AGE-RELATED CHANGES IN PHYSIOLOGY AND FUNCTION
PEARLS
❖ Not all body cells age in the same way or at the same rate; however, in general, the total number of cells in the body decreases with age while the remaining cells become less alike in structure and less organized in function.
❖ Cardiovascular cellular changes with age are numerous and range from lipofuscin accumulation at the poles of the nuclei of cardiac muscle to increased calcification in the media.
❖ In the pulmonary system, age changes can be organized according to mechanical properties, changes in flow, gas exchange, and impairment of lung defense.
❖ The decrease in aging muscle is associated with a selective loss of type II muscle fibers, a decrease in protein and nitrogen, and an increase in connective tissue and fat.
❖ Due to changes in the thermal regulatory response, older adults have prolonged cooling time following increased activity as well as a decrease of receptiveness to heat and cold.
❖ All 5 senses as well as proprioception/kinesthesia decline with age.
❖ The endocrine system plays a major role in the biological variability of aging.
The aging process occurs along the continuum of life. Beginning at conception and terminating in death, certain changes are recognized as transitional markers in the human aging process. Aging is viewed as characteristically decremental in nature and lacking in defined chronological points of transition although linear with time.1 The notion of a “maximal achievable life span” is inherent in the concepts of aging.2–4 Identifying and describing changes in function that are common to all individuals and not produced by pathology are very difficult tasks. The use of the term eugeric distinguishes changes related to the natural aging process from changes related to pathology.5 The hypothesis of eugeric death is that the decline of function continues linearly to the point where an internal environment compatible with cell life can no longer be maintained.
Although the process of aging is very complex and does not uniformly result in decreased functional capacity,6 this survey of the body’s systems will be based on the assumption that the losses associated with the aging process are due to declines in cellular functioning. The kidney is an excellent example of this—function is diminished in proportion to the number of nephrons lost. Skeletal muscle is another example. While muscle mass decreases secondary to the loss of muscle fibers, the remaining muscle mass is capable of oxygenation and consuming metabolic substrates at a constant rate. The most obvious aging changes are those in which function is totally lost; the capacity of the woman to reproduce is an excellent example, while another would be the loss in ability to hear sounds above a certain frequency.
Homeostasis is a concept describing the “constancy of the internal environment.”7 This internal constancy enables humans to survive in many environments and to withstand many biological and physiological challenges, thus expanding the habitable world of the human. Perhaps the single most salient and age-related difference is the diminishing ability of the body to respond to physical and emotional stress and return to the prestress level.8,9 This decrease in homeostatic capacity is observed in all of the systems of the body but is most marked in neuroendocrine interaction and the systemic functional alteration in the responsiveness of the nervous and endocrine systems.7
The changes associated with aging through adulthood into old age are gradual and dependent on exercise, nutrition, stress management, sleep, and the multitude of factors affecting the way we age. During adult life, in the absence of overt pathology, there is a slow decrement in function. Homeostasis can be maintained, albeit at a lower level. Another observation is that the more complex the function, the more decline is seen. There are important differences between the young and old when considering interacting systems. Decrements are greater in the functions involving a number of connections between nerve and nerve, nerve and muscle, and nerve and gland. For instance, the decrease in nerve conduction velocity is less than the decrease in the maximum breathing capacity. In the former, a single system is involved, whereas in the latter the coordination of a number of nerve and muscle activities is required.
Finally, it is important to keep in mind that individuals age at different rates. Different tissues and systems within one person demonstrate different aging rates as well. Therefore, although it is useful to discuss the average declines of function, it is important to keep in mind that any one individual may show remarkable variability from his or her peers.1
CARDIOPULMONARY CHANGES WITH AGE
Cardiovascular
Generally, there is a decrease in cardiac output at rest, a decline in the cardiovascular system’s response with stress, an increase in systolic blood pressure, and a progressive increase in peripheral vascular resistance to blood flow. A consistent myofibrillar change documented has been lipofuscin accumulation at the poles of the nuclei of cardiac muscle cells.10 Baker et al11 found that cardiac muscle cell diameter and protein production within the nuclear region of the cell were significantly increased with age. A marked decline in the total number of pacemaker cells at the sinoatrial node occurs at the approximate age of 60, and by the age of 75 there are less than 10% of pacemaker cells compared with the adult heart.11,12 It has also been found that there is an increase in interstitial fibrous tissue, specifically in the internodal tracts.11
Heart rate, loading conditions, intrinsic muscle performance, and neurohumoral efficiency all affect cardiac output. The maximum heart rate achievable declines linearly with age at peak exercise levels. Heart rate response is usually not affected at submaximal exercise levels.10,11 Heart rate response has been demonstrated to diminish in response to various physiological stimuli, such as coughing, postural changes, or during the Valsalva maneuver.12 The rate with which the heart rate peaks also becomes prolonged with increasing age.10 Cardiac filling and vascular impedance are loading conditions that influence cardiac output. The diastolic filling rate has been shown to decrease with age,13 and this is attributed to prolonged isometric relaxation; thickening and sclerosis of the mitral valve, which impedes ventricular filling; and an age-associated decrease in left ventricular compliance.13 Vascular impedance is affected by central aortic stiffness or compliance, peripheral vascular resistance, and the inertial properties of blood.12 Systolic pressure in the aorta and pulse pressures are increased with age.14 Late systolic pressure exceeds early systolic pressure in older adults, and the index of elastance (characteristic impedance) and peripheral resistance are increased with age.14 These changes are indicative of less aortic compliance and a reduced cross-section of the peripheral vasculature, which cause increases in pulse wave velocity and wave reflection. It is hypothesized that there is a consequent increase in vascular load, which could explain the age-associated reductions in stroke volume and cardiac output as well as the development of mild left ventricular hypertrophy and prolonged myocardial relaxation in older adults.14
Intrinsic muscle performance is estimated by the mean velocity of circumferential fiber shortening. This does not change with age; however, isometric relaxation and early diastolic relaxation are prolonged as age increases.15 Left ventricular wall thickness may result from prolonged relaxation.14
Cardiac output is also affected by neurohumoral regulation. In the myocardium and the peripheral vessels, end-organ responsiveness is decreased to β-adrenergic stimulation.16 The autonomic tone is increased based on plasma catecholamine levels. R eflex activity of the cardiovascular system (ie, Valsalva maneuver, orthostasis, and cough) is weaker in the older cardiovascular system due in part to decreased baroreceptor sensitivity and changes in the cardiopulmonary system.11,13,16
The ability of the body to maintain a homeostatic blood pressure is a function of the autonomic nervous system, the arterial baroreceptor reflex, circulating neurohumoral factors, the local vascular tone, and the extracellular fluid volume. There is an alteration in the homeostatic blood pressure regulatory role as a result of an altered autonomic nervous system with age.16 An elevation of blood pressure in the older adult can be attributed to an increase in plasma norepinephrine levels leading to an increase in sympathetic responsiveness.14 The functioning of the arterial baroreceptor reflex is lessened with age.11,16 Atherosclerosis and the presence of hypertension also decrease the baroreceptor reflex. Catecholamines and circulating neurohumoral factors assist in the maintenance of homeostatic blood pressure. These factors include renin, aldosterone, vasopressin, atrial natriuretic factor, and angiotensin II. The level of renin in the plasma has been found to remain unchanged or decrease in relation to age.16 It is hypothesized that this is due to a decrease in the concentration of active renin and is not a result of the concentration of renin substrate in the plasma. In proportion to the decreased renin activity in the plasma, there is a related decline in aldosterone and plasma angiotensin II levels with increasing age.16 Vasopressin remains unchanged or decreases with age. A decrease in vasopressin activity is most often related to blood loss or dehydration.13 The level of plasma atrial natriuretic factor does not show a change with age. Vascular tone may show a local increase with age although the constriction of the vessels is not changed in response to norepinephrine with age.12
The effect of these changes on the cardiovascular system is seen in changes in cardiac output, stroke volume, and blood pressure at rest and in response to stress. Resting heart rate does not show a consistent age-related change in humans.11 Resting cardiac rate and cardiac output remain relatively unchanged, although peripheral resistance (blood pressure) is increased.10 With age, myocardial weight tends to increase, and myocardial cells show an increase in lipofuscin deposition. M itochondria decrease in size, and myocardial cells are less responsive to catecholamine stimulation. A reduction in baroreceptor response and vascular elasticity combines to result in the tendency for older people to experience postural hypotension.7,10,14 When sitting at rest, cardiac output does not show a change with age; however, in the supine position, there is often an age-related decrease in cardiac output.11 There is also a similar position-associated change in stroke volume. This is explained by the changes with age in cardiac compliance and preload conditions. Resting blood pressures, both diastolic and systolic, tend to show an increase with age. It is not clear whether this increase in blood pressure is a reflection of eugeric aging, the result of heredity and/or environmental factors, or both.14
In response to stress, the cardiovascular system shows decreases in heart rate acceleration and ejection fraction with physical exertion. Postural changes also affect cardiac output and blood pressure as previously discussed. Following a moderately sized meal, older adults tend to show a decrease in systemic blood pressure.10 With increasing levels of exercise, there is a concomitant rise in systemic blood pressure. This rise is credited to the changes in preload conditions during cardiac filling. In the absence of pathology, the cardiovascular responses to increased activity levels described are consistently seen with increasing age; however, aerobic conditioning exercises may alter this. The effects of exercise on the cardiovascular system are positive and will be discussed in a subsequent chapter.
Pulmonary
In this chapter, aging changes defined by Lum et al as those changes that are “universal, intrinsic, progressive, and irreversible” within the cardiopulmonary system are discussed.17 The pathologies of the lung are discussed further in Chapter 5.
In the pulmonary system, age changes can be organized according to mechanical properties, changes in flow, changes in volume, alteration in gas exchange, and impairments of lung defense.18 Decreases in chest wall compliance and lung elastic recoil tendency are 2 mechanical properties that are altered with age. Increased calcification of the ribs, a decline in intercostal muscle strength, and changes in the spinal curvature all result in a lower compliance and increased work of breathing.17
At normal lung volume, airway resistance is not increased; however, normal aging results in a reduction of maximum voluntary ventilation, maximum expiratory flow, and forced expiratory volume in 1 second (alone and in relation to forced vital capacity). Although tidal volume remains fairly constant throughout life, vital capacity decreases, while residual volume increases.17
Ventilation, diffusion, and pulmonary circulation are the 3 major components of the respiratory system that lose efficiency with age.19 There is an increased thickening of the supporting membranes between the alveoli and the capillaries, a decline in total lung capacity, an increase in residual volume, a reduced vital capacity, and a decrease in the resiliency of the lungs. It is difficult to completely separate pulmonary changes as a result of aging from those associated with the pathology of emphysema or chronic bronchitis.18 Throughout a lifetime, exposure to occupational and environmental inhalants as well as cigarette smoke may result in chronic pulmonary changes and lung pathologies. These disease states closely parallel those of the aging process and also increase in incidence with advancing age.20 Normal pulmonary aging includes a loss of elastic tissue leading to expiratory collapse of the larger airways, difficulty with expiration, and dilatation of the terminal air passages.21
There are changes in the diffusion efficiency of the peripheral vascular system with age. Starting with the pulmonary system, impairment of gas exchange is illustrated by a reduced diffusing capacity of carbon monoxide, a lower resting arterial oxygen tension, and an increased alveolar-arterial oxygen gradient. The alveolar surface area and pulmonary capillary blood volume diminish with age. Small changes in red blood cell metabolism produce a decrease in 2,3-diphosphoglycerate. As a result, the oxygen dissociation curve shifts to the left, which makes oxygen less available at the tissue level.22
The ability to provide oxygen to working tissues is altered because normal aging affects the cardiopulmonary system in a variety of ways. In the absence of pathology, the heart and lungs can generally meet the body’s needs; however, reserve capacities are diminished. With any challenge, the body’s demand for oxygen and perfusion may exceed the available supply.
In an older person, normal changes result in an impairment of pulmonary defenses. Cilia are reduced in number, and those that remain become less strong. The mucus escalator and alveolar macrophages are less effective in removing inhaled particulate matter. In the absence of physiological challenges, the system maintains fairly adequate defenses. However, an older individual who is chronically exposed to air laden with particles will become at risk for pulmonary dysfunction.7,22
Cardiopulmonary Changes With Age and Therapeutic Considerations
The recovery period following effort is prolonged in older adults. Among other factors, this reflects a greater relative work rate, an increased proportion of anaerobic metabolism, slower heat elimination, and a lower level of physical fitness. During exercise, older individuals need to lengthen the cooldown phase of exercise to allow for a more gradual return to baseline vital signs. Abrupt cessation of exercise without considering an adequate recovery period could have negative effects for a person of any age, but it is particularly important in older adults.23
MUSCULOSKELETAL CHANGES WITH AGE
Cartilage
Connective tissue affected by aging includes bone, hyaline cartilage, elastic cartilage, and articular cartilage. Changes in bone are presented separately.
Hyaline cartilage is found in the nose and the rings of the respiratory passages as well as the joints. Elastic cartilage is found in parts of the larynx and the outer ear. Articular cartilage is found between the intervertebral discs, between the bones of the pelvic girdle, and at most articular joint surfaces.24 With aging, cartilage tends to dehydrate, becomes stiffer, and thins in weightbearing areas.
Cartilage is formed when the primitive mesenchymal cells are subjected to compressive forces in an environment of low oxygen concentration. The predominant secretions of the chondroblasts are glycoprotein, chondroitin sulfate, and hyaluronic acid. Collagen is produced in lesser amounts. Cartilage is a unique connective tissue in that it has no direct blood supply. Blood flow in adjacent bones and synovial fluid provide nutrients to the chondroblasts. A strong osmotic force created by glycoprotein secretions from the chondroblasts passing from the cells into the surrounding matrix attracts water with dissolved gases, inorganic salts, and organic materials into the matrix, providing materials necessary for normal metabolism. The concentration of glycoproteins in the matrix determines the amount of fluid drawn into the cartilage. Normal aging is accompanied by a reduction in the amount of chondroitin sulfate produced, resulting in a decrease in osmotic attraction forces and impairment in the ability of the matrix to attract and retain fluids.25
Nutrients enter the matrix of the cartilage only when compressive forces are absent.25 In a loaded or compressed state, fluid and nutrient substances are squeezed out. To provide regular movement of substances in and out of the cartilage, it is necessary that alternating application and release of compressive forces occur. Metabolites remain in the matrix in the absence of compression. The presence of metabolites reduces the oxygen content, resulting in a reduction of the secretion of glycoproteins and an increase in the amount of procollagen produced. With inactivity, hyaline cartilage is converted to fibrocartilage.25 Therefore, weightbearing exercises become particularly important in the older adult. The movement of nutritional substances in and out of the cartilage with activity could enhance the overall health of the cartilage, preserving the viability of the joints.
In synovial joints, the articular surfaces are covered by hyaline cartilage. Lubrication at the interface of the hyaline cartilage is provided by the secretion of hyaluronic acid by the chondroblasts. Hyaluronic acid molecules form a viscous layer covering the hyaline cartilage. Compression facilitates the production of hyaluronic acid, ensuring continual lubrication of the joint during movement. With the decrease in the secretion of hyaluronic acid with age, there is a reduction in the efficiency of the lubrication system of the joint.25 Degenerative changes of the cartilage are not reversible, and rehabilitation efforts need to be directed toward regular compression and release of compression in the aging joint. Normal weightbearing exercises are recommended to maintain cartilaginous health.
The cartilage that normally covers body joints thins and deteriorates with aging, especially in weightbearing areas. Because cartilage has no blood supply or nerves, erosion within the joint is often advanced before symptoms of pain, crepitation, and limitation of movement are perceived. Decreased hydration, reduced elasticity, and increased fibrous growth around bony prominences all contribute to increased stiffness and decreased function. Progressive cartilage joint deterioration leads to osteoarthritis in the affected joint(s).
Muscle
Sarcopenia is the loss of muscle mass and strength that occurs with aging resulting from a decrease in activity at the neuromuscular junction. It is a consequence of normal aging and is not necessarily related to disease, although muscle loss can be accelerated by chronic illness. Muscle wasting occurs in all humans, although it usually goes unnoticed. Sarcopenia results in muscle weakness, reduced activity level, increased prevalence of falls, morbidity, and loss of functional autonomy. It is the major cause of disability and frailty in older adults.26 There are many candidate mechanisms leading to sarcopenia, including age-related declines in alpha motor neurons, growth hormone production, sex steroid levels, and physical activity. Age-related decreases in growth hormone, insulin-like growth factor 1 and 2, estrogen, testosterone, and dehydroepiandrosterone and its sulfates play a major role in sarcopenia.27,28 In addition, fat gain, increased catabolic cytokines, and inadequate intake of dietary energy and protein are also potentially important causes of sarcopenia. The relative contribution of each of these factors is not yet clear. Sarcopenia can be reversed with high-intensity progressive resistive exercise, which can also slow its development. A major challenge in preventing an epidemic of sarcopenia-induced frailty in the future is developing public health screenings and interventions that deliver an anabolic stimulus to the muscle of older adults.
Skeletal
The skeletal system functions to support, protect, and shape the body. Additionally, bone has the metabolic functions of blood cell production, the storage of calcium, and a role in acid-base balance.
Musculoskeletal Changes With Age and Therapeutic Considerations
The most commonly known age-related change involving bone is calcium-related loss of mass and density. This loss ultimately causes the pathological condition of osteoporosis. Bone density is lost from within by a process termed reabsorption. As we grow older, an imbalance occurs between osteoblast activity (bone buildup) and osteoclast activity (bone breakdown). Osteoclast activity proves to be the stronger. As one ages, a decline in circulating levels of activated vitamin D3 occurs.29 This causes less calcium to be absorbed from the gut and more calcium to be absorbed from the bones to meet body needs. In postmenopausal women, decreased estrogen levels influence parathormone and calcitonin to increase bone reabsorption, which decreases bone mass. Certain factors such as immobility, decreased estrogens, steroid therapy, and hyperthyroidism, to name a few, are known to accelerate bone erosion to pathological levels. Easily occurring fractures are the most common result.30
Osteoporosis is a critical disorder in the older adult because it decreases the bone mineral content, and as a result, bone mass and strength decline with age. It is difficult to draw the line between what is normal and what is pathological in osteoporosis. Bone loss appears to be a normal aging process and has been characterized by decreased bone mineral composition, an enlarged medullary cavity, normal mineral composition, and biochemical abnormalities in plasma and urine. The rate of bone loss is about 1% per year for women starting at age 30 to 35 and for men at age 50 to 55. In older persons, regions of devitalized tissue with osteocyte lacunae and Haversian canals containing amorphous mineral deposits have been described.7 These have been identified as micropetrotic regions and are noted to increase in frequency in the skeleton with age. Thus, it is clear that the mineral content of bone qualitatively changes with age.30
Qualitatively, osteoporotic bone exhibits a reduction in bone mass with a resulting decrease in bone strength, and there is some evidence that alterations occur in the composition and structure of bone in older adults. Tensile strength of bone in humans is related to the number and size of osteons. It has been found that bone from older humans has smaller osteons and fragments and more cement lines than younger bone. This would account for some of the reduced bone strength of the older bone specimens. The remaining difference in strength results from the geometric structure of the bone in its distribution per unit area as a response to environmental stress placed on the bone. A more comprehensive discussion of osteoporosis is provided in Chapter 5.
Throughout life, red blood cells continue to be replaced after a life span of about 120 days. Some morphologic changes do occur with aging. Red cells are slightly smaller and more fragile. However, blood volume is well maintained until approximately 80 years of age. In the absence of pathology, few changes are seen in the white blood cells and the platelet count. What is lost with aging is the functional reserve to quickly accelerate the production of red blood cells when needed.7
NEUROLOGICAL CHANGES WITH AGE
Quantitatively, all muscle measures, including biological, anatomical, and physiological, decline after the age of 40.31 Many of the changes associated with aging indicate a decrease in the number of active functional units (ie, motor units or muscle fibers) and a loss in concentration of specific enzymes or fiber types.
Central Nervous System
After peaking in the early decades of life, brain mass or weight slowly decreases by as much as 6% to 7% by the time a person reaches 80 years old. Although the brainstem appears to be minimally affected by cell loss, widely varied but significant losses occur in the cerebral cortex lobes and cerebellar area. Central nervous system cells are postmitotic. The central neurons that remain continue to decline in numbers and efficiency of function.32
Cell number and composition both decrease. With aging, cells of the hippocampus undergo a degeneration caused by numbers of vacuoles surrounding dense central granules. Amyloid plaques develop, and lipofuscin is deposited within many remaining neuronal cells. After age 60, the number of neuronal microtubular structures may decrease and are often replaced by so-called neurofibrillary tangles.33 Although plaques and tangles occur with normal aging, they are most commonly associated with the occurrence of senile dementia of the Alzheimer type.
Impulse conduction and cerebral synaptic transmission are both delayed with aging, affecting the transmitter competence of the central nervous system. A particular explanation lies in the general decline of available neurotransmitters. Serotonin, catecholamines, and gamma-aminobutyric acid are less prevalent in the older brain. A decrease in the neurotransmitter dopamine is found in normal older adults but is also associated with the pathology of Parkinson disease.34
Conduction velocity of the central nervous system has been shown to decrease with advanced age. A loss of the myelin sheath and a loss of large myelinated fibers decrease axion abilities to transmit impulses, especially in the posterior spinal column tracts. These tracts provide for positive righting reflex responses. Remembering that balance impairment partially results from cerebellar losses (now coupled with central nervous system delays), one can begin to see why an older person has a greater tendency to fall and less ability to quickly correct center of balance before injury occurs.35
Intersystem Homeostasis
Thermal Regulation
The role of the hypothalamus in homeostatic regulation is a major factor in age-related declines. With increasing age, the hypothalamus becomes less sensitive to physiological feedback and consequently is less able to maintain the stability of the internal environment of the body. Processes such as increased body weight, increased serum cholesterol, and decreased glucose tolerance ensue and, subsequently, diseases result.36 The hypothalamic thermostat is the principal control center for regulating the body’s response to ambient, locally applied, and internal temperature gradients. Many investigators have attributed the increased rate of heat stroke, hypothermia, and climate-related deaths among the older population to faulty thermoregulation.37
Not only the hypothalamic thermostat and basal metabolic rate but also the overall reactivity of the autonomic nervous system decline with age, altering skin hydration and circulation in turn.38 The vasomotor system is less responsive to warming and cooling, and the normal transient bursts of vasoconstrictor activity are reduced. It is unclear whether or not thermoreceptors in the skin are altered. Because cold receptors are dependent on a good oxygen supply, it may be reasoned that a decreased circulatory supply may decrease the perception of cold because of the vulnerability of cold receptors to hypoxia.38
Age-related changes in the thermal regulatory response have clinical significance in the older adult’s ability to maintain homeostasis with increasing exercise levels; the cooling time following exercise is often prolonged. In addition, a decrease in the receptiveness of temperature gradients impacts the application of heat and cold modalities in treatment interventions. Consideration of these changes needs to be employed when treating the older patient.
Hormonal Balance
Aging is marked by deterioration not only in the function of individual cells and organs but also by a failure of mechanisms for the coordination of function between various parts of the body.39 A weakening of both neural and hormonal controls reduces the ability to adjust to external and internal stresses. Among other responsibilities, the body hormones contribute to (1) the regulation of circulating fluid volumes and cardiovascular performance, (2) the mobilization of fuels for exercise (maintenance of blood glucose, liberation of fat, and breakdown of protein), and (3) the repair of body structures with the synthesis of new protein (anabolism). All of the changes in these functions impact exercise tolerance and the healing process in older adults. Older adults are slower to reach homeostasis during exercise, and the return to a balanced homeostatic state following exercise is prolonged. In addition, the healing process is slower due to a diminished synthesis of new protein.39
Circulation
A blood flow rate of approximately 40 mL/min/100 g brain tissue is regarded as minimally necessary to maintain adequate cerebral perfusion. Compared with the flow rate of 50 to 60 mL/min/g experienced with youth, an older person may have as much as a 20% reduction in cerebral perfusion by the age of 70. Although cerebral perfusion is adequate if the body is not challenged, in the presence of pathology (eg, arteriosclerosis or decreased cardiac output), the older person experiences an increased risk of cerebral damage.7
Peripheral Nervous System
Aging is often characterized by reduced sensibility, coordination, and cognitive abilities as well as a reduced ability to react to changing circumstances. A general assumption is made that the loss of nerve tissue (ie, reduced cell number) is a predominant feature of aging. In reality, although some loss of nerve cells does take place during the aging process, the extent to which this loss occurs is less than usually assumed. The reduced level of nervous system functioning in older adults is better explained in terms of biochemical changes that take place in neurons during aging and senescence.39
The ionic exchange across the nerve membrane to produce a nerve impulse is a relatively simple mechanism that is altered little during the aging process. In older adults, there is no significant change in conduction velocity along a specified portion of a nerve trunk compared with that found in younger adults. In the older adult, as in the younger person, if a reduction in conduction velocity is found, some narrowing of the fiber affecting the integrity of the nerve or some impairment of blood flow to the nerve sheath may be assumed.40
Sensory
In the body, information is gathered, interpreted, and transmitted through the integration of the neurosensory system, which includes the nervous system and each of the 5 senses (ie, touch, smell, taste, vision, and hearing). Each of these systems is highly complex, and structural changes are known to occur with aging. The sum total of these changes results in a decline of neurosensory function.41
Touch
Peripheral receptors are responsible for the sense of touch. As with the other senses, touch also declines with age. Specific receptors for touch, pressure, pain, and temperature are found within the dermis and epidermis of the skin. Receptors can be freestanding or arranged in small corpuscular masses. Meissner corpuscles (touch-texture receptors), Pacinian corpuscles (pressure-vibration receptors), and Krause corpuscles (temperature receptors) as well as peripheral nerve fibers are noted to decline. Although quantitative studies have produced inconclusive results, because free nerve endings remain relatively unchanged, the ability to sense pain should remain intact. The older adult must take special care to avoid injury from concentrated pressures or temperature on the skin (eg, pressures from shoes that are too tight, bath water that is too hot).42
The skin is a very important element in touch. In general, skin wrinkles increase with advancing age, but the directional change of epidermal thickness remains controversial. The dermis does thin, loses elasticity, and has diminished vascularity.41 Loss of tissue support for the remaining capillaries results in fragility and easy bruising (senile purpura). Although the tanning response diminishes, the appearance of flat pigmented lentigines (age spots) increases with exposure to the sun, and such exposure also increases the risk of neoplastic development from actinic keratosis. The previously reviewed decline in cellular division results in a slower rate and efficiency of tissue repair following any trauma.43
Changes in the dermal appendages, such as hair, also occur with age. The degree to which hair becomes gray is largely genetically determined, but a reduction in hair follicles produces a reduction of hair. In contrast, after menopause, facial hair tends to increase in women. Nails grow more slowly and develop longitudinal ridges. The number and size of sweat glands are diminished, resulting in a reduction of sweat production.43
Health care providers need to be sensitive to the fact that some of the following changes may affect the self-esteem of an older person. If this is the case, sensitive psychological support must be provided in any interaction.
Vision
Although humans are strongly visual creatures, the eye is vulnerable to many age-related changes. Externally, the eyelids show an increase in wrinkling and ptosis resulting from losses of elastic tissue, orbital fat, and a decrease in muscle tone. Very often, the older person will develop an entropion (a turning inward of the eyelid) or an ectropion (an outward relaxation of the eyelid), which is particularly apparent with the lower lids. Aging results in diminished tear production, and ocular inflammation or infection may occur in some older adults if supplementary artificial tears are not provided.41
Arcus senilis, a deposition of lipids around the outer edge of the cornea, is a well-known, age-related phenomenon that does not interfere with vision. Arcus senilis has been associated with hyperlipidemia in younger people. However, no such association has been shown in older adults.44
In addition to becoming smaller with age, the ocular pupil reacts more slowly to light, and the ability to focus quickly from far to near declines. This loss of accommodation is termed presbyopia. The ability to focus is dependent on the ability of the ocular lens to change shape as needed. Presbyopia is partially caused by a decline in ciliary muscle efficiency; however, as a person ages, the ocular lens continues to grow while becoming more dense and inelastic. Increased stiffness results in less flexibility and resultant decreased ability to change shapes and focus on desired objects. Far vision is more easily achieved because the ciliary muscles relax and allow the lens to thin. Near vision requires the ciliary muscles to contract to increase the thickness of the lens. This is why older people may require bifocals, which offer a less strong prescription for far vision and another, stronger one for reading or close vision. Along with reduced accommodation, older people experience a decreased ability to adapt comfortably and quickly to changes of light and dark. Many older people say they had to give up driving at night because of this age-related change.44
The older eye demonstrates a tendency toward increased intraocular pressure. As the lens continues to grow into the anterior chamber of the eye, the chamber becomes smaller, and the circulation of aqueous humor is reduced. Although the healthy older eye can tolerate this change, it is possible for a pathological glaucoma to occur concurrently. The increased density of the lens may lead to a form of cataract, which can result in a complete loss of useful vision. On a less serious note, the aqueous humor may also develop a yellowish pigmentation, creating difficulty in distinguishing between greens and blues.41,44
An older person may comment to you on the presence of floaters in his or her visual field. With aging, the vitreous body of the eye loses hydration and tends to demonstrate some clustering of collagen material.43 This clustering causes shadows or opacities to be projected on the retinal wall. Although the presence of these opacities is normal, an increase in number can be indicative of retinal hemorrhage or detachment.43,44
Age-related changes can ultimately produce a decline in visual acuity. Even when errors of aged refraction are corrected, a loss of visual receptors in the aging retina or macula will result in a decrease of acuity. Although there are some treatments, there is no cure. Fortunately, with modern technology, the majority of older people are able to maintain a high degree of visual function and independence.45
Hearing
Although hearing loss may develop at any age, hearing losses do occur more frequently in the older years. A sensorineural hearing loss, often called presbycusis, is most common in older adults. With a sensorineural loss, sound is well conducted through the external and middle ear, but age-related impairments of the inner ear or auditory nerve prevent sound transmission to the brain. Age-related changes that may contribute to sensorineural hearing loss include sclerotic changes in the tympanic membrane, cochlear otosclerosis, a loss of hair-like receptors in the organ of Corti, and degeneration of the auditory nerve.43,46
Presbycusis results in a decreased ability to hear and discriminate speech, particularly at higher and lower frequency levels. Because normal speech contains a broad range of frequencies, the older person may realize that he or she is being spoken to but may not understand all that is being said.46 Difficulties increase when the speaker talks too quickly or when the individual with hearing impairment is unable to observe the speaker’s face.
Contrary to common belief, a sensorineural hearing loss does not always preclude the use of a hearing aid. Vision should be corrected so the skill of visual speech conception can be used as much as possible. When speaking with an older adult with sensorineural loss, words should be spoken slowly in a medium-pitched voice, and face-to-face communication should always be maintained.
Proprioception/Kinesthesia
Proprioception or kinesthetic sense is provided by sensory nerves that give information concerning movements and position of the body. These receptors are located primarily in the muscles, tendons, and the labyrinth system.7 Although a greater degree of sensory perceptual loss results from local system changes (ie, impaired vision from increased lens density), cerebral cortex cell loss may result in less cellular availability for sensory interpretation.47 This is of great clinical importance in that, as one ages, there may be a concomitant loss of position and movement sense. Coupled with losses in the other sensory systems, this could significantly affect an older individual’s awareness of limb or body position affecting safety during transfers and ambulation. (See also Chapter 12.)
Vestibular System
The vestibular system changes during the aging process. Degeneration occurs in the sensory receptors in both the otoliths and semicircular canals. The function of the vestibular system is to monitor head position and to detect head movements.47,48 When an individual is deprived of visual and lower extremity somatosensory information, the vestibular system is left to provide sensation for the control of balance. Healthy young adults are able to balance without meaningful visual or support surface information. On the other hand, healthy older adults lose their balance and might even fall when vestibular input is the only spatial orientation information available.48 All of the major sources of orienting information are compromised during the aging process. Dehydration and diseases further compound this problem. The vestibular system is discussed in more detail in Chapter 12.
Neurological Changes With Age and Therapeutic Considerations
Taste and Smell
The senses of taste and smell become less acute with age. As much as 80% of taste buds may atrophy, and perception of taste sensation (ie, sweet, salty, bitter, and sour) becomes less sharp. A reduction of saliva flow occurs as a person ages and may aggravate an already-dulled sense of taste. The olfactory bulb demonstrates age-related cell losses, which seem associated with decreased perceptions of various smells. It is proposed that these declines contribute to the appetite decline that is observed in and experienced by the majority of older people.43
Deficits in these chemical senses not only reduce the pleasure and comfort of food but also represent risk factors for nutritional and immune deficiencies as well as adherence to specific dietary regimens. Chemosensory decrements can lead to food poisoning or overexposure to environmentally hazardous chemicals that are otherwise detectable by taste and smell.49
In summary, pathological processes superimposed on physiological aging comprise changes in the sensory system including visual, hearing, olfactory, taste, and somatosensory functions. Representative changes of age-related changes are presbyopia, other visual problems, and presbycusis. Deep sensation of vibration and proprioception is decreased with age as well as superficial sensation (touch, temperature, and pain). A reduced sense of smell is seen in normal aging, but the prominent reduction detected by the odor stick identification test is noticed especially in the early stages of Alzheimer or Parkinson disease. A reduced sense of taste is well known, especially in salty sense, whereas the changes of sweet, bitter, and sour tastes are different among individuals.49 As a result, an impaired sensory system could induce deterioration of the activities of daily living and quality of life in older adults.
INTERNAL ORGAN SYSTEM CHANGES WITH AGE
Gastrointestinal
The gastrointestinal tract is subject to many changes throughout life. Although normal aging is not responsible for all gastrointestinal changes, it is sometimes difficult to differentiate the effects of aging from those that result from a lifetime of poor habits involving hygiene, food, and substance abuse. Epidemiologic studies are beginning to more strongly implicate lifestyle in relation to some changes in the gut.43
Internal Organ System Changes With Age and Therapeutic Considerations
It is a fallacy to believe that teeth must be lost with aging. Improved dental hygiene and nutrition can prevent common pathologies of tooth loss such as dental caries and periodontal disease. However, with age, the tooth does lose masticating enameled surface area. Intermaxillary spaces decrease, and tooth pulp may atrophy and regress.43 If teeth are lost, the older person may experience a migration of the normally opposing teeth, with local oral trauma occurring as a result.
The older esophagus demonstrates a reduction of motility and a hesitance of the lower esophageal sphincter to relax with swallowing. To define these changes, the term presbyesophagus was coined.50 When eating, the older person may experience an often uncomfortable substernal sense of fullness as food entry into the stomach is delayed. In contrast, the lower esophageal resting pressure declines with age. This weakening allows gastric contents to more easily reflux into the lower areas of the esophagus, causing heartburn to occur. Hiatal hernias frequently develop in the older person who has a reduced resting pressure of the lower esophageal sphincter.51
An age-related reduction in motility also affects the stomach, colon, and probably the small intestine. The gastric emptying time often is delayed.52 Degeneration of gastric mucosa occurs in a small number of older adults and may cause a decrease of intrinsic factor, digestive enzymes, and hydrochloric acid. Usually, this atrophic gastritis is not the sole cause of B12 malabsorption and resulting pernicious anemia, but gastrointestinal digestion can be reduced. Medications activated by an acid gastric condition may be less effective in the more alkaline environment of an older stomach. Additionally, an older individual may interpret this gastric discomfort as acid indigestion and further diminish the available acid supply by taking over-the-counter antacids.
A reduced blood supply to the gut and a decrease in the number of absorbing cells can hinder nutrient absorption in the small intestine. Decreased motility in the colon causes older adults to have a tendency to develop constipation. If the older adult is particularly immobile or dehydrated, constipation can easily lead to the more serious conditions of fecal impaction and bowel obstruction. Diverticulitis is also common in older adults. However, its occurrence is probably more related to a diet low in fiber and high in refined, low-residue foods.7,51 More on the gastrointestinal changes with aging are covered in Chapter 6.
Renal, Hepatic, and Urinary
It is generally accepted that liver mass and blood perfusion both decline with aging. Metabolism of many drugs is decreased, and, following injury, regeneration of hepatic cells occurs more slowly. When compared with younger people, no significant differences are found in the serum indicators of liver status. These indicators include the measurement of bilirubin clearance, glutamic oxaloacetic transaminase, serum glutamic pyruvic transaminase, and alkaline phosphatase production. Although total serum protein remains relatively stable, reduced albumin to globulin ratios result in a decline of colloid osmotic pressure. Protein binding of medications may also be decreased. Alterations in protein binding and the prolongation of drug effects within the body are two of the more serious results of normal age changes in the liver.7,53
When discussing these systems, the gallbladder and pancreas should be mentioned because they also demonstrate functional changes with aging. For example, the incidence of biliary stones increases in older adults, which is probably related to a reduced efficiency of cholesterol stabilization in the body. Controversy exists over the reduction of pancreatic mass with age. A decline in mass may be hidden by an increase in pancreatic fat deposition. Pancreatic cells become less homogeneous, and studies have generally reported a decline in enzyme volume and concentration, although adequate amounts are available for normal digestive functions.43,54 Another important endocrine age change is the decreased ability of the peripheral tissues to use available insulin produced by the pancreas. However, the most important pancreatic age change is the decreased ability of the beta cells to increase insulin production in response to a challenge of increased blood glucose.54,55
The older kidney demonstrates both a loss of parenchymal mass and a reduction of total weight. By the time a person is 85 years old, the amount of remaining functioning nephrons may be decreased by as much as 30% to 40% of what was available in youth. Vascular changes, like a reduction in glomerular capillary loops and increased tortuosity of arcuate and interlobar arteries, have been reported. Renal perfusion declines as much as 50% by the later decades of life.43 The Bowman capsule basement membrane thickens, the glomerular filtration rate declines, and blood urea nitrogen tends to increase. The renal tubules show a decline in excretory and reabsorptive capacities, and a loss of urine-concentrating abilities occurs. Older kidneys can maintain acid-base homeostasis in an unchallenged environment; however, they are unable to handle increased loads of either acid or base. The structural changes observed in the normal aging kidney support the theory that one should expect a decline in renal function as a person ages. However, reports by renal physiologists suggest that this is not always true.55 The suggestion has been made that vascular adaptations to structural changes may help to preserve the glomerular filtration rate by producing a state of hyperperfusion and hyperfiltration in the surviving nephrons.55
The urinary bladder demonstrates an increased number of uninhibited contractions frequently associated with cerebral arteriosclerotic changes and overconcentration of the urine. Increases in residual urine and reflux into the ureters provide an ideal environment for bacterial growth. Both asymptomatic and symptomatic bacteriuria are common in older adults.7,56
Endocrine
Proper functioning of the endocrine system is essential to maintain the majority of the body’s regulatory processes. In some cases (eg, reproductive hormones), age-related changes are well known. In other cases, specific information is nonexistent or unclear. Much available information remains highly controversial.
Although age-related structural changes in the thyroid do occur, in the absence of pathology, its function tends to remain adequate for body needs. A decrease in the basal metabolic rate is shown in older people but seems related to the reduction of lean body mass. Older adults are at risk for both hypo- and hyperthyroid problems. However, these problems are unrelated to changes that occur with normal aging.43,57,58
Tests of adrenal function show plasma glucocorticoid levels to be similar in the young and old. The adrenal cortex response to adrenocorticotropic hormone (ACTH) remains intact as does the pituitary’s release of ACTH in response to stress. However, circulating levels of aldosterone do decrease with age.57,58
The pituitary gland demonstrates a reduction in vascularity and an increase in deposition of connective tissue.7 A reduction in mass is not well established. With aging, nocturnal elevations in growth hormone disappear. Serum concentrations of ACTH and growth hormone are unchanged with aging.57 In most older adults, thyroid-stimulating hormone remains normal although a small percentage develop a slight increase in thyroid-stimulating hormone without obvious symptoms. Postmenopausal women show an increase in follicle-stimulating hormone and luteinizing hormone.57
Changes in reproductive hormones are most dramatic in the older woman. Following menopause, estrogen and progesterone levels significantly decrease. Serum androgen levels remain relatively unchanged. In the older man, blood levels of testosterone probably decrease, but agreement on this decline has not yet been reached.57
The uterus, ovaries, and fallopian tubes of the older woman become dysfunctional with menopause and decrease in size. A reduction in estrogen causes the vagina to shrink, thin, and lose mucosal protection. The breasts, labia, and clitoris all lose subcutaneous mass. In advanced years, pubic hair is lost. In contrast, an older man will continue to produce sperm throughout his life. However, sperm production and counts are reduced, while sperm abnormalities are increased. The testes may demonstrate very little loss of weight, but fibrous tissue deposition increases in the intertubular spaces. With age, the seminiferous tubule basement membrane thickens. Age changes in the prostate begin around age 40 and continue into the older years. The most disruptive change involves the replacement of smooth prostatic tissue by dense connective tissue. As the connective tissue accumulates, resulting prostatic hypertrophy impinges on the urethra and interferes with smooth release of urine.7,58
CONCLUSION
Aging as a universal occurrence is regarded as a biological, anatomical, and physiological or normal process distinct from pathological processes. As much as aging might be influenced by a predisposition to disease, it is not considered abnormal. Conceptually, this distinction seems clear enough, but when applied to specific cases, the boundaries become blurred. Some degree of decline is noted in all biological, anatomical, physiological, and functional components of the human body with age and is not considered pathological. Aging has been excluded from the domain of disease because it is considered normal. Aging is viewed as the result of the accumulation of unrepaired injuries resulting from mostly unavoidable, universal changes. If one defines disease as a reaction to injury, then is there a distinct aging process? If a steady accumulation of microinjuries causes a linear decline of function, does the presence of redundancy in any system translate into a linear loss of functional capacity, and is aging an accelerated age-specific failure of each system? The distinction between aging and disease becomes one of arbitrary degree. A variety of degenerative processes are repeatedly termed normal aging until they proceed far enough to cause clinically significant disability and become disease. In such cases, the distinction between aging and disease is more semantic than biologically, anatomically, physiologically, or functionally normal. All vital organs begin to lose some function as you age during adulthood. Aging changes occur in all of the body’s cells, tissues, and organs, and these changes affect the functioning of all body systems.
REFERENCES
1. Gallo LA, Tran M, Moritz KM, Wlodek ME. Developmental programming: variations in early growth and adult disease. Clin Exp Pharmacol Physiol. 2013;40(11):795-802.
2. Last C. Human evolution, life history theory, and the end of biological reproduction. Curr Aging Sci. 2014;7(1):17-24.
3. Fries JF. Aging, natural death and the compression of morbidity. N Engl J Med. 1968;303:113-123.
4. Kent S. The evolution of longevity. Geriatrics. 1980;35:98-104.
5. Moskalev A, Chernyagina E, de Magalhaes JP, et al. Geroprotectors. org: a new, structured and curated database of current therapeutic interventions in aging and age-related disease. Aging (Albany NY). 2015;7(9):616-628.
6. Andres R. Normal aging versus disease in the elderly. In: Andres EL, Bierman EL, Hazzard WR, eds. Principles in Geriatric Medicine. New York, NY: McGraw-Hill; 1985:38-41.
7. Kenney RA. Physiology of aging. Clin Geriatr Med. 1985;1(1):37-59.
8. Rodriguez Manas L. Determinants of frailty and longevity: are they the same ones? Nestle Nutr Inst Workshop Ser. 2015;83:29-39.
9. Shock NW. Physiological theories of aging. In: Rockstein M, ed. Theoretical Aspects of Aging. New York, NY: Academic Press; 1974:119-136.
10. Wei JY. Cardiovascular anatomic and physiologic changes with age. Top Geriatr Rehabil. 1986;2:1:10-16.
11. Baker PB, Arn AR, Unverferth DV. Hypertrophic and degenerative changes in human hearts with aging. J Coll Cardiol. 1985;5:536A.
12. Hagstrom L, Henein MY, Karp K, Waldenstrom A, Lindgvist P. Impact of age and sex on normal left heart structure and function. Clin Physiol Funct Imaging. 2016;6(10):11111-11231.
13. Hoshida S, Shinoda Y, Ikeoka K, Fukuoka H, Inui H, Watanabe T. Age- and sex-related differences in diastolic function and cardiac dimensions in a hypertensive population. ESC Heart Fail. 2016;3(4):270-277.
14. Reddy AK, Jogendra MR, Rossendorff C. Blood pressure measurement in the geriatric population. Blood Press Monit. 2014;19(2):59-63.
15. Miyatake K, Okamoto M, Kinoshita N, et al. Augmentation of atrial contribution to left ventricular inflow with aging as assessed by intracardiac Doppler flowmetry. Am J Cardiol. 1984;53:586-589.
16. Frolkis W, Shevtchuck VG, Verkhratsky NS, Stupina AS, Karpova SM, Lakiza TY. Mechanisms of neurohumoral regulation of heart function in aging. Exp Aging Res. 1979;5(5):441-477.
17. Lum S, Stocks J, Stanojevic S, et al. Age and height dependence of lung clearance index and functional residual capacity. Eur Respir J. 2013;41(6):1371-1377.
18. Nowicka U, Wiatr E, Radzikowska E, et al. Pulmonary function abnormalities in regard to age at the time of diagnosis of hypersensitivity pneumonitis. Adv Exp Med Biol. 2015;861:75-84.
19. Davenport MH, Beaudin AE, Brown AD, Leigh R, Poulin MJ. Ventilatory responses to exercise and CO2 after menopause in healthy women: effects of age and fitness. Respir Physiol Neurobiol. 2012;184(1):1-8.
20. Zadai CC. Cardiopulmonary issues in the geriatric population: implications for rehabilitation. Top Geriatr Rehabil. 1986;2(1):1-9.
21. Verbanck S, Thompson BR, Schuermans D, et al. Ventilation heterogeneity in the acinar and conductive zones of the normal ageing lung. Thorax. 2012;67(9):789-795.
22. Lowery EM, Brubaker AL, Kuhlmann E, Kovacs EJ. The aging lung. Clin Interv Aging. 2013;8:1489-1496.
23. Wang E, Naess MS, Hoff J, et al. Exercise-training-induced changes in metabolic capacity with age: the role of central cardiovascular plasticity. Age (Dordr). 2014;36(2):665-676.
24. Hole JW. Human Anatomy and Physiology. Dubuque, IA: Wm C. Brown Co; 1988.
25. Walker J. Connective tissue plasticity: issues in histological and light microscopy studies of exercise and aging in articular cartilage. J Orthop Sports Phys Ther. 1991;14(5):189-197.
26. Roubenoff R. Sarcopenia: a major modifiable cause of frailty in the elderly. J Nutr Health Aging. 2000;4(3):140-142.
27. Tseng BS, Marsh DR, Hamilton MT, Booth FW. Strength and aerobic training attenuate muscle wasting and improve resistance to the development of disability with aging. J Gerontol. 1995;50:113-119.
28. Booth FW, Weeden SH, Tseng BS. Effect of aging on human skeletal muscle and motor function. Med Sci Sports Exerc. 1994;26(5):556-560.
29. Bidlack WR, Kirsch A, Meskin MS. Nutritional requirements of the elderly. Food Technol. 1988;40:61-70.
30. Turunen MJ, Prantner V, Jurvelin JS, Kroger H, Isaksson H. Composition and microarchitecture of human trabecular bone change with age and difference between anatomical locations. Bone. 2013;54(1):118-125.
31. McKinnon NB, Connelly DM, Rice CL, Hunter SW, Doherty TJ. Neuromuscular contributions to the age-related reduction in muscle power: mechanisms and potential role of high velocity power training. Ageing Res Rev. 2017;35:147-154.
32. Jang YC, Van Remmen H. Age-associated alterations of the neuromuscular junction. Exp Gerontol. 2011;46(2-3):193-198.
33. Kakimoto A, Ito S, Okada H, Nishizawa S, Minoshima S, Ouchi Y. Age-related sex-specific changes in brain metabolism and morphology. J Nucl Med. 2016;57(2):221-225.
34. Burchinsky SG. Neurotransmitter receptors in the central nervous system and aging: pharmacological aspects (review). Exp Aging. 1984;19:227-239.
35. Bohannon RW, Larkin PA, Cook AC, et al. Decrease in timed balance test scores with aging. Phys Ther. 1984;64:1067-1070.
36. Besdine RW, Harris TB. Alterations in body temperature (hypothermia and hyperthermia). In: Andres R, Bierman EL, Hazzard WR, eds. Principles in Geriatric Medicine. New York, NY: McGraw-Hill; 1985:209-217.
37. Asmussen E. Aging and exercise. In: Horvath SM, Yousef MK, eds. Environmental Physiology, Aging, Heat and Altitude. New York, NY: Elsevier/North Holland; 1981.
38. Ajiduah AO, Paolone AM, Wailgum TD, Irion G, Kendrick ZV. The effect of age on tolerance of thermal stress during exercise. Med Sci Sports Exerc. 1983;15:168.
39. DeCarli C, Kawas C, Morrison JH, Reuter-Lorenz PA, Sperling RA, Wright CB. Session II: mechanisms of age-related change and targets for intervention: neural circuits, networks, and plasticity. J Gerontol A Biol Sci Med Sci. 2012;67(7):747-753.
40. Grimby G. Physical activity and muscle training in the elderly. Acta Med Scand Suppl. 1986;711:233-237.
41. Corso JF. Sensory processes and age effects of normal adults. J Gerontol. 1971;26:90-105.
42. Bowden JL, McNulty PA. Age-related changes in cutaneous sensation in the healthy human hand. Age (Dordr). 2013;35(4):1077-1089.
43. Jacobs R. Physical changes in the aged. In: O’Hara-Devereaux M, Andrus LH, Scott CD, eds. Eldercare. New York, NY: Grune & Statton; 1981.
44. Klein R, Klein BE. The prevalence of age-related eye diseases and visual impairment in aging: current estimates. Invest Ophthalmol Vis Sci. 2013;54(14):5-13.
45. Boyer GG. Vision problems. In: Carnevali P, Patrick B, eds. Nursing Management for the Elderly. Philadelphia, PA: JB Lippincott Co; 1989.
46. Vaden KI, Matthews LJ, Eckert MA, Dubno JR. Longitudinal changes in audiometric phenotypes of age-related hearing loss. J Assoc Res Otolaryngol. 2017;18(2):371-385.
47. Woollacott MH, Shumway-Cook A, Nashner LM. Aging and posture control: changes in sensory organization and muscular coordination. Int J Aging Hum Dev. 1986;23:97-114.
48. Cyran CA, Boegle R, Stephan T, Dieterich M, Glasauer S. Age-related decline in functional connectivity of the vestibular cortical network. Brain Struct Funct. 2016;221(3):1443-1463.
49. Iwanmoto T, Hanyu H, Umahara T. Age-related changes of sensory system. Nihon Rinsho. 2013;71(10):1720-1725.
50. Khan TA, Shragge BW, Crispen JS, et al. Esophageal mobility in the elderly. Am J Dig Dis. 1977;22:1049-1054.
51. Bartol MA, Heitkemper M. Gastrointestinal problems. In: Carnevali P, Patrick B, eds. Nursing Management for the Elderly. Philadelphia, PA: JB Lippincott Co; 1989.
52. Horowitz M, Maddern GT, Chateron BE, et al. Changes in gastric emptying rates with age. Clin Sci (Lond). 1984;67:213-218.
53. Tan JL, Eastment JG, Poudel A, Hubbard RE. Age-related changes in hepatic function: an update on implication for drug therapy. Drugs Aging. 2015;32(12):999-1008.
54. Goldman R. Decline in organ function with age. In: Rossman I, ed. Clinical Geriatrics. 2nd ed. Philadelphia, PA: Lippincott; 1979.
55. Lindeman RD. Is the decline in renal function with normal aging inevitable? Geriatr Nephrol Urol. 1998;8(1):7-9.
56. Mizuno MS, Pompeu E, Castelucci P, Liberti EA. Age-related changes in urinary bladder intramural neurons. Int J Dev Neurosci. 2007;25(3):141-148.
57. Goodman NE, Cobin RH, Ginzburg SB. American Association of Clinical Endocrinologists medical guidelines for clinical practice for the diagnosis and treatment of menopause. Endocr Pract. 2011;17:1-25.
58. Minaker KL. Common clinical sequelae of aging. In: Goldman L, Schafer AI, eds. Goldman’s Cecil Medicine. 24th ed. Philadelphia, PA: Elsevier Saunders; 2011.
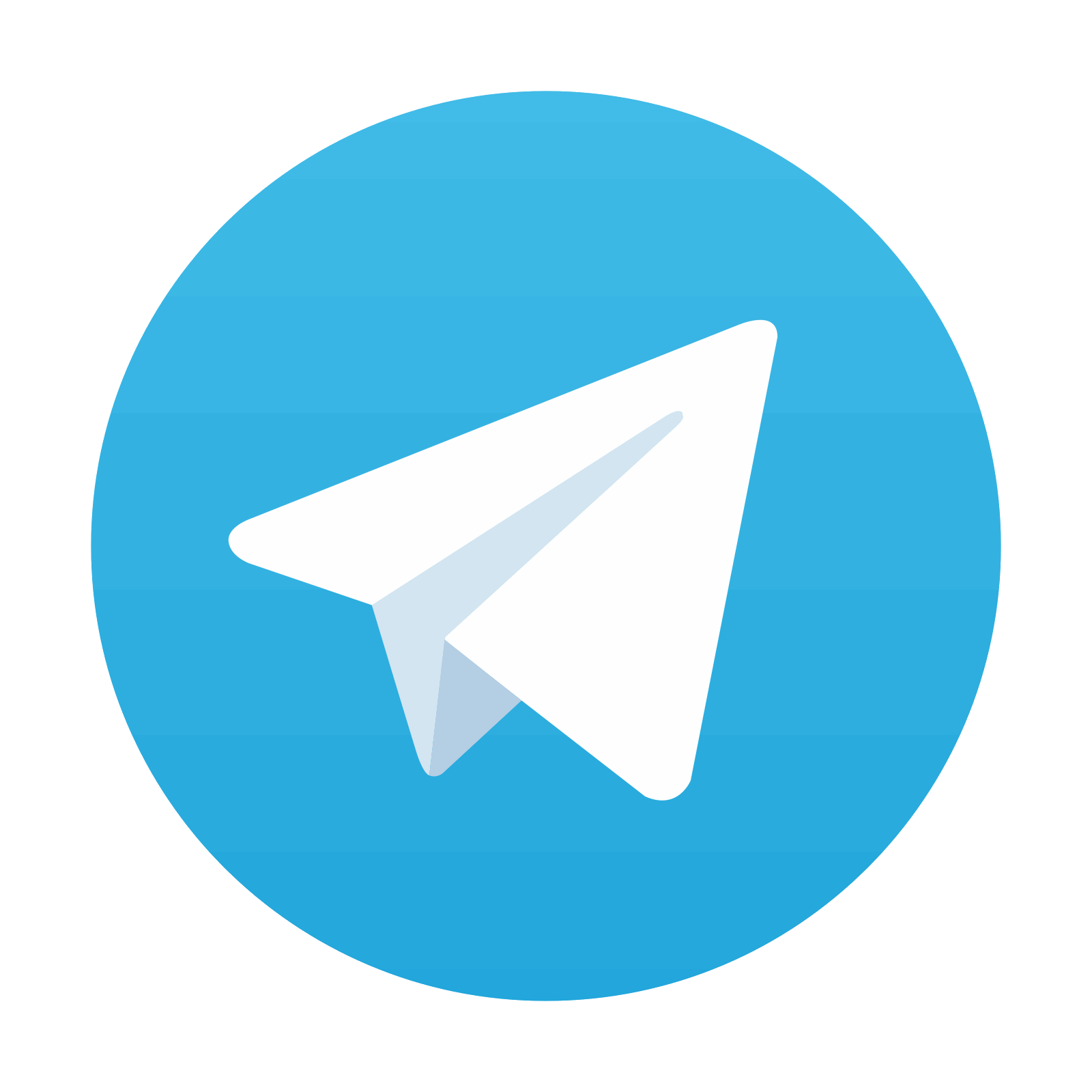
Stay updated, free articles. Join our Telegram channel

Full access? Get Clinical Tree
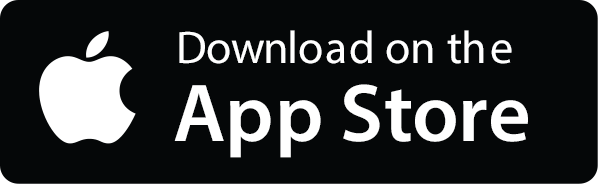
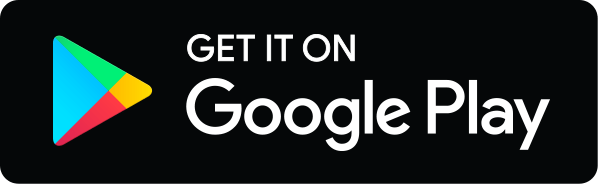