Altered Fractionation Schedules
This chapter discusses radiotherapy fractionation schedules that are different from the conventional fractionation in the United States of 1.8- to 2-Gy doses given once daily, Monday through Friday. The first section addresses background radiobiology. The second section reports outcomes of clinical studies. New results of pure hypofractionated radiotherapy for prostate and breast cancer have been added to this edition. Hypofractionated radiation schedules with highly conformal stereotactic body radiotherapy techniques or brachytherapy are discussed elsewhere in this book. Regimens are classified as either hyperfractionated or accelerated. In hyperfractionation, the total dose is increased, the size of dose per fraction is significantly reduced, the number of dose fractions is increased, and overall time is relatively unchanged. In accelerated fractionation, overall time is significantly reduced.
BACKGROUND RADIOBIOLOGY
Perhaps the most important consequence of altering a fractionation schedule is the sensitive change in late effects as the dose per fraction changes.1 Acute reactions are more sensitive to changes in the rate of dose accumulation.
The classic descriptions of early and late reactions are couched in terms of target cell killing. This characterization is on firmer ground with acute effects, for which direct connections can be made between depletion of identified cell populations and measurable injury, than with late effects, for which such identification is more problematic2 (see also Chapter 2 of this book). Despite this shortcoming, the conventional understanding of the potential advantages of alternative fractionation strategies is framed within the target cell concept.
Time–Dose Parameters
The time–dose parameters that determine normal tissue tolerance are total dose, overall duration of treatment, size of dose per fraction, and frequency of dose fractions. The last two determine the rate of dose accumulation, sometimes referred to as the weekly dose rate. The intensity of acute reactions in epithelial and other tissues organized into stem cell, maturation, and functional compartments (e.g., bone marrow) reflects the balance between the rate of cell killing by irradiation and the rate of regeneration of surviving stem cells. This balance depends primarily on the rate of dose accumulation. The fraction size is also a factor in determining the severity of acute reactions (large fractions being more damaging gray unit–for–gray unit than small ones) but to a lesser extent than is the case for late reactions. After an acute reaction has peaked (e.g., moist desquamation of the skin or confluent mucositis of the mucosa has occurred), further stem cell killing cannot produce an increase in intensity of the acute reaction but manifests as an increased time to heal the reaction. If sufficient stem cells do not survive to repopulate tissues, acute reactions may progress into a consequential late injury.3
The conventional view is that late reactions occur in tissues characterized by slow cellular turnover, such as mature connective tissues and the parenchymal cells of various organs. Because cellular depletion in such tissues does not manifest until after a typical course of radiation therapy is completed, the rate of dose accumulation and overall duration of treatment would be of minor significance in determining the severity of late reactions. Therefore, late reactions would depend primarily on total dose, size of dose per fraction, and interfraction interval.
There are difficulties with this simplified description. For example, there is evidence that the frequency of some late reactions correlates with the level of acute reactions, possibly through an influence of the rate of dose accumulation.4–5,6 In addition, there is evidence that late effects can be modified by pharmacologic intervention. For example, amifostine administration protects lung tissue and the esophageal mucosa in the treatment of lung cancer,7 and other agents have been shown to affect the development of radiation-induced nephritis (captopril) and fibrosis (pentoxifylline and vitamin E).
Size of Dose per Fraction and Length of Interfraction Interval
The influence of fraction size on radiation therapy outcome is manifest through the slope of the response to multifractionated doses, and this is a reflection of the repair capacity of the target cells. The experimental literature was reviewed,8 and the results shown in Figure 2.18 (Chapter 2) indicate that changes in isoeffect doses for late effects with changing dose per fraction (solid curves) are steeper than for acute effects (dashed curves). The significance of this is that if tumors are similar to acutely responding normal tissues in their sensitivity to changing fraction size, then a gain in the therapeutic ratio can be realized by significantly reducing the fraction sizes and escalating the total dose (hyperfractionation). These results are independent of any mathematical models and rest instead on the data shown in Figure 2.18. It is, however, convenient to be able to quantify the fractionation sensitivity, and this is most easily done using the linear-quadratic (LQ) model, assuming that the target cell hypothesis is correct and that the LQ model correctly describes the target-cell survival curves. Given these conditions, the ratio α/β of the parameters of the LQ model is a quantitative measure of this sensitivity to changes in fraction size8: low ratios signify high fractionation sensitivity, and high ratios signify low fractionation sensitivity. Low ratios imply relatively large changes in isoeffective dose when dose per fraction is changed, and the converse is true for high values. The implication is that the tolerance dose for late effects can be increased more by the use of smaller fraction sizes than the tolerance dose for tumors and acute effects (hyperfractionation). The α/β ratios for some animal normal tissues are set out in Table 2.1 in Chapter 2 and for human tissues and tumors in Table 12.1.
In general, the estimated values of α /β for early and late reactions in human normal tissues are consistent with results from experimental animals. With regard to tumors, squamous cell carcinomas of the head and neck, cervix, and skin and non–small cell lung cancers (NSCLCs) are characterized by high α/β ratios, in agreement with rodent models. However, data from melanomas and liposarcomas suggest somewhat lower α/β ratios for these tumor types. The α/β ratio for breast adenocarcinomas may be lower than those for other carcinomas listed in Table 12.1.9 The situation is different with prostate tumors, which contain unusually small fractions of cycling cells.10 Prostate tumors might not respond to changes in fractionation in the same way as other cancers11,12 and respond to changes in fractionation more like a late-responding normal tissue. In mathematical terms, the α/β ratio for prostate cancer is low, in the range 1 to 3 Gy, which is comparable to that for late sequelae.13–16 The radiobiology of prostate cancer appears to favor large fractions, with evidence emerging from hypofractionated high-dose-rate (HDR) brachytherapy.17
The arguments presented here really relate to the α/β value for prostate cancer in relation to the α/β value for the relevant late-responding normal tissue. There is good evidence both from animal11,18–22 and from human5,23,24–25 studies that for late rectal sequelae a value of α/β of >4 Gy is higher than for most other late sequelae. If the α/β value for prostate cancer is actually less than that for the surrounding late-responding normal tissue, hypofractionation (by external beam or HDR) at the appropriate dose would be expected to yield increased tumor control for a given level of late complications or decreased late complications for a given level of tumor control.
Whereas hypofractionation in a curative setting may result in unacceptable late effects,26,27 reports of hypofractionation for prostate cancer reveal minimal long-term urologic or bowel morbidity even with the much poorer dose distributions than are now routine, such as a 6-fraction 6-Gy protocol from London28 and a 15-fraction 3.1-Gy protocol from the Christie Hospital, Manchester, United Kingdom.16 Clinical trials of prostate cancer hypofractionation have been started using intensity-modulated radiation therapy (IMRT). In those studies that have reported on potential late sequelae, there is little indication of any unexpected late sequelae after median follow-up periods of 31,29 48,30 66,31 68,32 and 97 months.28 Early results of randomized trials of prostate hypofractionation are given in the second section of this chapter and Table 12.5.
TABLE 12.1 ESTIMATES OF α/β FOR HUMAN TISSUES AND TUMORS
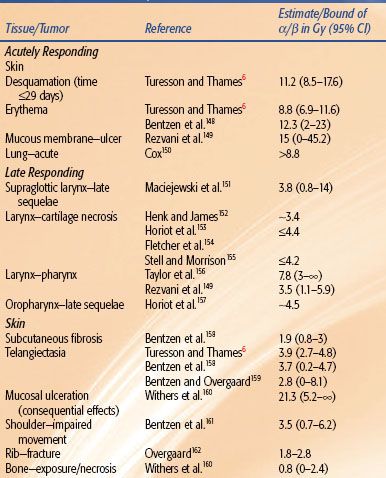
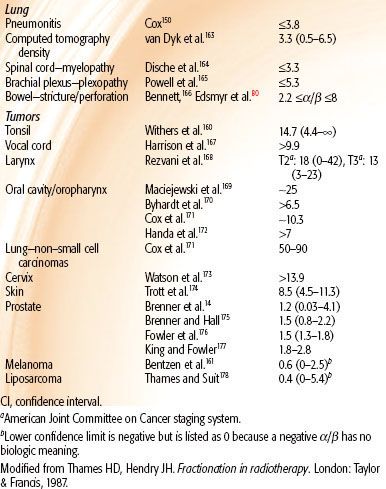
Repair Kinetics
To realize an increase in tolerance of late-responding tissues through dose fractionation, the time interval between the dose fractions needs to be adequate to allow repair to approach completion. If doses are too closely spaced, injury will accumulate between dose fractions, and successive doses will become increasingly more damaging. This emphasizes the importance of repair kinetics, which is quantified by the half-time for repair. Of the tissues in experimental models in which repair kinetics have been studied, half-times for repair tend to be longest (1 to several hours) in the skin, kidney, and spinal cord, shortest (approximately 1/2 hour) in the jejunal mucosa, and intermediate in the lung and colon.2,33–34,35–42 The exact values vary according to the experimental protocol, and considerable overlap exists in the confidence limits of repair half-time. The important point is to ensure an adequate interfraction interval during hyperfractionation.
Repair kinetics is of particular importance in determining the response of the spinal cord to fractionation schedules of more than one daily fraction. In rats, experimental data showed that repair is best described by a biexponential function in which the slower component has a half-time of 3.8 hours.43 This would imply that any fractionation schedule using more than one fraction per day is associated with some degree of incomplete repair in the spinal cord. A clinical report of radiation myelopathy occurring in four patients whose spinal cords received 45 to 48 Gy in 28 fractions of 1.5 Gy three times a day with a 6-hour interval over 9 consecutive days supports this observation,44 although incomplete repair cannot fully account for the observed frequency of injury.45 Two reports from the Radiation Therapy Oncology Group (RTOG)46,47 showed an increased rate of other late complications in patients treated on hyperfractionated protocols when the mean interval was <4.5 hours. For clinical practice, it is prudent to account for the potential compounding effect of incomplete repair. A minimum 6-hour interval between dose fractions is adequate.48
Data on repair kinetics in human normal tissues are extremely sparse, but, as indicated earlier, some evidence suggests that interfraction recovery may be slower in humans than in rodents. Bentzen et al.49 analyzed late complications in the Continuous, Hyperfractionated, Accelerated Radiation Therapy (CHART) randomized trial, and their findings are in agreement with this picture. Estimated repair half-times, with 95% confidence intervals, were 4.9 hours (3.2, 6.4) for laryngeal edema, 3.8 hours (2.5, 4.6) for skin telangiectasia, and 4.4 hours (3.8, 4.9) for subcutaneous fibrosis. These results are consistent with observations from two other randomized altered fractionation trials: European Organization for Research and Treatment of Cancer (EORTC) 22791 and EORTC 22851.50,51 Six hours should be regarded as the minimum interfraction interval for twice-daily fractionation.
Overall Time
The intensity of acute reactions is determined primarily by the rate of dose accumulation (weekly dose rate). Acute reactions represent a deficit in the balance between the rate of cell killing by radiation and cell regeneration from surviving stem cells. After the stem cell population is depleted the acute reaction peaks, and further depopulation produces no apparent increase in severity of the reaction. This means that the peak intensity of acute reactions is influenced more by the rate of dose accumulation than by the total dose, after a certain threshold of total dose has been reached.
Conversely, the time taken to heal depends on total dose, provided that the weekly dose rate exceeds the regenerative ability of the surviving stem cells. This is because healing is a function of the absolute number of stem cells surviving the course of treatment, and the higher the total dose, the lower is the number of stem cells surviving. Although most classic late radiation sequelae (e.g., spinal cord injury) show little or no dependence on overall time, overall time may be of significance for another set of late effects: consequential late effects that are attributed to severe and prolonged epithelial denudation rather than to direct radiation injury of the mesenchymal tissues normally associated with late reactions.3,52–55
The cure rates of many cancers (particularly squamous cell carcinomas) are also highly dependent on overall treatment time, with decrease in tumor control with longer treatment times. This has been interpreted in terms of accelerated regeneration of tumor clonogens.56 After a variable lag period, surviving tumor clonogens regenerate rapidly during fractionated radiation therapy to the extent that each additional day of treatment requires approximately 0.6 Gy, on average, to offset clonogenic cell regeneration, again suggesting a clonogenic cell doubling time of 3.5 to 5 days. This is illustrated in Figure 2.6 in Chapter 2.57–59
Other evidence has been adduced for accelerated regeneration of surviving tumor cells after therapeutic intervention.60 The majority of recurrences of squamous cell carcinomas of the head and neck occur within 2 years of treatment.61 This would have required approximately 30 volume doublings of nonsterilized tumor clonogens, and the median doubling time must have been about 6 days.43 Comparison of split-course treatment with continuous-course treatment suggests that 0.5 Gy per day is required to compensate for treatment interruption. Assuming that 2 to 3 Gy in 2-Gy fractions is necessary to reduce the surviving fractions of clonogenic cells by 50%, four to six doublings must occur during the 3-week treatment split, yielding a clonogenic cell doubling time of 3.5 to 5 days. Therefore, after initiation of radiotherapy, surviving clonogens in squamous cell carcinomas of the head and neck can regenerate with doubling times as short as 3 to 5 days.
Conversely, reduction of the overall treatment time may increase the probability of local tumor control. Molecular marker profiles (TP53, E-cadherin, KI-67, and EGFR) may aid in the selection of patients likely to benefit from reduction in overall treatment time.62
Isoeffect Formulas
The effect of changes of dose fractionation schedule on the total dose required to produce a certain level of biologic effect is approximated by isoeffect curves or formulas. The first clinical isoeffect curve was produced by Strandqvist.63 This was followed by other studies,64–66,67 culminating in the nominal standard dose formula of Ellis68: D = N0.24 × T0.11, where D is the dose, N is the number of dose fractions, and T is the overall time. None of these explicitly included dose per fraction.
In the early 1980s, it was pointed out that the different exponents for early and late effects could be interpreted in terms of survival curves for different target cell populations8 in which the curves for late effects were “curvier” than those for acute effects. The consequence of this is that isoeffect doses for various late effects in normal tissues are more sensitive to changes in dose per fraction than are corresponding doses for acute effects. With the widespread use of the LQ model to quantify the fractionation sensitivity, the following model has gained in popularity: D1 = D2(α/β + d2)/(α/β + d1), where D2 is the reference total dose given in fractions of size d2, and it is desired to calculate the total dose D1 in fractions of size d1 that would be isoeffective. This basic formula assumes complete repair between dose fractions, and no time factor is incorporated in it (see Chapter 2 for a detailed discussion). Thus, the basic formula may be used only when dose fractions are spaced widely enough apart to ensure complete repair and when either the endpoint is time independent (as with most late reactions) or the two schedules being compared involve the same overall time–dosing intensity.
Whereas the LQ-based isoeffect model is internally consistent for a wide range of tissue types and endpoints, clinical application of the model for derivation of new fractionation schedules is limited by at least two factors. First, there is the lack of precision of estimates of α/β. Even in closely controlled animal systems, estimates of α/β show large confidence intervals (Table 2.1 in Chapter 2). The α/β ratios of human data are consistent with the experimentally determined α/β ratios but have very wide confidence bounds (see Table 12.1). Second, as discussed earlier, the severity of complications can be modulated by various agents, including growth factors, radioprotectors, and pharmacologic agents, and current isoeffect concepts will doubtlessly have to be modified as the results of future studies become available. The bottom line is that no isoeffect formula is sufficiently reliable to preempt clinical judgment, and, in the final analysis, each new fractionation schedule must be tested clinically to establish its safety.
Rationale for Hyperfractionation
The basic rationale of hyperfractionation is that the use of small-dose fractions allows higher total doses to be administered within the tolerance of late-responding normal tissues, and this translates into a higher biologically effective dose to the tumor. For this rationale to hold, the α/β ratio for tumor cells must be greater than that for the dose-limiting normal tissue. Acutely responding tissues as a class have higher α/β ratios than late-responding normal tissues. Because of the kinetic similarity between tumors and acutely responding normal tissues, it may be predicted that tumors (with possible exceptions, such as the prostate) also tend to have large α/β ratios. Other rationales for hyperfractionation are radiosensitization through redistribution and lesser dependence on oxygen effect. The greater the number of dose fractions, the greater is the chance that cells would be in a more radiosensitive phase at the time of the next fraction. With small fractional doses, the influence of tumor cell hypoxia is reduced on two counts. First, the proportion of hypoxic cells needs to be higher to increase significantly the surviving fraction and, second, the oxygen enhancement ratio is lower.69
Hyperfractionation has been tested in more than 20 randomized trials, and these are summarized in the data presented in Table 12.2.
Rationale for Accelerated Fractionation
The rationale for accelerated fractionation is that reduction in overall treatment time decreases the opportunity for tumor cell regeneration during treatment and therefore increases the probability of tumor control for a given total dose. Because overall treatment time has little influence on the probability of late normal tissue injury, a therapeutic gain should be realized, provided the size of dose per fraction is not increased and the interval between dose fractions is sufficient for complete repair to take place.
When the overall duration of treatment is markedly reduced, it is necessary to reduce total dose to prevent excessively severe acute reactions. A therapeutic gain is then realized only if the reduction in dose is less than the dose equivalent of blocked regeneration of tumor cells due to shortened time.
Strategies to accelerate radiation can be divided into two categories: (a) pure accelerated fractionation regimens, with reduced overall treatment time without concurrent changes in the fraction size or total dose (examples are given in Table 12.3); and (b) hybrid accelerated fractionation, with reduced overall treatment time in conjunction with changes in other parameter(s), such as the fraction size, total dose, and time distribution. Four forms of hybrid accelerated fractionation were designed, and the regimes tested in randomized clinical trials are given in Table 12.4. The categories are further described as follows (Fig. 12.3):
Type A: There is drastic reduction of the overall time, with substantial decrease in the total dose.
Type B: Duration of treatment is more modestly reduced, with total dose kept in the same range, and there is a break in treatment.
Type C: Duration of treatment is more modestly reduced, with total dose kept in the same range, with a concomitant boost phase.
CLINICAL STUDIES
This section summarizes the results of hyperfractionated (HF) radiotherapy trials and accelerated fractionation (AF) radiotherapy trials. A new subsection summarizes results of pure hypofractionated radiotherapy (fraction size of >2 Gy). There is also a summary of combined altered fractionation with concurrent chemotherapy; critical fractionation issues to consider when IMRT is used; common clinically practiced fractionation schedules; and future directions in combining molecular targeting with altered fractionation. Hypofractionated radiation schedules with highly conformal stereotactic body radiotherapy techniques or brachytherapy are discussed elsewhere in this book.
TABLE 12.2 DATA OF PHASE III CLINICAL TRIALS ADDRESSING HYPERFRACTIONATION
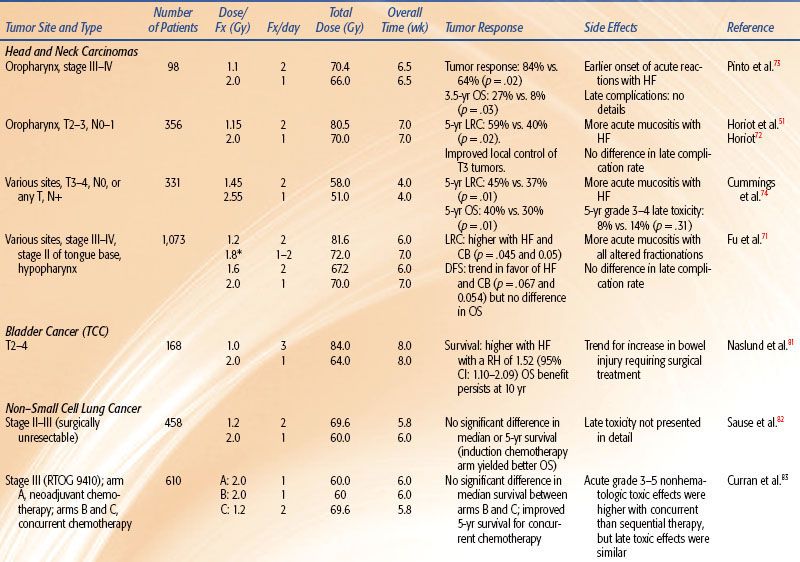
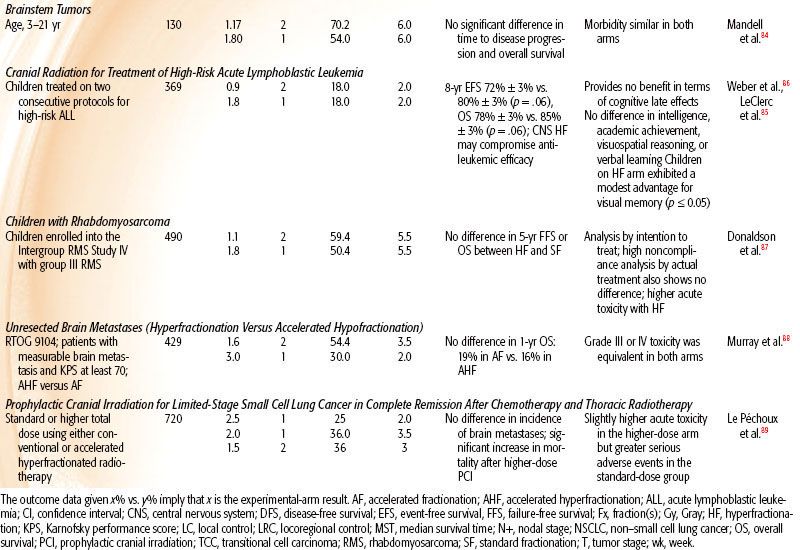
TABLE 12.3 DATA OF PHASE III CLINICAL TRIALS ADDRESSING PURE ACCELERATED FRACTIONATION
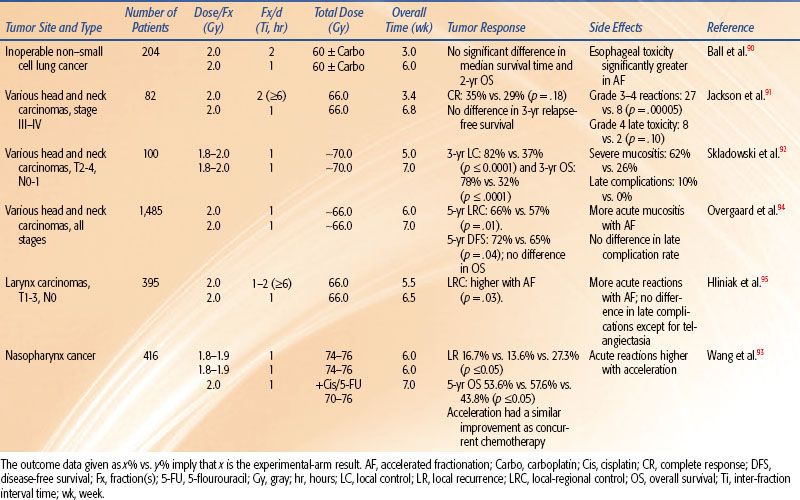
Results of Hyperfractionated Radiotherapy Trials
The key findings of hyperfractionated radiotherapy include the following:
• HF is better than standard fractionation in locoregional control of intermediate to locally advanced head and neck carcinoma. This was also associated with an improvement in survival in three trials.56
• Reducing the fraction size from 2 Gy to 1.1 to 1.2 Gy permits a 7% to 17% total radiation dose escalation without increase in late complications. This supports the existence of differential fractionation sensitivity (variable α/β ratios) between human late-responding normal tissues and head and neck carcinomas.
• There is significant survival benefit at 5 years with hyperfractionated radiotherapy on meta-analysis versus 2% with accelerated radiotherapy for head and neck cancer.
Altered fractionation radiotherapy improves survival in patients with head and neck squamous cell carcinoma.70 Comparison of the different types of altered radiotherapy suggests that hyperfractionation provides the greatest benefit. An individual patient data meta-analysis was conducted to see the effect of altered fractionation radiotherapy. It revealed a significant absolute survival benefit of 3.4% at 5 years with altered fractionation radiotherapy (hazard ratio [HR] = 0.92, 95% confidence interval [CI] = 0.86 to 0.97; p = .003) among 6515 patients with nonmetastatic head and neck squamous cell carcinomas in 15 randomized trials comparing conventional radiotherapy with altered fractionation. Significant survival benefit at 5 years with hyperfractionated radiotherapy was 8%, with accelerated radiotherapy was 2% (without total dose reduction), and was 1.7% (with total dose reduction; p = .02). Locoregional control was better with altered fractionation (6.4% at 5 years; p < .0001), with particularly effect improved local control and less benefit for nodal control. The benefit was significantly higher in the youngest patients, <50 years old.
Table 12.2 summarizes reported prospective, randomized trials addressing hyperfractionation for the treatment of patients with head and neck, bladder, lung, and brainstem tumors, whole-brain radiotherapy for pediatric acute lymphoblastic leukemia, whole-brain radiotherapy for brain metastases or prophylactic whole-brain radiotherapy, and rhabdomyosarcoma. The most striking results are from trials in head and neck squamous cell carcinoma (HNSCC), for which hyperfractionation was accompanied by an increase in dose.
TABLE 12.4 DATA OF PHASE III CLINICAL TRIALS ADDRESSING HYBRID ACCELERATED FRACTIONATION
FIGURE 12.1. The importance of survival curve shoulder shape rather than width for the response to fractionated irradiation. (1) Two survival curves with the same D0, Dq, and n but with different initial slopes and shoulder curvatures. In terms of the linear quadratic model of cell survival, the α/β ratio is lower for curve A than for curve B. (2, 3) Effect of change in fraction size on the dose required for a given effect. (2) When the shoulder has a steep initial slope and little curvature (high α/β), a change in dose per fraction from 3 to 1.5 Gy would only slightly increase the total dose needed to produce a given survival fraction. (3) When the shoulder has a shallow initial slope and marked curvature (low α/β), a much greater increase in total dose is necessary to produce a given survival fraction when the same change in dose per fraction is made. (From Peters LJ, Brock WA, Travis EL. Radiation biology at clinically relevant fractions. In: DeVita V, Hellman S, Rosenberg SA, eds. Important advances in oncology. Philadelphia: JB Lippincott, 1991:65–83.)
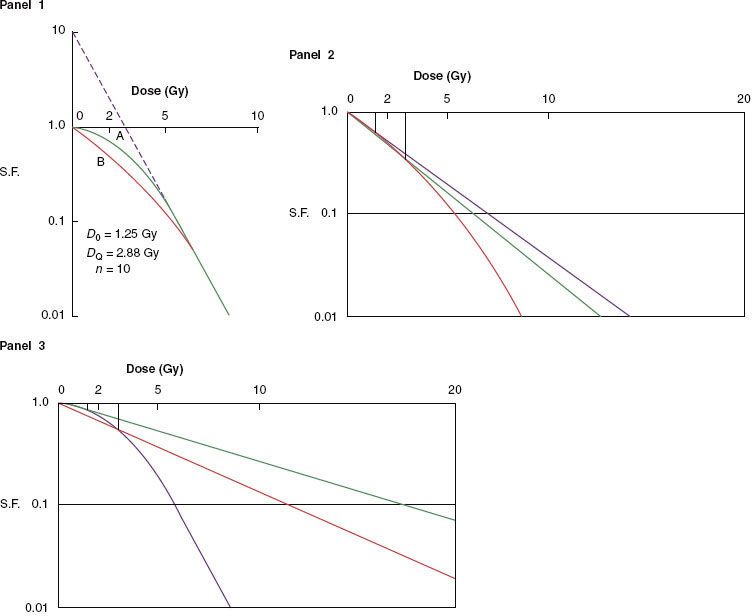
FIGURE 12.2. Effect of change in size of dose fraction (with overall time held constant) on the total dose necessary to produce a given level of acute and late effects. The curves are normalized to the “conventional” 2 Gy per fraction. Changes in fraction size have a relatively greater effect on the isoeffective doses for late reactions than for acute reactions and for the response of tumors with high α/β ratios. Consequently, by reducing the dose per fraction—for example, from 2 to 1.2 Gy—the total dose for equivalent late effects can be increased from O to A, which is greater than the increase (O to B) required to achieve an equivalent tumor response. The increment in dose from B to A represents the therapeutic differential achieved by hyperfractionation.
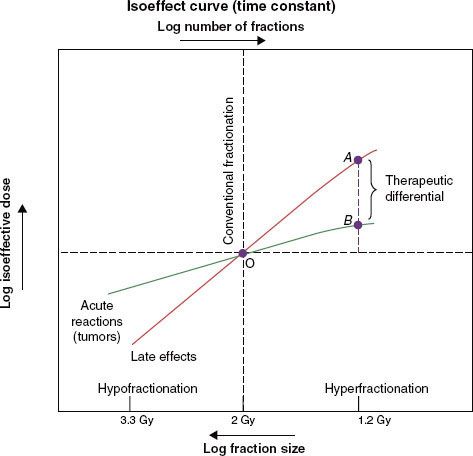
FIGURE 12.3. Conventional and accelerated fractionation schedules. For each regimen, the large-field treatment is depicted by the bars above the horizontal line and the boost-field irradiation by the bars below the line. The dotted bars represent treatment omitted in the lower ranges of total dose. fx, fraction.
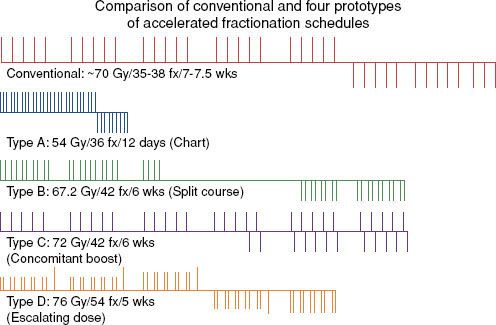
Head and Neck
In all four head and neck trials51,71–74 HF allowed a higher total dose to be delivered, which produced improved locoregional control by 8% to 20%. In three of these trials, HF improved overall survival by 10% to 19%. In all four studies, HF produced more severe acute mucositis but no increase in late morbidity. A Brazilian Group73 tested HF of 70.4 Gy at 1.1 Gy twice daily and showed improved local response by 20% and 3.5-year overall survival from 8% to 27%. The EORTC tested 80.5 Gy HF at 1.15 Gy per fraction twice per day for 7 weeks.51 The 10-Gy increase in dose improved locoregional control from 38% to 56% and overall survival.57,72 The Princess Margaret Hospital (Toronto, Canada) tested 58 Gy at 1.45 Gy twice per day over 4 weeks versus 51 Gy at 2.55 Gy per fraction once daily (a standard fractionation at that institute).74 The 7-Gy increase in dose improved locoregional control from 37% to 45% and improved 5-year overall survival from 30% to 40%.
The RTOG 9003 trial71 tested 81.6 Gy at 1.2 Gy per fraction HF twice per day over 6 weeks versus 70 Gy at 2 Gy per fraction versus two AF regimes (a continuous and a split-course accelerated regime), as shown in Tables 12.2 and 12.4. HF improved locoregional control from 46% to 54.4% (similar to the improvement by AF with the concomitant boost arm of the trial). This trial gives strong evidence that total dose and treatment duration are important to outcome. Locoregional control was significantly improved by an increase of the total dose without changing overall time using HF or by accelerated overall treatment time without changing total dose using concomitant boost fractionation.
Meta-analysis of hyperfractionated and accelerated radiotherapy in unresected locally advanced squamous cell carcinoma of the head and neck showed a substantial prolongation of median survival (14.2 months; p < .001) for hyperfractionated compared to conventional radiotherapy.75 Of the four hyperfractionation trials,51,71,73 one from Barcelona76 has been criticized for questionable quality.77 All four trials showed a statistically significant survival benefit. Studies testing HF without dose escalation did not show a survival advantage.78,79 HF is a useful tool to enable dose escalation without an increase of severe late toxicity.
Bladder Cancer
In the study by Edsmyr et al.,80 T2–4 bladder tumors were randomized to either three 1-Gy daily fractions 4 hours apart with interfraction intervals to 84 Gy, or a single daily fraction of 2 Gy to total dose of 64 Gy, both given in a split course over 8 weeks. Cystoscopic complete response rate increased from 36% to 65% by HF (p < .001), and the 5-year survival rate also significantly increased. However, severe late complications were higher in the hyperfractionated arm, indicating that the dose chosen might not be equivalent for late normal tissue injury. An updated analysis revealed that the survival benefit persisted for 10 years, but there was a trend for higher bowel complications requiring surgery in the HF group.81
Non–Small Cell Lung Cancer
The joint Intergroup trial82 of the RTOG, the Eastern Cooperative Oncology Group (ECOG), and the Southwest Oncology Group compared induction chemotherapy (cisplatin and vinblastine) plus 60 Gy in 2-Gy fractions or HF (69.6 Gy in 1.2-Gy fractions) with conventional fractionation (60 Gy in 2-Gy fractions). HF, although slightly better, did not significantly improve survival over the standard fractionation.
Two concurrent regimens with standard or hyperfractionated radiotherapy were tested as part of three arms of RTOG 9410, a phase III trial. Six hundred ten patients had either neoadjuvant cisplatin and vinblastine or the same chemotherapy concurrent with 60-Gy standard fractionation versus concurrent cisplatin and etoposide with hyperfractionated radiotherapy with 69.6 Gy delivered as 1.2-Gy twice-daily fractions. Median survival times were 14.6, 17.0, and 15.6 months, respectively. Five-year survival was statistically significantly higher for patients treated with the concurrent regimen with once-daily radiotherapy (TRT) compared with the sequential treatment (5-year survival: sequential, arm 1, 10% [20 patients], 95% CI = 7% to 15%; concurrent, arm 2, 16% [31 patients], 95% CI = 11% to 22%, p = .046; concurrent, arm 3, 13% [22 patients], 95% CI = 9% to 18%). Late toxic effects were similar.83
Childhood Brainstem Tumor
The trial of the Pediatric Oncology Group84 compared the efficacy of 70.2 Gy given as 1.17 Gy per fraction versus 54 Gy in 1.8-Gy fractions, both in combination with 100 mg/m2 cisplatin. This study showed no difference in the median time to disease progression, median time to death, or survival rates at 1 and 2 years, with no significant difference in toxicity.
Cranial Radiation Therapy for High-Risk Acute Lymphoblastic Leukemia
To test whether hyperfractionated (twice daily) therapy can reduce incidence and severity of late toxicities associated with 18-Gy prophylactic cranial radiotherapy, 369 children on two consecutive Dana-Farber Cancer Institute Consortium protocols were randomized to 18 Gy delivered in ten 1.8-Gy fractions once daily over 2 weeks versus 18 Gy delivered in twenty 0.9-Gy fractions. No benefit was seen in terms of cognitive late effects, and the results suggested that HF may compromise antileukemic efficacy.85,86
Group III Rhabdomyosarcoma
A study of 490 children with group III rhabdomyosarcoma (RMS) who were randomized to hyperfractionated radiotherapy (HFRT; 59.4 Gy in fifty-four 1.1-Gy twice-daily fractions) versus conventionally fractionated radiotherapy (CFRT) to 50.4 Gy in the Intergroup RMS Study IV showed that HFRT did not improve local/regional control, failure-free survival, or overall survival compared with CFRT and that HFRT actually produced more acute toxicity.87 Unlike studies in HNSCC showing positive results with dose-escalated HFRT, an escalation of dose by 9.5 Gy did not improve outcome for RMS.
Unresected Brain Metastasis
RTOG 9104 compared 1-year survival and acute toxicity rates between an accelerated hyperfractionated radiotherapy (1.6 Gy twice a day) to a total dose of 54.4 Gy and an accelerated hypofractionated arm of 30 Gy in 10 daily fractions in patients with unresected brain metastasis. Of 429 analyzable patients, the median survival time was 4.5 months in both arms. The 1-year survival rate was 19% in the hypofractionated arm versus 16% in the hyperfractionated arm. Grade III or IV toxicity was equivalent in both arms. In spite of an escalated dose, HF was of no benefit.88
Prophylactic Cranial Irradiation for Limited-Stage Small Cell Lung Cancer
To test whether higher-dose prophylactic cranial irradiation (PCI) would reduce brain metastases, 720 patients with limited-stage small cell lung cancer (SCLC) who were in complete remission after chemotherapy and thoracic radiotherapy were randomized to standard 25 Gy in 10 daily versus 36 Gy either as 18 daily 2-Gy fractions or accelerated hyperfractionated as 24 twice-daily 1.5-Gy fractions in 16 days. There was no significant difference in the 2-year incidence of brain metastases and a lower overall survival in the higher-dose group. PCI at 25 Gy was recommended as the standard of care in limited-stage SCLC.89
Results of Accelerated Radiotherapy Trials
Key findings of AF regimens include the following:
• Modest acceleration by 1 week by delivering six fractions of 2 Gy per week or a concomitant boost regimen without dose reduction or treatment break yields superior locoregional control of head and neck carcinomas without increase in late toxicity but without clear impact on survival. Acceleration by more than 3 weeks with a 10% total dose reduction (<6 to 7 Gy) also improves the locoregional control without demonstrable increase in late complications. However, a further 5% to 8% total dose reduction abrogates the gain in tumor control but appears to reduce the severity of some late normal tissue complications, such as fibrosis and edema.
• Mucositis per se or its consequential late toxicity prevents delivery of more than 12 Gy per week when given in two fractions of 2 Gy per day for 5 days a week or daily fractions throughout weekends to a total dose of 66 to 70 Gy (pure acceleration).
• Acceleration achieves significantly improved local control for well-differentiated tumors and advanced primary mucosal site tumors but may be of little benefit to advanced nodal disease and poorly differentiated tumors. This supports the existence of the accelerated proliferation phenomenon in mucosa-derived tumor cells.
The trials are classified into either pure accelerated, with same total dose, or hybrid accelerated, of which there are three types: (a) accelerated with dose reduction, (b) accelerated with split course, and (c) accelerated with concomitant boost.
Pure Accelerated Fractionation
Table 12.3 summarizes the radiation regimens and outcomes of six reported randomized trials of pure AF for the treatment of patients with NSCLC lung and head and neck carcinoma.
Non–Small Cell Lung Cancer
A study of 204 patients randomized them to 60 Gy in 3 weeks versus 60 Gy in 6 weeks, both with and without concurrent carboplatin.90 The results showed no survival advantage with either AF or concurrent carboplatin. The study showed that 60 Gy in 3 weeks induced a significantly greater esophagitis than did 60 Gy in 6 weeks.
FIGURE 12.4. Zone of therapeutic gain in squamous cell carcinomas of the head and neck for different accelerated fractionation schedules. Type A is 54 Gy in 36 fractions over 12 days, type B is 67.2 Gy in 42 fractions over 42 days, and type C is 72 Gy in 42 fractions over 40 days. For explanation, see text. (From Peters LJ, Brock WA, Travis EL. Radiation biology at clinically relevant fractions. In: DeVita V, Hellman S, Rosenberg SA, eds. Important advances in oncology. Philadelphia: JB Lippincott, 1991:65–83.)
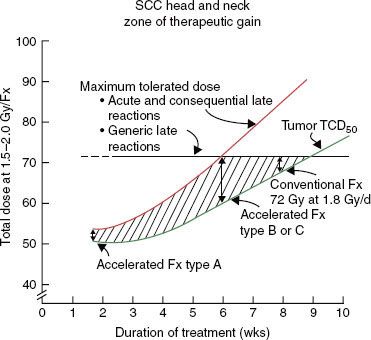
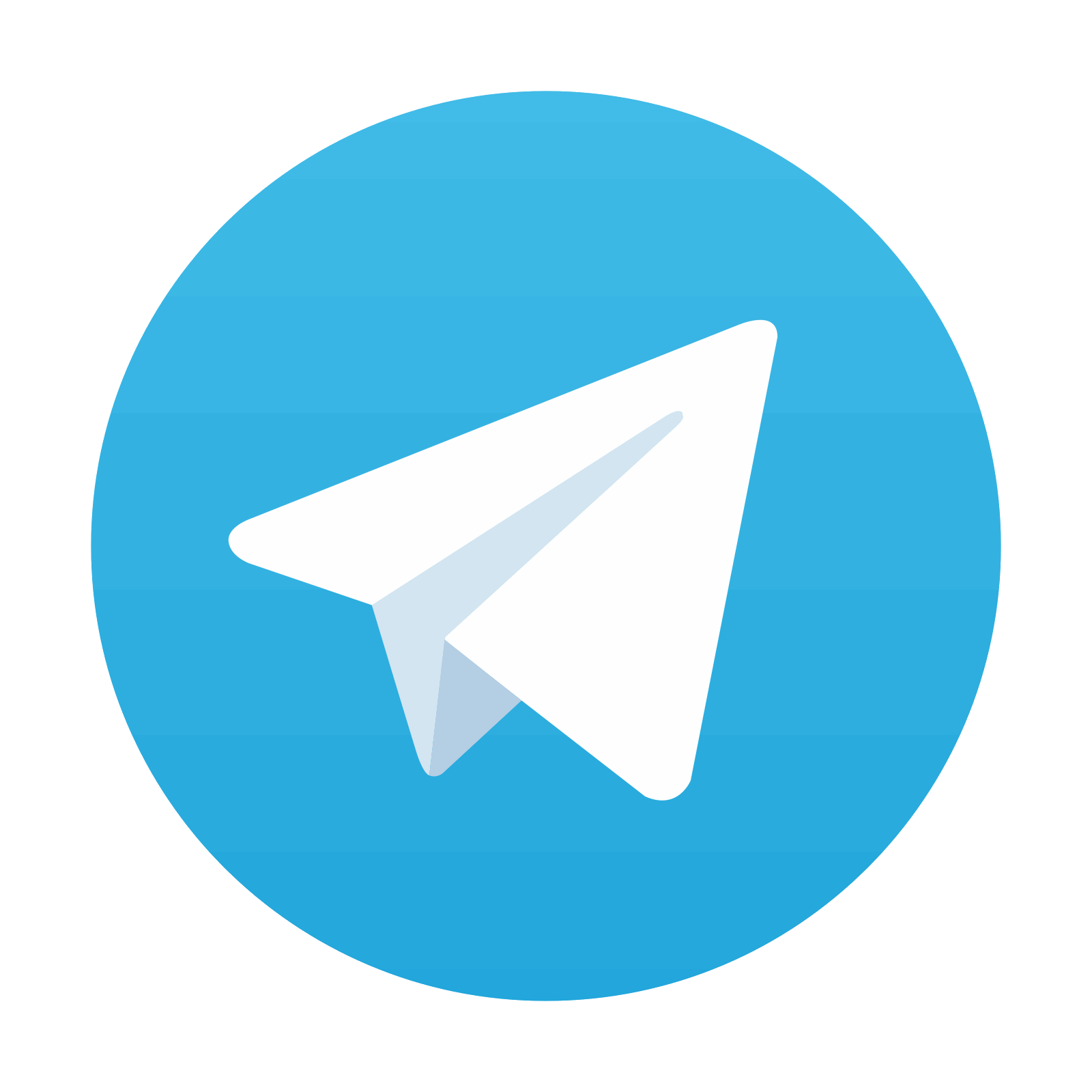