Aneurysms (Large and Small Arteries)
Carlos E. Sanchez
Sangita Kapur
Neal L. Weintraub
Aneurysms of the aorta and its branch vessels are a major health care concern; more than 15,000 patients die each year in the United States from aortic aneurysms, and the incidence and prevalence continue to rise.1 Unfortunately, most aneurysms are asymptomatic, and their presentation is often acute with catastrophic complications.2
As the largest blood vessel of the body, the aorta serves as a conduit to deliver oxygenated blood from the left ventricle to the systemic circulation and all organs and tissues. The normal human adult aortic wall is composed of three layers. The intima is the innermost layer and consists of the endothelium residing on a basement membrane with subendothelial connective tissue; the media is a thick layer composed of layers of elastic fibers arranged concentrically with interposed smooth muscle cells bounded by an internal and external elastic lamina; and the outer layer, the adventitia, is composed of a loose collagen network containing the vasa vasorum and nerves. The aorta is divided into thoracic and abdominal portions defined by the location above or below the diaphragm. The thoracic aorta is subsequently divided into four parts: the aortic root, which consists of the aortic valve annulus, aortic valve cusps, and the sinuses of Valsalva; the ascending aorta, which begins at the sinotubular junction and extends to the brachiocephalic artery; the aortic arch, which begins at the origin of the brachiocephalic artery and gives rise to the neck and head artery branches; and the descending aorta, which begins at the isthmus between the left subclavian artery and the ligamentum arteriosum and ends at the diaphragm. Below the diaphragm, the abdominal aorta continues caudally, giving rise to mesenteric and renal arteries and ending at the bifurcation of the common iliac arteries. The abdominal aorta is divided into suprarenal and infrarenal portions based on the location relative to the takeoff of the renal arteries.
The normal size of the aorta varies by anatomical location, age, gender, body surface area, and imaging modality. On a study based on helical computed tomography (CT) in patients without cardiovascular disease, the mean thoracic aortic diameters were 2.98 cm at the aortic valve sinus, 3.09 cm at the ascending aorta proximal to the innominate artery, and 2.43 cm at the descending thoracic aorta at the level of the diaphragm. In general, the normal abdominal aorta exhibits a diameter of approximately 2 cm.3 Aneurysms can develop in all portions of the aorta and in smaller arteries. The purpose of this chapter is to summarize the key aspects of aneurysm disease of the aorta and its major branches, focusing on pathogenesis, diagnosis, and management.
ABDOMINAL AORTIC ANEURYSM
Abdominal aortic aneurysm (AAA) is considered to be present when the anteroposterior diameter of the aorta is ≥3.0 cm4 or when the vessel wall exceeds the normal diameter by 50%.5 The infrarenal aorta is the most common site of AAA; populationbased screening programs have demonstrated an incidence of up to 9% in asymptomatic adults.6 The incidence and prevalence of AAA is on the rise, and whether this is related to the implementation of ultrasound screening programs, widespread use of CT, an aging population, or a true increase in the disease incidence is unclear. Despite advances in the treatment options for aneurysms, rupture continues to be the most widely recognized and feared complication with an overall mortality rate of up to 90%.7
Risk Factors and Pathophysiology
Multiple risk factors have been recognized to be associated with AAA. Smoking is strongly associated with the development of AAA; in particular, the duration of smoking, and not the number of cigarettes smoked per day, has been associated with the increased risk.8 In addition, males exhibit a prevalence of AAA that is five- to sixfold greater than females.9 The risk of rupture in women, however, is significantly higher than in men, and aneurysm rupture occurs at smaller aortic diameters in women.10 The prevalence of AAA increases with age, with the highest rate in patients older than 65 years. Hypertension and having a first-degree relative with AAA have also been associated with increased risk for AAA. Family history of AAA also carries a greater risk of rupture, and aneurysms tend to occur at a younger age.11
Atherosclerosis is closely linked to the pathophysiology of AAA. Although AAA frequently occurs in patients with atherosclerosis, and both diseases share many of the same risk factors, they exhibit distinct pathologic features and anatomic predilection. The hallmark pathologic feature of atherosclerosis is foam cell formation, whereas aneurysms are typified by intense matrix degradation, inflammation, oxidative stress, and apoptosis of smooth muscle cells. Additionally, atherosclerotic lesions are predominantly intimal in location, whereas the media and adventitia are primarily involved in AAA. The media and adventitial layers contain large amounts of matrix structural proteins, particularly elastin and collagen, which impart strength to resist aortic expansion and wall tension.6 Degradation of the extracellular matrix proteins weakens the aortic wall with subsequent remodeling and dilatation. Once the aorta dilates and an
aneurysm is formed, tension in the aortic wall increases progressively based on Laplace law (tension is proportional to the product of pressure and radius), causing the aneurysm to enter a progressive and sustaining loop of enlargement.
aneurysm is formed, tension in the aortic wall increases progressively based on Laplace law (tension is proportional to the product of pressure and radius), causing the aneurysm to enter a progressive and sustaining loop of enlargement.
One of the defining features of AAA is inflammation.11 The inflammatory infiltrate is most prominent in the media and adventitia of the aortic wall and is composed predominately of macrophages and T lymphocytes, although neutrophils, B lymphocytes, NK cells, and mast cells are also present.12 Activated macrophages are a major source of matrix metalloproteinases (MMPs), a group of structurally related, zinc-dependent endopeptidases that are also synthesized by vascular wall cells, particularly smooth muscle cells. In addition, macrophages secrete serine proteases and cysteine proteases that work in concert with MMPs to degrade structural proteins, thereby playing a key role in aneurysm formation.13,14 MMPs are secreted as inactive zymogens (pro-MMPs) that require activation extracellularly. Among the numerous classes of proteases that can lead to tissue destruction, MMP-2 and MMP-9 have in particular been implicated in the pathogenesis of AAA. Deletion of either MMP-2 or MMP-9 prevented AAA formation in a mouse model.15 Also, MMP-9 was reported to be the most abundant protease secreted by human AAA tissue explants in vitro.16 Additionally, MMP-9 transcript levels were found to be higher in aneurysms measuring 5.0 to 6.9 cm compared to aneurysms measuring 3.0 to 4.9 cm.17 In a study in ruptured AAA, MMP-8 and MMP-9 levels were increased at the aneurysm rupture site compared to the adjacent unruptured wall, suggesting that extracellular matrix breakdown may be the final mechanism causing AAA rupture.18 Notably, systemic inhibitors of MMPs abrogated AAA in mouse models and are under study in humans with AAA.13,19,20
Lymphocytes, particularly CD4+ T-helper cells, have a unique activated memory phenotype and produce proinflammatory cytokines (e.g., interleukin-6, tumor necrosis factoralpha, interferon-gamma, and osteopontin), leading to further recruitment of immune cells and induction of apoptotic cell death. Apoptosis of smooth muscle cells within the aneurysmal wall is thought to contribute to aneurysm formation and progression, since smooth muscle cells are largely responsible for the production of elastin and collagen. The degradation of aortic extracellular matrix proteins by proteases, together with a reduced capacity to synthesize new matrix proteins due to smooth muscle cell apoptosis, may thus lead to impaired repair of the aortic wall resulting in weakening and dilatation.21
Oxidative stress induces MMP activation and smooth muscle cell apoptosis and is increased in the setting of AAA (reviewed in ref.21). A study of aortic tissue in patients undergoing surgical repair demonstrated that levels of reactive oxygen species (ROS) are increased locally in AAA tissue as compared with adjacent nonaneurysmal aorta, in part through the activation of NADPH oxidase, leading to marked increases in oxidative stress.22 A subsequent study in mice demonstrated that deletion of p47phox, a cytosolic subunit of NADPH oxidase, reduced aortic oxidative stress, macrophage infiltration, and MMP-2 activity and markedly attenuated AAA formation.23 ROS also stimulate the secretion of cyclophilin A from vascular smooth muscle cells. Cyclophilin A, a member of the immunophilin family of proteins and a target of the immunosuppressive drug cyclosporine, binds to the highly glycosylated protein CD147 (also known as extracellular MMP inducer) on smooth muscle cells and induces its translocation to the cell surface, where it plays a critical role in stimulating MMP activity and matrix degradation. Absence of cyclophilin A reduced aortic inflammation, oxidative stress, and matrix degradation and prevented AAA formation and rupture in a mouse model.24 An example of the typical pathologic features of human AAA is shown in FIGURE 72.1.
Mural thrombus is frequently present lining the walls of AAA and can be both prognostically and clinically significant. Although AAA size is the most commonly used determinant to predict the risk of rupture, some studies suggest that the presence of intramural thrombus portends a higher AAA expansion rate and risk of rupture.25,26 The presence of a mural thrombus in AAA has been associated with a thinner arterial wall, lower density of smooth muscle cells in the media, and heightened inflammation and elastolysis in the aneurysm wall.27 This might be caused by the increased production of proteases originating from neutrophils and other circulating cells trapped within the mural thrombus in the aneurysmal wall.28 While intramural thrombus size and growth has been suggested to be prognostically significant in asymptomatic patients with aneurysms ≤5.5 cm in size, larger studies are needed to confirm this association before it is incorporated in the decision making of timing of aneurysm repair.
Diagnosis
AAAs are frequently asymptomatic and commonly detected as an incidental finding on imaging studies or, in the present era, through AAA screening programs. Multiple imaging modalities are currently available for diagnosing and monitoring AAA with excellent sensitivity and specificity. The indication of each type of imaging modality for AAA is determined by availability, cost/benefit ratio, and technical considerations. Ultrasonography is the preferred modality for screening AAAs, with the advantage that it is relatively inexpensive, noninvasive, and it does not require administration of a contrast agent or expose patients to radiation. The evaluation of AAA with ultrasound involves measuring the anteroposterior, longitudinal, and transverse dimensions of the aorta. The sensitivity, specificity, and accuracy to detect and quantify infrarenal AAA approaches 100%.29 Limitations of this imaging technique are that it is technician dependent, difficult to implement in morbidly obese patients, associated with significant interobserver variability, and usually not able to visualize aneurysm extension to major branches (i.e., iliac arteries), which limits its utility for planning operative repair.
The use of routine ultrasound screening for AAA in asymptomatic patients is a controversial topic. The U.S. Preventive Service Task Force (USPSTF), in its 1996 report, found insufficient evidence to recommend routine AAA screening in asymptomatic adults. Since then, numerous randomized, controlled trials evaluating population-based screening for AAA have been published. The largest trial, the Multicenter Aneurysm Screening Study, randomized 67,800 patients aged 65 to 74 years to an invitation for abdominal ultrasound screening or to an uninvited control group. After 4 years of follow-up, the AAArelated mortality in ultrasound-screened patients was reduced by 42% compared to uninvited controls.30 The trial also provided firm estimates of the cost-effectiveness of a screening program. At 4 years, the cost was 57,000 dollars per qualityadjusted life year, and when extrapolated to 10 years, the cost was estimated at 12,500 dollars. The authors concluded that at
4 years, the cost-effectiveness of screening for AAA is acceptable according to the current health services threshold.31 Based on these data, and three other population-based studies, the USPSTF revisited the benefits and harms of population-based AAA screening in 2005, concluding that for men aged 65 to 75 years, an invitation to attend AAA screening reduces AAArelated mortality.32 Unfortunately, these data do not specifically address AAA screening in women, who were underrepresented in the trials. The use of screening ultrasound for AAA in the United States is currently recommended for men aged 65 to 75 years who have smoked, and for both men and women in this age group with a family history of AAA.
4 years, the cost-effectiveness of screening for AAA is acceptable according to the current health services threshold.31 Based on these data, and three other population-based studies, the USPSTF revisited the benefits and harms of population-based AAA screening in 2005, concluding that for men aged 65 to 75 years, an invitation to attend AAA screening reduces AAArelated mortality.32 Unfortunately, these data do not specifically address AAA screening in women, who were underrepresented in the trials. The use of screening ultrasound for AAA in the United States is currently recommended for men aged 65 to 75 years who have smoked, and for both men and women in this age group with a family history of AAA.
CT is a well-established and widely used method for the diagnosis of AAA. The advent of multidetector CT, with its ability to perform volumetric imaging with isotropic voxels and a spatial resolution <1 mm, makes it an excellent modality for the diagnosis and follow-up surveillance of AAA. The multiplanar imaging ability of CT renders it an extremely accurate technique to serially evaluate changes in aneurysm size over time. In addition, CT permits better visualization of suprarenal aneurysms and improved assessment of the renal, mesenteric, and iliac arteries through axial images and three dimensional (3-D) reconstruction displays, thereby providing a complete description of the abdominal and pelvic vasculature prior to repair (FIGURE 72.2). An important use of CT is to measure the infrarenal neck of the aneurysm, the length of which is extremely important for endograft fixation during endovascular AAA repair. The infrarenal neck of the aneurysm is defined as the distance along the aorta from the renal arteries to the proximal origin of the aneurysm. Disadvantages of CT are its cost compared to ultrasound, exposure to radiation (particularly with repeated scans), and the use of intravenous contrast.
Noncontrast or contrast-enhanced magnetic resonance angiography (MRA) also clearly demonstrates the aortic anatomy, providing similar information to that of the CT scan. MRA is highly sensitive and specific at diagnosing and quantifying aortic diameter in AAA. In addition, MRA delineates the entire aorta and thus defines the anatomic relationship between the aorta and its principal branches for preoperative aortic aneurysm assessment. Advantages of MRA over CT scan in patients who need serial monitoring of aortic size are the absence of ionizing radiation and iodinated contrast; however, MRA is much more expensive and less widely available. Moreover, some patients cannot tolerate MRA due to implanted metal devices and/or claustrophobia and longer imaging times.
Management
Surveillance and Timing of Repair
Aneurysm size remains the single-most important predictor for aneurysm rupture and the main indication for repair in asymptomatic patients with AAA. The size of the aneurysm is directly related to the risk of rupture; however, the exact size upon which surgical intervention is justified remains a topic of debate. The optimal management and timing of surgical repair of AAA was addressed by two large randomized trials, the U.K. Small Aneurysm Trial and Aneurysm Detection and Management (ADAM) Veterans Affairs Cooperative Study. Both
trials randomized patients with small aortic aneurysm diameters (4.0 to 5.5 cm in U.K. Small Aneurysm Trial and 4.0 to 5.4 cm in ADAM Study) to undergo early elective surgery or follow-up surveillance. Collectively, there was no difference in survival between the early surgery group and the surveillance group after approximately 5 years of follow-up. The U.K. Small Aneurysm Trial extended their follow-up to 8 years, showing a slightly lower total mortality in the early surgery group. However, their 30-day surgical mortality rate was 5.8% compared to 2.1% in the ADAM trial.33,34 The U.K. Small Aneurysm Trial also reported an annual risk of AAA rupture of 1.5% or less for aneurysms smaller than 5.0 cm in diameter and 6.5% for aneurysms 5.0 to 5.9 cm in diameter. Above 6 cm, the risk of AAA rupture rises sharply, with an annual risk of rupture of up to 33% in aneurysms 7.0 cm in diameter or larger.10,35 These trials demonstrated that in patients with asymptomatic AAA, follow-up surveillance and deferment of repair until aneurysm diameter exceeds 5.5 cm is safe and associated with a very low rate of rupture. An important limitation is that female population was underrepresented in these trials; consequently, recommendations made for men may not apply stringently to women. Currently, the threshold for repair recommended by professional vascular surgery societies in women with AAA is 4.5 to 5.0 cm, based on the recognition that aneurysms tend to rupture at smaller sizes in women than in men.36
trials randomized patients with small aortic aneurysm diameters (4.0 to 5.5 cm in U.K. Small Aneurysm Trial and 4.0 to 5.4 cm in ADAM Study) to undergo early elective surgery or follow-up surveillance. Collectively, there was no difference in survival between the early surgery group and the surveillance group after approximately 5 years of follow-up. The U.K. Small Aneurysm Trial extended their follow-up to 8 years, showing a slightly lower total mortality in the early surgery group. However, their 30-day surgical mortality rate was 5.8% compared to 2.1% in the ADAM trial.33,34 The U.K. Small Aneurysm Trial also reported an annual risk of AAA rupture of 1.5% or less for aneurysms smaller than 5.0 cm in diameter and 6.5% for aneurysms 5.0 to 5.9 cm in diameter. Above 6 cm, the risk of AAA rupture rises sharply, with an annual risk of rupture of up to 33% in aneurysms 7.0 cm in diameter or larger.10,35 These trials demonstrated that in patients with asymptomatic AAA, follow-up surveillance and deferment of repair until aneurysm diameter exceeds 5.5 cm is safe and associated with a very low rate of rupture. An important limitation is that female population was underrepresented in these trials; consequently, recommendations made for men may not apply stringently to women. Currently, the threshold for repair recommended by professional vascular surgery societies in women with AAA is 4.5 to 5.0 cm, based on the recognition that aneurysms tend to rupture at smaller sizes in women than in men.36
![]() FIGURE 72.2 CTA volume rendered (A) and maximum-intensity projection (MIP) images (B) demonstrating a fusiform bilobed AAA with atherosclerotic calcifications (arrow). |
Rapid expansion rate is another important determinant that can also predict aneurysm rupture, with large aneurysms typically expanding more rapidly than small aneurysms in accordance with Laplace law. This hampers the exact prediction of aneurysm growth rate, even within the same individual over time. Based on multiple reports, however, the average annual expansion rates for AAA are 0.1 to 0.5 cm for aneurysms measuring <4.0 cm at the time of discovery, 0.4 to 0.5 cm for aneurysms 4.0 to 6.0 cm, and up to 0.7 to 0.8 cm for larger aneurysms.37 In general, follow-up surveillance imaging is recommended every 12 months for individuals with AAA measuring 3.5 to 4.4 cm in diameter and every 6 months for those with an AAA measuring 4.5 to 5.4 cm in diameter, with a recommendation for elective aneurysm repair when diameter reaches 5.5 cm or increases by more than 0.5 cm within a given year.38
Endovascular versus Surgical Repair
Endovascular abdominal aortic aneurysm repair emerged almost two decades ago as an alternative approach to AAA repair in individuals not deemed to be surgical candidates. Endovascular aneurysm repair (EVAR) now has become the treatment of choice to repair infrarenal AAA, accounting for more than half of all AAA repairs in the United States.39 The technique involves the use of a percutaneous stent graft, placed under fluoroscopy guidance to cover the aneurysm, excluding it from the circulation. It usually requires sufficient proximal and distal nonaneurysmal segments for adequate attachment of the stent struts to prevent distal migration. It is important to note that AAA endovascular repair has certain anatomical requirements for procedural success, and not all patients with AAA have suitable anatomy.40 The implantation success rate, however, has approached 100% in recent years. Nevertheless, a common adverse effect continues to be the leakage of blood into the aneurysm, also called endoleak. Although they may spontaneously resolve, persistent endoleaks may lead to continual aneurysm expansion or rupture, requiring an additional intervention to repair either by endovascular implantation of a graft extension cuff or, less commonly, by conversion to open surgical repair.
Two randomized, prospective trials comparing AAA endovascular repair versus open surgical repair in individuals deemed healthy enough to undergo surgery have addressed the shortto late-term outcomes of EVAR. In the short-term outcomes, the Endovascular Aneurysm Repair trial 1 (EVAR-1), and the Dutch Randomized Endovascular Aneurysm Management (DREAM) trial, showed an almost identical improved 30-day mortality rate after EVAR compared to open surgical repair: 1.7% versus 4.7%, respectively, in the EVAR-1 trial and 1.2% versus 4.6% in the DREAM trial, respectively. Mid- and longterm data, however, have failed to demonstrate an all-cause mortality benefit, with overall survival rates converging after 2 years for both groups.41,42 The role of endovascular repair in
patients considered at high risk for complications or death with open repair has also been investigated in the United Kingdom Endovascular Repair-2 (EVAR-2) trial. The study randomized patients to elective endovascular AAA repair or no repair, with both groups equally treated medically. The study found no benefit of endovascular repair in patients who are deemed high risk for open aneurysm repair, even at long-term followup.43 Therefore, the optimal indications for endovascular repair remain to be precisely defined. Patient preference is very important when making treatment decisions, and patients should be well informed about the pros and cons of each approach.
patients considered at high risk for complications or death with open repair has also been investigated in the United Kingdom Endovascular Repair-2 (EVAR-2) trial. The study randomized patients to elective endovascular AAA repair or no repair, with both groups equally treated medically. The study found no benefit of endovascular repair in patients who are deemed high risk for open aneurysm repair, even at long-term followup.43 Therefore, the optimal indications for endovascular repair remain to be precisely defined. Patient preference is very important when making treatment decisions, and patients should be well informed about the pros and cons of each approach.
Medical Management
The cornerstone of medical therapy is risk factor modification. Current tobacco use has shown to be strongly associated with a significant increase in aneurysm growth rate. Hence, smoking cessation should be strongly encouraged in all patients with AAA and is likely the single-most important therapeutic intervention. Hypertension should also be controlled. Several studies have suggested that beta-blockers and angiotensin-converting enzyme inhibitors have a beneficial effect on aneurysm progression, but these data have been insufficient to make strong recommendations for their specific use to prevent aneurysm expansion.44 Patients with AAA frequently have concomitant coronary artery disease, and beta-blockers are generally recommended for patients undergoing vascular surgery to reduce perioperative cardiac risk. Despite no clear relationship between cholesterol levels and AAA expansion rate, statin therapy has been shown in small studies to reduce infrarenal aneurysm growth rate and to reduce aortic MMP-9, which plays an important role in aneurysm formation and expansion.45,46 In a recent study, the effects of statins on aneurysmal growth was examined in 150 patients with AAA over a median period of 3 years. Statin users averaged 2.0 mm/y aneurysm growth rate compared to 3.6 mm/y in nonusers.47 A recent meta-analysis also suggested that statin therapy is associated with a lower rate of expansion in patients with small AAA.48 Although these results are encouraging, larger trials are needed to confirm efficacy in order for statin therapy to be recommended for the treatment of AAA, in the absence of conventional indications.
Inflammatory AAA
Inflammatory AAA (IAAA) has been described as a variant of AAA defined by the presence of a thickened aneurysm wall, marked perianeurysmal and retroperitoneal fibrosis, and dense adhesions of adjacent abdominal organs.49 IAAA account for 3% to 10% of AAA with a male-to-female predilection reported of up to 30:1 and with an age of occurrence 5 to 10 years younger than the mean age of patients with atherosclerotic aneurysms.50 Unlike atherosclerotic AAA, symptoms of IAAA are common and highly suggestive of inflammation. Patients usually present with the triad of abdominal or back pain, weight loss, and an elevated sedimentation rate.51 As with all AAA, inflammatory cell infiltrate is the pathophysiologic hallmark, but with a more pronounced inflammatory response in IAAA. Histologic features include internal elastic lamina disruption and loss of smooth muscle and elastic fibers of the media along with inflammatory infiltrates.49 The exact etiology is unclear, but it has been hypothesized that enhanced inflammation might be related to infections such as Chlamydia and cytomegalovirus.52,53 The rupture rate for IAAA has been suggested to be lower than noninflammatory AAA. CT scan is the gold standard diagnostic tool for IAAA, characterized by a thick inflammatory soft tissue rind around the aorta and demonstration of perianeurysmal fat stranding indicative of inflammation, which distinguishes IAAA from the more common atherosclerotic AAA.51 More recently, increased metabolism in the AAA wall has been visualized by 18F-fluorodeoxyglucose (FDG) positron emission tomography (PET)/CT. FDG uptake has been shown to correlate with inflammation, histopathologic characteristics of aneurysm wall instability, and clinical symptoms and may contribute in the near future to improved prediction of AAA rupture risk.54 Surgical repair is the treatment of choice for IAAA, although EVAR has emerged as an option. Findings at operation include a thickened aortic wall and a shiny, white periaortic and retroperitoneal inflammatory reaction involving adjacent structures.51
Aortic Dissection
Aortic dissection is a tear of the intima layer with bleeding into the wall of the aorta resulting in separation of the layers and formation of a new lumen (false lumen). In 90% of patients, the dissection plane tracks within the media layer. Population-based studies have shown that the incidence of acute aortic dissection ranges from 2 to 3.5 cases per 100,000 person-years, twice that of AAA rupture.55 The most common predisposing factor for acute aortic dissection is arterial hypertension, with a strong male predominance. Other predisposing factors include genetic conditions such as Marfan syndrome or Loeys-Dietz syndrome, nonsyndromic familial disorders, previous aneurysms, atherosclerosis, and inflammatory diseases. Underlying genetic disorders are particularly common in patients younger than 40 years of age who suffer aortic dissection, and only a small minority of these patients have a history of aortic aneurysm prior to dissection.56 Thus, in most patients, aortic dissection represents a syndrome distinct from aortic aneurysm. For a comprehensive review on aortic dissection, please refer to ACC/AHA guidelines for the diagnosis and management of patients with thoracic aortic disease.57
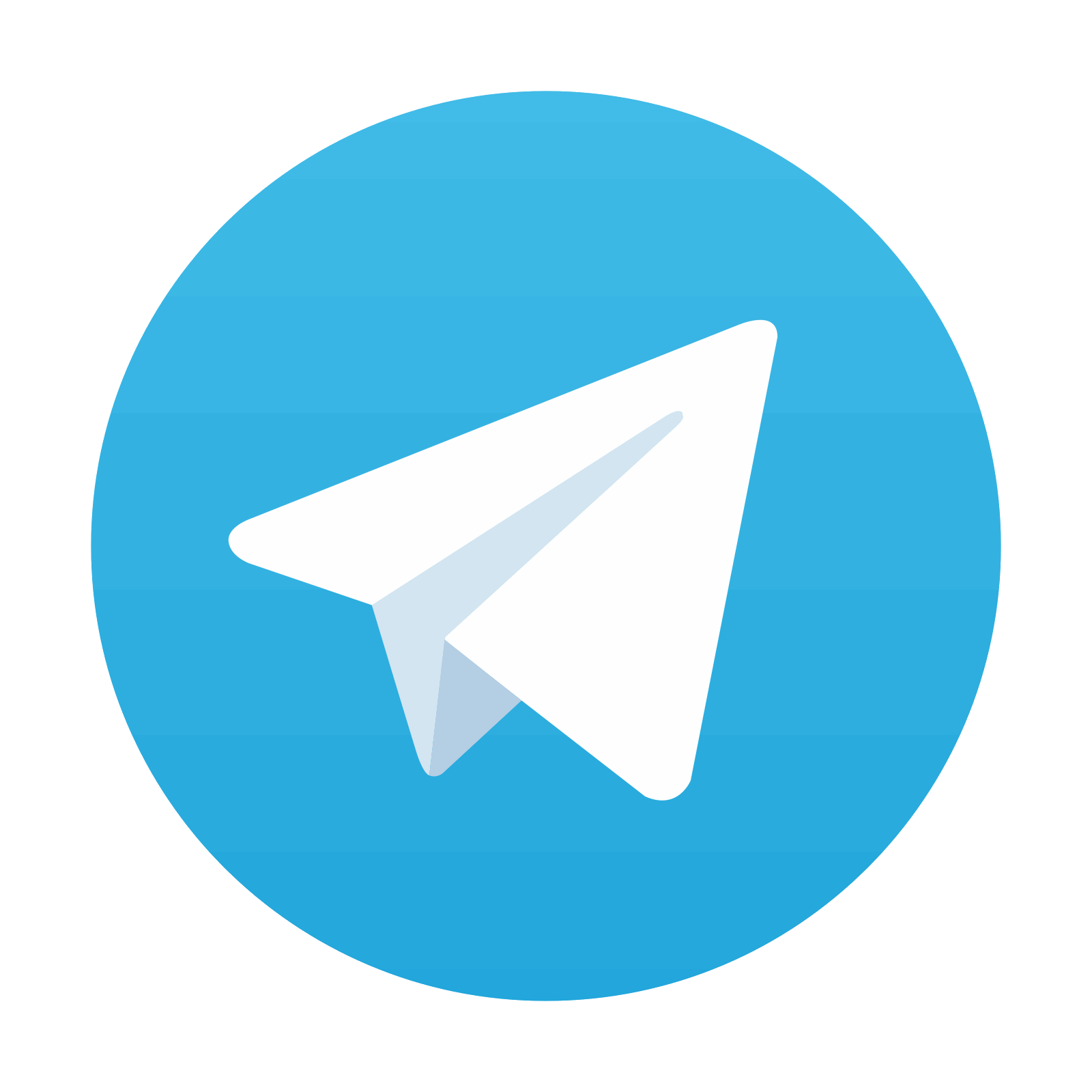
Stay updated, free articles. Join our Telegram channel

Full access? Get Clinical Tree
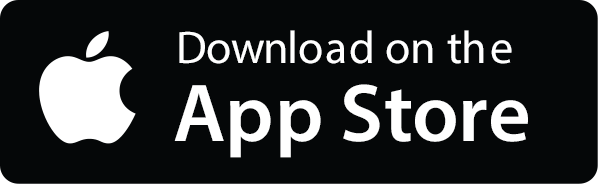
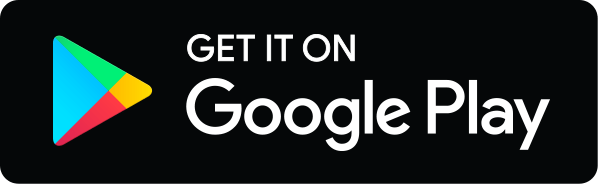