Anorexia and cachexia
Takao Ohnuma, MD, PhD
Overview
Cancer cachexia is a wasting syndrome with extensive loss of skeletal muscle mass with or without adipose tissue. It may be contrasted with simple starvation in which fat replaces glucose as the preferred fuel to spare lean body mass. It results from altered metabolism rather than just an energy deficit, and cannot be reversed by conventional nutritional support. The causes of cancer-related cachexia are multifactorial including production of procachectic cytokines and metabolic derangements. European cancer community is credited in developing new definition and classification of cancer cachexia. Recent studies identified anamorelin, an oral ghrelin analogue, as a new agent for the treatment of cancer cachexia.
Introduction
Cachexia is a wasting syndrome that may accompany a plethora of diseases, including cancer, chronic obstructive pulmonary disease, AIDS, and rheumatoid arthritis. It is associated with central and systemic increases of pro-inflammatory factors, and with decreased quality of life, poor responses to pharmacological treatment and shortened survival.1, 2
Extensive loss of skeletal muscle mass with or without adipose tissue in cachexia may be contrasted with simple starvation in which fat replaces glucose as the preferred fuel to spare lean body mass. Cancer cachexia results from altered metabolism rather than just an energy deficit, and it cannot be reversed by conventional nutritional support.3, 4
Cachectic patients have a poor tolerance for surgery, chemotherapy, and radiotherapy.5–8 Cross-sectional images at the level of the fourth lumbar vertebra in patients who underwent surgery revealed that covariate-adjusted mortality increased significantly as lean core muscle area decreased.7–9
A lack of universally accepted definitions, diagnostic criteria and classification has impeded progress in both clinical trials and clinical practice. The definition and the classification of cancer cachexia developed in 2010 are shown in Tables 1 and 2.10
Table 1 Diagnosis of cancer cachexia
Measurement | Amount | Comments |
Weight loss | >5% | In past 6 months (in absence of simple starvation) |
or | ||
Body mass index | <20 | If weight loss >2% |
or | ||
Appendicular skeletal muscle index | Consistent with sarcopenia | |
Dual energy X-ray | Men < 7.26 kg/m2 | If weight loss >2% |
Women < 5.45 kg/m2 | ||
Absorptiometry | Men < 32 cm2 | Upper arm |
Women < 18 cm2 | ||
Anthropometry | Men <55 cm2 | Lumbar CT imaging |
Women <39 cm2 | ||
Bioelectrical impedance | Men <14.6 kg/m2 | Unreliable if obese or edematous |
Women <11.4 kg/m2 |
Source: Fearon et al. 2011.10 Reproduced with permission from Elsevier.
Table 2 Stages of cancer cachexia
Precachexia |
Anorexia, metabolic change, weight loss <5% |
Cachexia |
Weight loss >5% in past 6 months (without starvation) |
or |
Body mass index <20 and weight loss >2% |
or |
Anorexia and systemic inflammatory changes and sarcopenia and weight loss >2% |
Refractory cachexia |
Progressing catabolic state in a cancer usually unresponsive, low performance score and predicted survival < 3 months |
Source: Fearon et al. 2011.10 Reproduced with permission from Elsevier.
There is consensus that focus should move from end-stage wasting to supporting patients’ nutritional and functional state throughout the course of anticancer treatment. When inadequate nutrient intake predominates (malnutrition), this can be managed by conventional nutritional support. In the presence of systemic inflammation/altered metabolism that characterizes cachexia, a multimodal approach including novel therapeutic agents will likely be required. Three supportive care issues are critical: ensuring sufficient energy and protein intake, maintaining physical activity to maintain muscle mass, and (if present) reducing systemic inflammation. The results of phase II/III trials based on novel drug targets (e.g., cytokines, ghrelin receptor, androgen receptor, and myostatin) are expected by 2016. If effective therapies emerge, early detection of malnutrition and cachexia will be increasingly important.11
Etiology and mechanisms
The causes of cancer-related cachexia are multifold and can be grouped into three interrelated categories: anorexia and early satiety, mechanical obstruction of the alimentary tract, and metabolic derangement.
Anorexia in cancer patients can be disease-related, treatment-related and emotional distress-related. Anorexia may result from early satiety, nausea, or dysgeusia, a change in taste. Potential etiologic factors of anorexia and early satiety are presented in Table 3. Abnormalities of taste sensation and olfaction for specific food aromas have been demonstrated in cancer patients.48
Table 3 Possible causes of cancer anorexia
Name | Character | References |
Cytokines | TNFα | 121314 |
IL-1 | 141516 | |
IL-6 | 14 17181920212223242526272829303132 | |
Neuropeptides | Dysfunction in the brain circuits | 33 |
Serotonin | Increases in serum and central nervous system (CNS) | 34 35 |
Serum lactate | Tumor product | 36 37 |
Glucagon and similar peptides | 38 39 | |
Satietins | Proteins isolated in plasma | 40 41 |
Hypercalcemia | Occasional paraneoplastic findings | 42 |
Bombesin | Neuropeptide from small-cell lung cancer | 43 44 |
Toxohormone-L | Lipolytic factor purified from ascitic fluid of patients with hepatoma | 45 46 |
Anticancer agents (emetogenic) | Cisplatin, doxorubicin, nitrogen mustard, others | 47 |
Cytokines
TNF-α, IL-1, IL-6 [and its subfamily members such as ciliary neurotrophic factor (CNTF) and leukemia inhibitory factor (LIF)], and IFN-γ produced by host immune cells and/or tumor cells have all been implicated as mediators of cancer cachexia.49–51 These cytokines, called procachectic factors, are characterized in animals by the induction of anorexia, weight loss, an acute-phase protein response, protein and fat breakdown, rises in levels of cortisol and glucagon and falls in insulin level, insulin resistance, anemia, fever, and elevated energy expenditure. Direct interaction may occur with leptin, neuropeptides, or serotonin as mechanisms of induction of cancer anorexia. Anticachectic factors, IL-4, IL-10, IL-12, IL-15, IFN-α, and insulin-like growth factor I (IGF-I), act in opposition to procachectic factors.
Procachectic cytokines are produced by host immune cells including T helper 1 cells, macrophages, and myeloid-derived suppressor cells as part of a persistent inflammatory response. Proteolysis inducing factor (PIF) appears to be produced exclusively by tumors. PIF and TNF-α appear to induce cachexia through a similar pathway, by activating nuclear factor kappa B (NF-κB) transcription factor in muscles.52, 53
An elevated adrenergic state appears to be similar across tumor types resulting in elevated rates of energy expenditure.54, 55 The primary site of lean body mass depletion is the skeletal muscle, due to an increased protein turnover without equivalent protein synthesis.54 Available data suggest loss of myosin heavy chain (MyHC) and reduction in fiber size.54, 56 The adenosine triphosphate-ubiquitin-dependent pathway regulating protein breakdown is upregulated in certain cases of cancer cachexia.54, 57
IL-6 is a multifunctional cytokine involved in a variety of host defenses and pathological processes.17 IL-6-secreting cells can induce wasting of both muscle and fat stores and ultimately death.18, 19 Serum IL-6 levels were elevated in most experimental models of cachexia. IL-6 was demonstrated to be a sensitive predictor of weight loss in a number of series, including patients with advanced small-cell lung cancer20 and colon cancer.21, 22 Elevated IL-6 levels were associated with reduced survival in a variety of cancer types.23 In addition to IL-6 itself, other IL-6 family cytokines have also been implicated in muscle wasting, including CNTF24, 25 and LIF.26, 27
IL-6 and related ligands activate signaling by binding to ligand-specific α-receptors (IL-6 receptor-α, also known as gp80) in either membrane-bound or soluble forms.28 These ligand receptor complexes induce activation of three major pathways: the signal transducers and activators of transcription 1 and 3 (STAT1/3), ERK, and phosphatidylinositol 3-kinase/Akt pathways.29 These pathways interact with downstream kinases to influence gene expression.30
One pathway activated strongly by IL-6 family ligands is the JAK/STAT3 pathway. STAT3 activation was shown to be a common feature of muscle wasting, activated in muscle by IL-6 in vivo and in vitro and by different types of cancer and sterile sepsis. Moreover, STAT3 activation proved both necessary and sufficient for muscle wasting. Conversely, inhibiting STAT3 pharmacologically with JAK or STAT3 inhibitors reduced muscle atrophy downstream of IL-6. These results indicated that STAT3 was a primary mediator of muscle wasting in cancer cachexia and other conditions of high IL-6 family signaling.31, 32
Although the underlying mechanisms of muscle cachexia in cancer appear non-overlapping to those in the muscular dystrophies, a dysfunctional dystrophin glycoprotein complex (DGC) appears to be a common link between these two disease states.58–60
Tumor-induced alterations in DGC represent a key early event in cachexia.59 DGC was examined in muscle biopsies. Compared to healthy controls, in 60% of patients with gastrointestinal cancer, dramatic reduction in dystrophin was found, linked with hyperglycosylation of DGC proteins.59
While TNF-α induces IL-1, both cytokines exercise their anorectic effect through the brain as well as directly on the gastrointestinal tract, for example, slowed gastric emptying.12, 15 In carcinogen-induced tumors in rats, despite changes in other tissues, IL-1β and its receptor mRNA were the only ones upregulated in the brain, suggesting that they played a major role in this model of cancer-associated anorexia.16
Serum levels of circulating TNF-α, IL-1, IL-6 and IFN-γ did not correlate with the anorexia/weight loss syndrome in cancer patients.13, 14 A central mechanism of action in the production of cachexia has been postulated for many cytokines, including IL-1, IL-6, IL-8, TNF-α, IFN-α and other chemokines. Additional comments are pertinent for serotonin and dysfunction of neuropeptidergic circuits. A close relationship between elevated plasma-free tryptophan and anorexia was observed in patients with cancer.34 Increase in blood tryptophan results in elevated tryptophan levels in the cerebrospinal fluid, inducing increased synthesis of serotonin, a major mediator of cancer anorexia.35
Involvement of proteasome activity appears to be a rare event in cancer patients.54, 61 Some findings suggest involvement of the autophagic-lysosomal proteolytic system and cathepsin B during cancer cachexia development in humans.61, 62
Insulin and ghrelin
Insulin, secreted from the exocrine pancreas, and leptin, produced primarily by adipocytes, both circulate at levels proportional to body fat content. They enter the central nervous system (CNS) in proportion to their plasma levels. As weight increases, insulin secretion is increased both at the basal state and in response to meals. Insulin promotes fat storage and leptin synthesis by fat cells. Leptin has a more important role than insulin in the CNS control of energy homeostasis, for example, leptin deficiency causes obesity with hyperphagia that persists despite high insulin levels, whereas obesity is not induced by insulin deficiency.63, 64 Endogenous control of appetite by the neuropeptide network can be categorized as orexigenic and anorexigenic (Table 4). In cachectic tumor-bearing animals, lower circulating levels of leptin and decreased adipose tissue leptin mRNA content have been described.65 Similarly, serum leptin levels were reduced in patients with both advanced lung cancer and colon cancer.66, 67 Plasma leptin levels showed gender-dependent associations, and significantly lower levels were found among cachectic women than among cachectic men.68
Table 4 Orexigenic (anabolic) and anorexigenic (catabolic) neuropeptides
Orexigenic molecules |
Neuropeptide Y (NPY) |
Agouti-related protein (AGRP) |
Melanin-concentrating hormone (MCH) |
Hypocretin 1 and 2 (orexins A and B) |
Galanin |
Norepinephrine |
Opioids |
Ghrelin |
Anorexigenic molecules |
α-Melanocyte-stimulating hormone (α-MSH) |
Corticotropin-releasing hormone (CRH) |
Thyrotropin-releasing hormone (TRH) |
Cocaine- and amphetamine-regulated transcript (CART) |
Pro-opiomelanocortin (POMC) |
Insulin |
IL-1β |
Urocortin |
Glucagon-like peptide 1 (GLP-1) |
Oxytocin |
Neurotensin |
Serotonin |
Dopamine |
Histamine |
Cholecystokinin |
Bombesin |
Calcitonin-gene-related peptide |
Pituitary adenylate-cyclase-activating polypeptide |
Leptin |
Source: Data from Refs. 33 and 63.
Ghrelin, the “hunger hormone,” which functions as a neuropeptide in the CNS, is a peptide produced by ghrelin cells in the gastrointestinal tract. When the stomach is empty, ghrelin is secreted. When the stomach is stretched, secretion stops. Ghrelin acts on hypothalamic brain cells both to increase hunger and to increase gastric acid secretion and gastrointestinal motility in preparation for food intake. The receptors for ghrelin and leptin, with opposing effects, are found on the same brain cells. Ghrelin also plays an important role in regulating reward perception in the ventral tegmental area (a site that plays a role in processing sexual desire and developing addictions) through its interaction with dopamine and acetylcholine.
Ghrelin is encoded by the GHRL gene and is produced from the presumed cleavage of the prepropeptide ghrelin/obestatin. Full-length preproghrelin is homologous to promotilin and both are members of the motilin family.
Ghrelin’s peculiar structure, consisting of 28 amino acids with a single fatty acid n-octanoylated at Ser 3, not only allows binding to the growth hormone (GH) secretagogue receptor but also confers on the molecule a large variety of pleiotropic effects on different metabolic pathways.69 Two different forms of ghrelin originating from the same precursor are detectable in serum: an unacylated ghrelin (UnAG) and a lower concentration of acylated ghrelin. The acylation of ghrelin is performed mainly in the stomach by the intracellular ghrelin-O-acyltransferase, an enzyme only discovered in 2008. Acylated ghrelin, but not UnAG, can bind the growth hormone secretagogue receptor-1a (GHSR-1a), widely expressed in hypothalamic and pituitary regions, mediating GH release, able to enhance appetite and increase adipose tissue deposits. In a large variety of high-affinity binding sites, UnAG and acylated ghrelin act together, modulating the release of downstream mediators. In addition, novel receptors for UnAG in brain, gut, fat and muscle have been identified, which might promote cell proliferation, differentiation and survival.70
Ghrelin’s physiological functions include appetite modulation, enhancement of GH release, energy homeostasis, glucose homeostasis, anti-inflammatory action, effects on cardiovascular system, regulation of gastrointestinal tract motility, sexual function, and osteogenesis. GHSR is widely expressed in brain regions controlling body weight, emotional responses, memory, and learning.
The circulating level of ghrelin was reported to be increased in patients with muscle wasting and cancer cachexia. Ghrelin counteracted tumor-induced anorexia in animal systems, and its administration increased food intake and body weight gain.71–73
The main molecular mechanisms regulating the cancer anorexia-cachexia syndrome include alterations in brain neurochemistry. In particular, the hypothalamic melanocortin system appears not to respond appropriately to peripheral inputs, and its activity is diverted largely towards the activation of catabolic stimuli promoting metabolism of carbohydrates, lipids, and proteins in peripheral tissues leading to insulin resistance, increased lipolysis, and accelerated muscle proteolysis.74 Proinflammatory cytokines (TNF-α and IL-1β) and hypothalamic serotonergic neurons have been implicated in the dysfunction of the hypothalamic melanocortin system.33 Two peptide systems in particular appear to be strongly influential in the control of feeding behavior: these are the orexigenic neuropeptide Y (NPY), and the anorexigenic POMC (pro-opiomelanocortin) systems.75 Many of these mediators exert their effects through changes in the NPY/POMC systems. Both originate in the hypothalamic arcuate nucleus (ARC) and extend projections widely over the brain.75 The role of cytokines in cancer anorexia may depend on both the NPY and POMC systems. Hypothalamic IL-1 mRNA has been shown to be significantly increased in methylcholanthrene-induced sarcoma bearing rats. Levels of IL-1 in cerebrospinal fluid of these rats are also increased.76, 77 Injection of IL-1β into the hypothalamus causes significant change in gene expression in skeletal muscle within hours including upregulation of the ubiquitin proteasome pathway. This clearly demonstrates the potential for neural control of muscle protein synthesis and degradation.78 It has not yet been fully explored in patients with cancer cachexia.54
Alimentary tract dysfunction
Abnormalities in perception of taste and smell have been described in cancer patients. Tumors of the mouth, oropharynx, esophagus, stomach, pancreas, liver, and peritoneum may compromise oral intake by mechanical interference. Intestinal obstruction is a common complication of cancer. Malabsorption often occurs secondary to enzymatic insufficiency in pancreas carcinoma or secondary to lymphoma of the intestine or mesentery.79, 80
Delayed gastric emptying and slowing of peristalsis are pathogenic mechanisms that may contribute to early satiety.81 Early satiety is common in patients with decreased upper gastrointestinal motility.82, 83
Biochemical and metabolic derangement
High rates of glucose utilization with production of lactic acid are characteristic features of the neoplastic cell (Warburg effect). Glucose utilization by transplanted tumors in mice was second only to the brain.84 Hexokinase, which catalyzes the first step of the glycolytic pathway, often highly overexpressed in tumor cells, is a major player in this process. Binding of tumor hexokinase to the outer mitochondrial membrane provides the enzyme with preferential access to ATP generated in the mitochondrion and increases the activity and stability of the enzyme.85 The end product of the hexokinase reaction, glucose-6-phosphate, serves not only as a source of ATP via glycolysis but is also a key intermediate in the metabolic processes essential for cell growth and proliferation. Lactic acid produced via glucose metabolism may be utilized by other tissues for energy purposes or may be transported to the liver for resynthesis to glucose. The cyclic metabolic pathway, in which glucose is converted to lactic acid by glycolysis in tumor tissue and then reconverted to glucose in the liver, is referred to as the Cori cycle. Conversion of glucose to lactate in cancer cells yields two ATPs, whereas lactate to glucose conversion in the liver requires six ATPs. Thus, a systemic energy losing or futile substrate cycle, involving this interplay of tumor glycolysis and host gluconeogenesis, may be an important cause of cancer cachexia.86 Assuming that all lactate produced is recycled to glucose, the cancer cell acts as an energy parasite. It may be calculated, however, that if 85% of lactate passes through the gluconeogenic pathway and 15% is oxidized, the host’s handling of tumor-produced lactate would be energetically neutral. It has been suggested that the increase in the Cori cycle is insignificant in terms of energy expenditure and that increased glucose catabolism itself is responsible for weight loss and development of cachexia.87
Another mechanism of futile cycle is activation of the uncoupling proteins (UCPs) in brown adipose tissues (UCP1) and in skeletal muscles (UCP2 and 3).88–91 UCPs-3 mRNA levels were higher in the muscle of six patients with gastrointestinal adenocarcinomas with major weight loss than in six similar patients with stable weight and six controls. UCPs-2 mRNA levels did not differ significantly between groups.90 Elevations in muscle UCPs-3 activity may enhance energy expenditure and this in turn could contribute to tissue catabolism. Other mechanisms of muscle wasting such as mitochondrial dysfunction and activation of PPARγ coactivator-1 may also exist.92–95
Recently, extensive re-examination of Warburg effects has been made.96 Unlike most normal cells, many cancer cells derive a substantial amount of their energy from aerobic glycolysis converting most incoming glucose to lactate even in the presence of oxygen. Although ATP production by glycolysis can be more rapid than by oxidative phosphorylation, it is far less efficient in terms of ATP generated per unit of glucose consumed. This shift therefore demands that tumor cells implement an abnormally high rate of glucose uptake to meet their needs for energy, biosynthesis, and redox. Aerobic glycolysis putatively provides a biosynthetic advantage for tumor cells in that a high flux of substrate through glycolysis allows for effective shunting of carbon to key subsidiary biosynthetic pathways. A key molecule produced as a result of altered cancer metabolism is reduced nicotinamide adenine dinucleotide phosphate (NADPH), which functions as a cofactor and provides reducing power in many enzymatic reactions crucial for macromolecular biosynthesis. NADPH is also an antioxidant and forms part of the defense against reactive oxygen species (ROS) that are produced during rapid proliferation. High levels of ROS can damage macromolecules, inducing senescence and apoptosis. Glutathione (GSH) and thioredoxin (TRX), antioxidant molecules, are maintained in reduced form by NADPH. In addition to the genetic changes that alter tumor cell metabolism, factors of the microenvironment such as hypoxia, pH, and low glucose concentrations play a major role in determining the metabolic phenotype of tumor cells. It has been shown that cancer cells secrete hydrogen peroxide. As a consequence, oxidative stress in cancer-associated fibroblasts drives autophagy, mitophagy, and aerobic glycolysis. This “parasitic” metabolic coupling converts the stroma into a “factory” for the local production of recycled and high-energy nutrients (such as L-lactate) to fuel oxidative mitochondrial metabolism in cancer cells. A loss of stromal Cavolin-1 (Cav-1) is identified as a new biomarker for hypoxia, oxidative stress, autophagy, and the “reverse Warburg effect”, leading to early tumor recurrence and poor clinical outcome.97, 98
Reactive oxygen species (ROS) and oxidative stress
Metabolic reprogramming of distant adipose tissues and muscle occurs in cancer cachexia. Fatty acids are taken up and catabolized by cancer cells to generate ATP and promote tumor growth. Cancer cells frequently invade adipose tissue, inducing the release of free fatty acids from adjacent adipocytes and this release promotes tumor growth.
High levels of free fatty acids in the plasma are a key feature of cancer cachexia and advanced cancer. Fatty acids are the preferred plasma catabolites utilized in cancer cachexia, as measured by comparing plasma disappearance of 14C-labeled palmitate and glucose and measuring generation of 14C-labeled CO2. Free fatty acids are catabolized to generate acetyl-CoA, ketone bodies, and ATP in tumors. In sum, the catabolic effects exerted by cancer cells on remote adipocytes and myocytes are similar to those that cancer cells exert on fibroblasts adjacent to cancer cells or cancer-associated fibroblasts.
Adipose triglyceride lipase (ATGL) and hormone sensitive lipase (HSL) are key enzymes in the generation of free fatty acids by adipocytes and ultimately in ketogenesis. High ATGL and HSL activity is implicated in cancer cachexia with myocyte apoptosis and loss of muscle mass. Muscle wasting is induced by autophagy and lysosomal degradation in cancer cachexia. ATGL and HSL knockdown studies reveal that they mediate muscle degradation. This highlights the significant reciprocal interactions between metabolism, autophagy, and cachexia.99
MicroRNA (miR-), Hormonal Aberration, and Tumor Parasitism, Signaling pathway
Regulation of gene expression has been linked to miR-378 in a human cachexia model.100, 101
Low levels of testosterone in males with advanced cancer102, 103 and high plasma cortisol values in patients with malignant tumors have been reported.104, 105
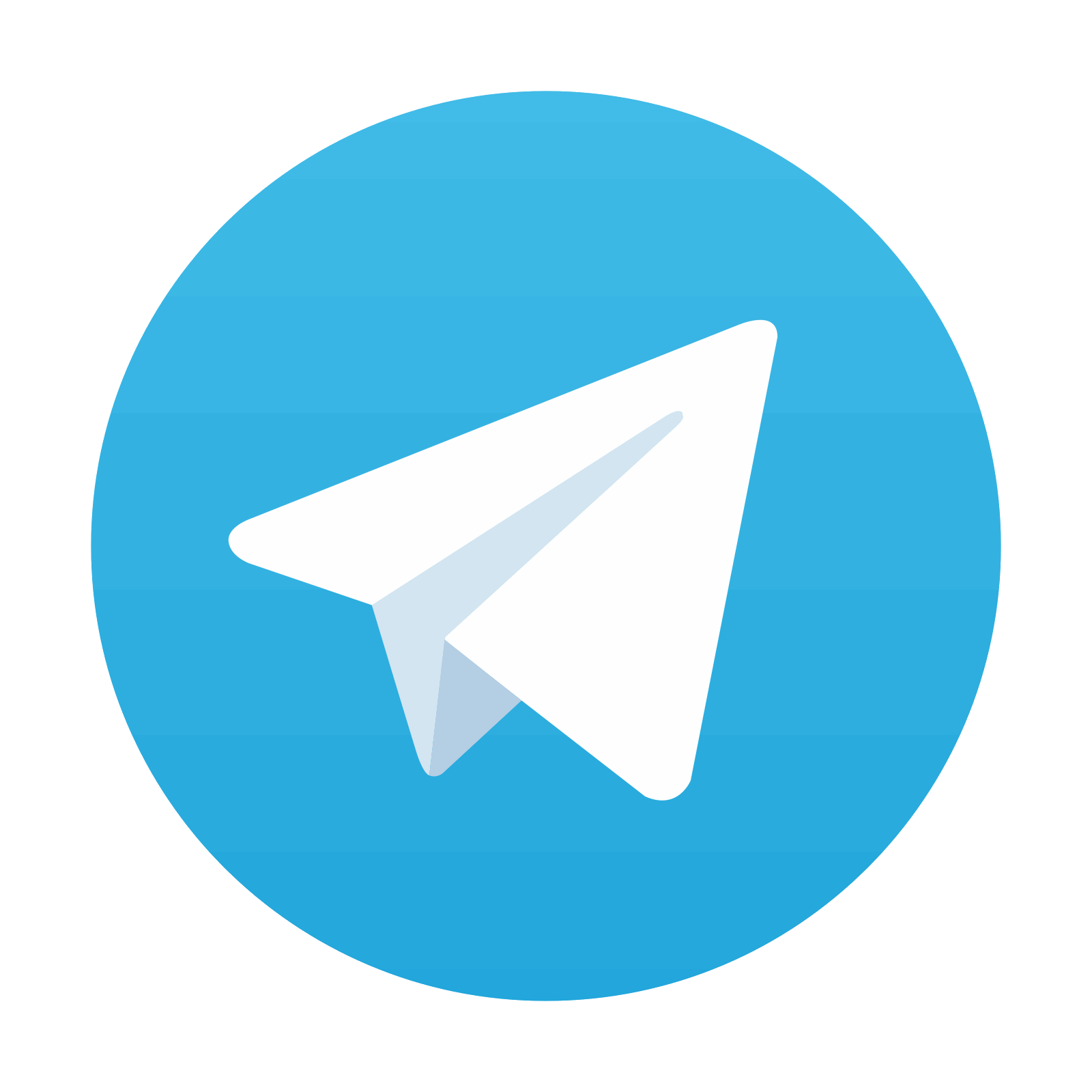
Stay updated, free articles. Join our Telegram channel

Full access? Get Clinical Tree
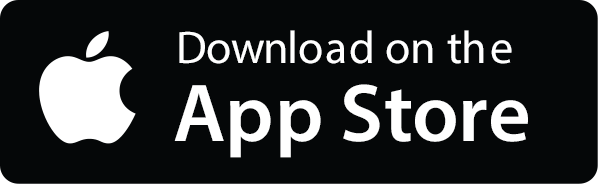
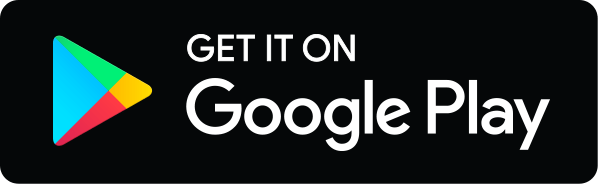