 (red) and CEP 17 (green) showing amplification of ERBB2. b Chromogenic in situ hybridization for epstein-barr encoding RNA. With the assay used, positive cells are dark blue, which is all of the cells in the picture
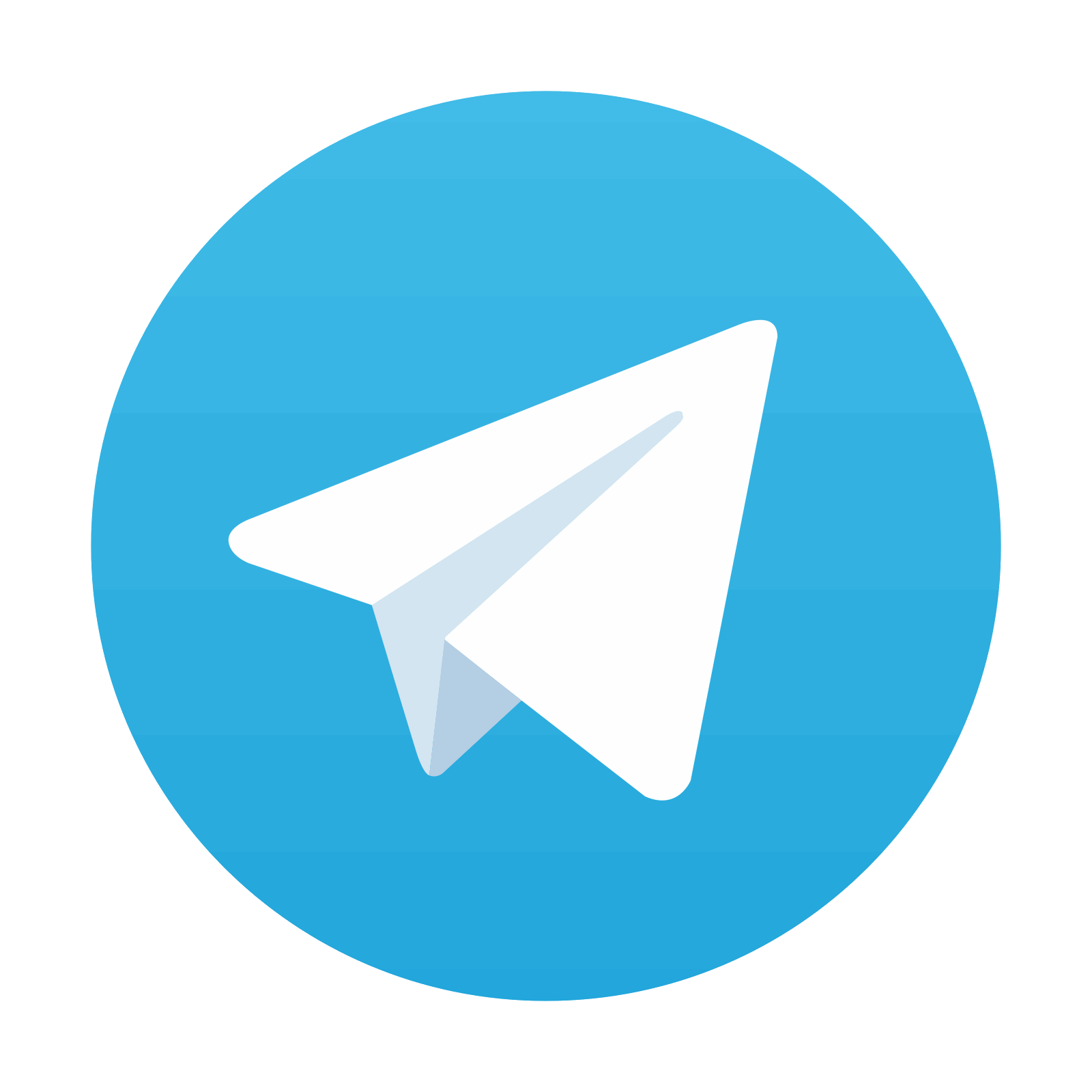
Stay updated, free articles. Join our Telegram channel

Full access? Get Clinical Tree
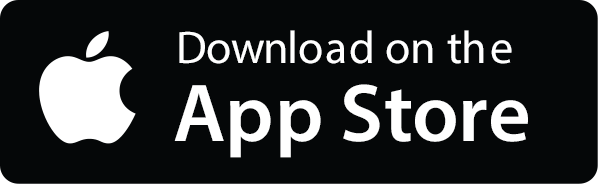
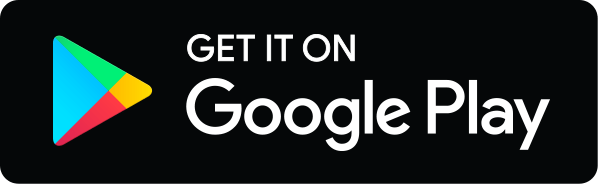
