*Normal black and Middle Eastern subjects may have lower counts. In normal pregnancy the upper limits are: total leucocytes 14.5 × 109/L, neutrophils 11 × 109/L.
Figure 8.1 White blood cells (leucocytes): (a) neutrophil (polymorph); (b) eosinophil; (c) basophil; (d) monocyte; (e) lymphocyte.
The function of phagocytes and immunocytes in protecting the body against infection is closely connected with two soluble protein systems of the body: immunoglobulins and complement. These proteins, which may also be involved in blood cell destruction in a number of diseases, are discussed together with the lymphocytes in Chapter 9.
Neutrophil (polymorph)
This cell has a characteristic dense nucleus consisting of between two and five lobes, and a pale cytoplasm with an irregular outline containing many fine pink–blue (azurophilic) or grey–blue granules (Fig. 8.1a). The granules are divided into primary, which appear at the promyelocyte stage, and secondary (specific) which appear at the myelocyte stage and predominate in the mature neutrophil (Fig 8.7). Both types of granule are lysosomal in origin (Fig. 8.7). The lifespan of neutrophils in the blood is only 6–10 hours.
Neutrophil precursors
These do not normally appear in normal peripheral blood but are present in the marrow (Fig. 8.2). The earliest recognizable precursor is the myeloblast, a cell of variable size that has a large nucleus with fine chromatin and usually two to five nucleoli (see Fig. 13.4). The cytoplasm is basophilic and no cytoplasmic granules are present. The normal bone marrow contains up to 5% of myeloblasts. Myeloblasts give rise by cell division to promyelocytes which are slightly larger cells and have developed primary granules in the cytoplasm. These cells then divide and differentiate to myelocytes which have specific or secondary granules. The nuclear chromatin is now more condensed and nucleoli are not visible. Separate myelocytes of the neutrophil, eosinophil and basophil series can be indentified. The myelocytes give rise by cell division and differentiation to metamyelocytes, non-dividing cells, which have an indented or horseshoe-shaped nucleus and a cytoplasm filled with primary and secondary granules. Neutrophil forms between the metamyelocyte and fully mature neutrophil are termed ‘band’, ‘stab’ or ‘juvenile’. These cells may occur in normal peripheral blood. They do not contain the clear, fine filamentous connections between nuclear lobes that is seen in mature neutrophils.
Figure 8.2 The formation of the neutrophil and monocyte phagocytes. Eosinophils and basophils are also formed in the marrow in a process similar to that for neutrophils.
Monocytes
These are usually larger than other peripheral blood leucocytes and possess a large central oval or indented nucleus with clumped chromatin (Fig. 8.1d). The abundant cytoplasm stains blue and contains many fine vacuoles, giving a ground-glass appearance. Cytoplasmic granules are also often present. The monocyte precursors in the marrow (monoblasts and promonocytes) are difficult to distinguish from myeloblasts and monocytes.
Eosinophils
These cells are similar to neutrophils, except that the cytoplasmic granules are coarser and more deeply red staining and there are rarely more than three nuclear lobes (Fig. 8.1b). Eosinophil myelocytes can be recognized but earlier stages are indistinguishable from neutrophil precursors. The blood transit time for eosinophils is longer than for neutrophils. They enter inflammatory exudates and have a special role in allergic responses, defence against parasites and removal of fibrin formed during inflammation.
Basophils
These are only occasionally seen in normal peripheral blood. They have many dark cytoplasmic granules which overlie the nucleus and contain heparin and histamine (Fig. 8.1c). In the tissues they become mast cells. They have immunoglobulin E (IgE) attachment sites and their degranulation is associated with histamine release.
The blood granulocytes and monocytes are formed in the bone marrow from a common precursor cell (see Fig. 1.2). In the granulopoietic series progenitor cells, myeloblasts, promyelocytes and myelocytes form a proliferative or mitotic pool of cells while the metamyelocytes, band and segmented granulocytes make up a post-mitotic maturation compartment (Fig. 8.3). Large numbers of band and segmented neutrophils are held in the marrow as a ‘reserve pool’ or storage compartment. The bone marrow normally contains more myeloid cells than erythroid cells in the ratio of 2: 1 to 12: 1, the largest proportion being neutrophils and metamyelocytes. In the stable or normal state, the bone marrow storage compartment contains 10–15 times the number of granulocytes found in the peripheral blood.
Figure 8.3 Neutrophil kinetics. CSF, colony-stimulating factor; G, granulocyte; IL, interleukin; M, monocyte; SCF, stem cell factor.
Following their release from the bone marrow, granulocytes spend only 6–10 hours in the circulation before moving into the tissues where they perform their phagocytic function. In the bloodstream there are two pools usually of about equal size: the circulating pool (included in the blood count) and the marginating pool (not included in the blood count). They spend on average 4–5 days in the tissues before they are destroyed during defensive action or as the result of senescence.
Control of granulopoiesis: myeloid growth factors
The granulocyte series arises from bone marrow progenitor cells which are increasingly specialized. Many growth factors are involved in this maturation process including interleukin-1 (IL-1), IL-3, IL-5 (for eosinophils), IL-6, IL-11, granulocyte–macrophage colony-stimulating factor (GM-CSF), granulocyte CSF (G-CSF) and monocyte CSF (M-CSF) (see Fig. 1.7). The growth factors stimulate proliferation and differentiation and also affect the function of the mature cells on which they act (e.g. phagocytosis, superoxide generation and cytotoxicity in the case of neutrophils; phagocytosis, cytotoxicity and production of other cytokines by monocytes) (Fig. 1.6).
Increased granulocyte and monocyte production in response to an infection is induced by increased production of growth factors from stromal cells and T lymphocytes, stimulated by endotoxin, IL-1 or tumour necrosis factor (TNF) (Fig. 8.4).
Figure 8.4 Regulation of haemopoiesis; pathways of stimulation of leucopoiesis by endotoxin, for example from infection. It is likely that endothelial and fibroblast cells release basal quantities of granulocyte–macrophage colony-stimulating factor (GM-CSF) and granulocyte colony-stimulating factor (G-CSF) in the normal resting state and that this is enhanced substantially by tumour necrosis factor (TNF) and interleukin-1 (IL-1) from monocytes.
Clinical applications of G-CSF
Clinical administration of G-CSF intravenously or subcutaneously produces a rise in circulating neutrophils. G-CSF is used in clinical practice. Short-acting G-CSF is given daily. A longer actingpegylated (PEG) G-CSF can be given once in 7–14 days. Some of the indications are as follows:
- Post-chemotherapy, radiotherapy or stem cell transplantation In these situations, G-CSF accelerates granulocytic recovery and shortens the period of neutropenia (Fig. 8.5). This may translate into a reduction of length of time in hospital, antibiotic usage and frequency of infection but periods of extreme neutropenia after intensive chemotherapy cannot be prevented.
- Acute myeloid leukaemia As in other haematological malignancies treated with intensive chemotherapy G-CSF has been used to reduce infections, hospital stay and antibiotic usage. There is no evidence that G-CSF injections can precipitate relapse of the disease.
- Myelodysplasia G-CSF has been given alone or in conjunction with erythropoietin in an attempt to improve bone marrow function (without accelerating leukaemic transformation).
- Lymphomas G-CSF is given to reduce infection, delay in giving chemotherapy and hospitalization after chemotherapy. A single injection of pegylated G-CSF immediately after chemotherapy is often used.
- Severe neutropenia Both congenital and acquired neutropenia, including cyclical and drug-induced neutropenia, have been found to respond well to G-CSF.
- Severe infection G-CSF has been used as an adjuvant to antimicrobial therapy.
- Peripheral blood stem cell harvesting G-CSF is used to increase the number of circulating multipotent progenitors, improving the harvest of sufficient peripheral blood stem cells for transplantation.
Figure 8.5 Typical effect of granulocyte colony-stimulating factor (G-CSF) on recovery of neutrophils following autologous bone marrow transplantation.
Monocytes spend only a short time in the marrow and, after circulating for 20–40 hours, leave the blood to enter the tissues where they mature and carry out their principal functions. Their extravascular lifespan after their transformation to macrophages (histiocytes) may be as long as several months or even years. They may assume specific functions in different tissues (e.g. skin, gut, liver) (Fig. 8.6). One particularly important lineage is that of dendritic cells which are involved in antigen presentation to T cells (see Chapter 9). GM-CSF and M-CSF are involved in their production and activation.
Figure 8.6 Reticuloendothelial system: distribution of macrophages. APC, antigen presenting cells.
Disorders of neutrophil and monocyte function
The normal function of neutrophils and monocytes may be divided into three phases.
Chemotaxis (cell mobilization and migration)
The phagocyte is attracted to bacteria or the site of inflammation by chemotactic substances released from damaged tissues or by complement components and also by the interaction of leucocyte adhesion molecules with ligands on the damaged tissues. The leucocyte adhesion molecules also mediate recruitment, migration and interaction with other immune cells. They are also variously expressed on endothelial cells and platelets (see Chapter 1).
Phagocytosis
The foreign material (e.g. bacteria, fungi) or dead or damaged cells of the host are phagocytosed (Fig. 8.7). Recognition of a foreign particle is aided by opsonization with immunoglobulin or complement because both neutrophils and monocytes have Fc and C3b receptors (see Chapter 9). Opsonization of normal body cells (e.g. red cells or platelets) also makes them liable to destruction by macrophages of the reticuloendothelial system, as in autoimmune haemolysis, idiopathic (autoimmune) thrombocytopenic purpura or many of the drug-induced cytopenias.
Figure 8.7 Phagocytosis and bacterial destruction. On entering the neutrophil, the bacterium is surrounded by an invaginated surface membrane and fuses with a primary lysosome to form a phagosome. Enzymes from the lysosome attack the bacterium. Secondary granules also fuse with the phagosomes, and new enzymes from these granules including lactoferrin attack the organism. Various types of activated oxygen, generated by glucose metabolism, also help to kill bacteria. Undigested residual bacterial products are excreted by exocytosis.
Macrophages have a central role in antigen presentation: processing and presenting foreign antigens on human leucocyte antigen (HLA) molecules to the immune system. They also secrete a large number of growth factors and chemokines that regulate inflammation and immune responses.
Chemokines are chemotactic cytokines of which there are two main classes: CXC (α) chemokines, small (8–10 000 MW) pro-inflammatory cytokines which mainly act on neutrophils, and CC (β) chemokines such as macrophage inflammatory protein-1α (MIP-1α) and RANTES, which act on monocytes, basophils, eosinophils and natural killer (NK) cells. Chemokines may be produced constitutively and control lymphocyte traffic under physiological conditions; inflammatory chemokines are induced or up-regulated by inflammatory stimuli. They bind to and activate cells via chemokine receptors and play an important part in recruiting appropriate cells to the sites of inflammation.
Killing and digestion
This occurs by oxygen-dependent and oxygen-independent pathways. In the oxygen-dependent reactions, superoxide (O2−), hydrogen peroxide (H2O2) and other activated oxygen (O2) species, are generated from O2 and reduced nicotinamide adenine dinucleotide phosphate (NADPH). In neutrophils, H2O2 reacts with myeloperoxidase and intracellular halide to kill bacteria; activated oxygen may also be involved. The non-oxidative microbicidal mechanisms involve microbicidal proteins. These may act alone (e.g. cathepsin G) or in conjunction with H2O2 (e.g. lysozyme, elastase). They may also act with a fall in pH within phagocytic vacuoles into which lysosomal enzymes are released. An additional iron binding protein, lactoferrin, is present in neutrophil granules and is bacteriostatic by depriving bacteria of iron and generating free radicals (Fig. 8.7). Finally, nitric oxide (NO) generated through NO synthase from L-arginine is another mechanism by which phagocytes kill microbes.
Defects of phagocytic cell function
Chemotaxis
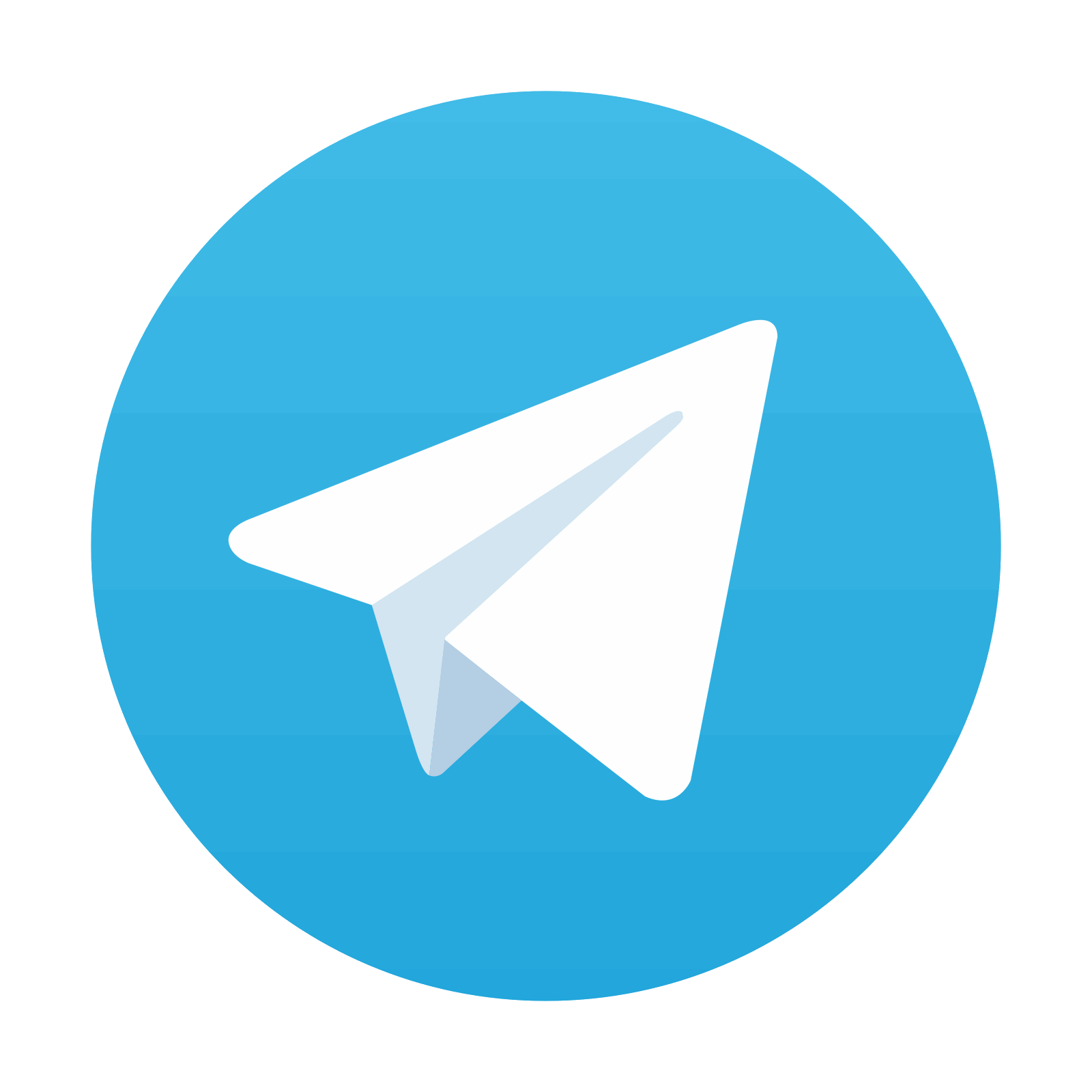
Stay updated, free articles. Join our Telegram channel

Full access? Get Clinical Tree
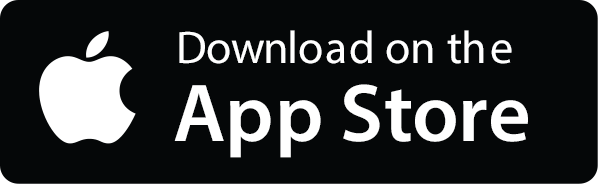
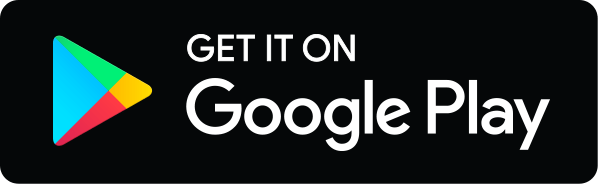