β Agonists
Jacqueline A. Pongracic
Since bronchoconstriction has long been regarded to be a hallmark of asthma, bronchodilation has become an important component of asthma therapy. Among the various agents available for this purpose, β-adrenergic agonists have played a prominent role. Short-acting β agonists are the mainstay of rescue therapy. The more recent availability of long-acting preparations has changed the way β agonists may be used.
Historical Perspectives
Sympathomimetic agents have been used for asthma for thousands of years. Ephedrine, which is found in Ma huang (Ephedra sinica), has been used by the Chinese since 3000 BC. Because its therapeutic benefits were noted to wane over time, while adverse reactions such as central nervous system stimulation increased, new and improved sympathomimetic agents were highly desired. Subcutaneous injections of adrenaline were used in the early 1900s but were also associated with unacceptable side effects. It was only 60 years ago that the first β-adrenergic agonist, isoproterenol, appeared on the scene (1). As a potent, nonselective β agonist, isoproterenol was associated with many side effects but fewer than occurred with adrenaline. These toxicity issues and the identification of α and β adrenoreceptors led to the development of the β 2-selective agonist, albuterol, in the 1960s. Since then, a variety of other β 2-selective agonists have been developed as well. Pirbuterol, terbutaline, and fenoterol are short, rapidly acting β agonists (SABA). Fenoterol is potent, but less β 2 selective than the others, and it is not available in the United States. In response to continued concerns about side effects, further examination and refinements in these molecules have led to the production of an enantiomeric form of albuterol, called levalbuterol. Long-acting β agonists (LABA) have also become available. Salmeterol and formoterol represent this newer class of β-adrenergic agonists.
Mechanism of Action and Pharmacology
β-Adrenergic agonists exert their effects through interactions with membrane-bound receptors. Three types of β-adrenergic receptors have been characterized: β 1, β 2, and β 3. The β 1 receptors predominate in the heart, whereas β 3 receptors are found in adipose tissue. The β 2 receptors are ubiquitous; in the lung, residing in smooth muscle, submucosal glands, epithelium, and alveoli as well as in smooth muscle and endothelium of the pulmonary arterial system. Radioligand binding studies and computed tomographic imaging have shown that these receptors are present in greater concentrations in the central lung and alveoli. There are β 2 receptors also found on a variety of inflammatory cells commonly associated with asthma, including mast cells, macrophages, neutrophils, eosinophils, and lymphocytes. β2 adrenoceptors are present in very high concentrations in airway smooth muscle, and less so in epithelial cells, endothelial cells, type II cells, and mast cells.
The β2-adrenergic receptor, illustrated in Figure 34.1, is a member of a superfamily of 7-transmembrane G-protein–coupled receptors encoded by a gene on chromosome 5 (2). Until recently, a lock-and-key mechanism by which β agonists engaged the receptor was hypothesized. However, it appears that β2-adrenergic receptors vacillate between inactive and active states (3) and β agonists may shift the equilibrium to favor the activated state. An agonist drug, such as albuterol binds to the extracellular domain of the receptor and induces a conformational change so that the intracellular regions of the receptor may bind to a G protein. As a result, adenylyl cyclase is activated and causes an increase in cyclic adenosine monophosphate (cAMP). cAMP acts as a second messenger by activating protein kinase A, which causes phosphorylation with resultant relaxation of airway smooth muscle. cAMP also augments intracellular calcium ion stores leading to relaxation of airway smooth muscle.
![]() Figure 34.1 The structure of the human β2-adrenergic receptor. Regions involved in G protein coupling are bolded. Sites involved in β2-agonist binding are marked as X. |
Review of the development of β adrenergic agents highlights the functional differences among these medications. The early β agonists were initially modeled after adrenaline and noradrenaline. Structural modifications of these catecholamines were noted to impart functional changes in these compounds. For example, substitutions in the hydroxyl groups on the benzene ring reduce inactivation by the gastrointestinal enzyme catechol O-methyltransferase, as is the case for albuterol, terbutaline, metaproterenol, and fenoterol. These specific alterations increase duration of action and allow for oral administration. Modifications of the side-chain increase selectivity for the β 2 receptor, reduce inactivation by monoamine oxidase, and extend duration of action, as is seen for albuterol, terbutaline, pirbuterol, and procaterol. Salmeterol and formoterol have much larger lipophilic side chains that account for their long-lasting β 2-selective effects. Despite their structural and functional similarities, salmeterol and formoterol have different mechanisms of action at the cellular level (4). Salmeterol, which is highly lipophilic, is rapidly taken up into the cell after which it gradually diffuses out to interact with the receptor. Its side chain engages with an exocite of the receptor acting as an anchor to prevent dissociation of the agonist from the receptor while the rest of the molecule engages and disengages the active site of the receptor like a hinge (2).
The response to agonists also varies by polymorphisms of the β2-adrenoceptor. There are two genes for the receptor so that individuals may be homozygous or heterozygous for any given polymorphism. Studies have found nine distinct polymorphisms characterized by a single base alteration. Some, but not all, studies have shown that mutations at codons 16 and 27 are associated with altered bronchodilator responses (5,6) and pulmonary function (7,8).
Short-Acting β Agonists
SABAs induce relaxation of airway smooth muscle quickly. For example, albuterol produces bronchodilation within 5 minutes of inhalation. Its pharmacologic effects peak after 60 to 90 minutes and last 4 to 6 hours. Because β 2 receptors are also found on a variety of inflammatory cells, investigators have postulated that β 2 agonists may also possess anti-inflammatory effects. Albuterol inhibits histamine release from activated mast cells in vitro (9). Inhibitory effects have also been demonstrated on eosinophils (10–13), lymphocytes (14–16), and neutrophils (17,18). In vivo studies of albuterol have failed to uphold an anti-inflammatory effect and, in fact, show a potentiated late-phase response, elevation in sputum eosinophils, and increased number of activated eosinophils in bronchial biopsy specimens (19,20).
Until recently, all SABAs in clinical use have been racemic mixtures of two mirror-image stereoisomers, called R and S, in equal parts. (R)-isomers induce bronchodilator responses, whereas (S)-isomers do not. Studies performed in humans have demonstrated that regular use of racemic albuterol is associated with increases in airway responsiveness to allergen (19,21). In vitro, (R)-albuterol induces bronchodilation in isolated human trachea (22), whereas (S)-albuterol augments contractile responses to histamine and leukotriene C4 in bronchial tissue (23). In isolated
smooth muscle cells, (S)-albuterol increases calcium influx (24,25). (S)-albuterol has much less affinity for β 2 receptors than does (R)-albuterol (26). (S)-albuterol appears to have proinflammatory effects as well, with evidence of eosinophil activation demonstrated through elevations in superoxide and eosinophil peroxidase (27,28).
smooth muscle cells, (S)-albuterol increases calcium influx (24,25). (S)-albuterol has much less affinity for β 2 receptors than does (R)-albuterol (26). (S)-albuterol appears to have proinflammatory effects as well, with evidence of eosinophil activation demonstrated through elevations in superoxide and eosinophil peroxidase (27,28).
In vivo, differences between (R)-albuterol and (S)-albuterol are also evident. (S)-albuterol is metabolized 10 times more slowly than (R)-albuterol (29–31) and is detectable in the blood stream for up to 24 hours after administration of racemic albuterol (30). Formulations of (R)-albuterol, called levalbuterol, are available for nebulized and metered-dose inhaler (MDI) administration. The safety and efficacy of levalbuterol in adults and children has been well documented. A multicenter randomized study in 362 teenagers and adults with moderate-to-severe asthma reported that 0.63 mg of levalbuterol was as effective as 2.5 mg of racemic albuterol over 4 weeks of administration (32). Because of the flat dose–response curve, this study failed to show a significant difference with regard to efficacy between levalbuterol and racemic albuterol. Similarly, there was no difference in dose-dependent side effects between levalbuterol and racemic albuterol. In a smaller study of levalbuterol and racemic albuterol in children, lower doses of levalbuterol were as effective as 2.5 mg of racemic albuterol, and all treatments were equally well tolerated in terms of side effects (33). Other studies have addressed whether levalbuterol has a bronchoprotective effect. Using methacholine challenge, a small randomized, double-blind, placebo-controlled study showed a small, sustained bronchoprotective effect for levalbuterol as compared with (S)-albuterol and racemic albuterol (34). A small increase in airway hyperresponsiveness was seen for (S)-albuterol. Other investigations have not confirmed this finding (35,36), yet regular treatment with (R)-albuterol and racemic albuterol results in partial loss of bronchoprotection after methacholine challenge (36).
Long-Acting β Agonists
LABAs provide bronchodilation for 12 hours, much longer than that seen with SABAs. Interestingly, salmeterol and formoterol differ in time to onset of action. Salmeterol effects are seen in 10 to 20 minutes, whereas formoterol actions begin in as little as 1 to 3 minutes. In addition to their bronchodilatory properties, LABA have bronchoprotective effects. This has been shown for bronchoprovocation with methacholine (37–39), histamine (40), exercise (41), hyperventilation (42,43), sulfur dioxide (44), and distilled water (45).
Salmeterol and formoterol also inhibit allergen-induced early- and late-phase airway responses and accompanying bronchial hyperresponsiveness (46–48). This has led to speculation about potential anti-inflammatory effects by LABA. In vitro studies support these effects. Salmeterol inhibits antigen-induced mediator release from human lung mast cell preparations (49) and thromboxane B2 synthesis from human alveolar macrophages (50). Salmeterol and formoterol also inhibit neutrophil leukotriene B4 production (51). Several in vivo studies of salmeterol in humans have documented no anti-inflammatory effect as measured by bronchoalveolar lavage (52,53), bronchial biopsy (54), sputum or circulating eosinophils or eosinophilic cationic protein (ECP) (55–57), or urinary leukotriene E4 excretion (58), despite improvements in peak expiratory flow rates and decreased need for rescue therapy. Other studies have documented reductions in sputum eosinophils (59), ECP in bronchoalveolar lavage (60), serum ECP (61), and airway mucosal mast cells and eosinophils (62). Most evidence suggests that despite their anti-inflammatory effects in vitro, LABA do not exhibit significant anti-inflammatory effects in vivo. When used in combination with inhaled corticosteroids, LABAs do not appear to offer additional anti-inflammatory effects in most studies (63–65). It is important to note that these agents do not appear to enhance airway inflammation.
Clinical Use of β Agonists in Asthma
Current national guidelines promote the regular daily use of anti-inflammatory, or “controller,” agents for persistent asthma (66). Despite the use of controller therapy, some individuals may develop breakthrough symptoms or acute exacerbations of their disease. SABAs are recommended for the relief of acute asthma symptoms and also for prevention of exercise-induced bronchospasm. SABAs are preferred over other bronchodilators, such as methylxanthines and anticholinergic agents, because SABAs exhibit faster onset of action without significant adverse effects when used appropriately. These guidelines also suggest that the frequency with which SABAs are needed for symptom relief serves as a useful marker of asthma control and of the need for adjusting anti-inflammatory therapy. In fact, SABA prescription refills have been shown to be a good marker for asthma morbidity, with refills typically occurring on or the day after asthma-related emergency department visits, and hospitalizations (67).
SABA may also be used to confirm the diagnosis of asthma by establishing whether reversible bronchospasm exists (66).
SABA are also effective for the prevention of symptoms, such as exercise-induced bronchospasm, when used 5 to 15 minutes before exercise (68,69). Given their short duration of action, SABA are not well suited for the prevention of nocturnal symptoms.
Similar to other SABAs, levalbuterol should be used for rescue and prevention of exercise-induced bronchoconstriction but not for maintenance therapy. Randomized controlled trials of levalbuterol versus albuterol conducted in the emergency department have shown conflicting results for superiority of levalbuterol on hospitalization rates (70), time to discharge (71), and clinical improvement (72
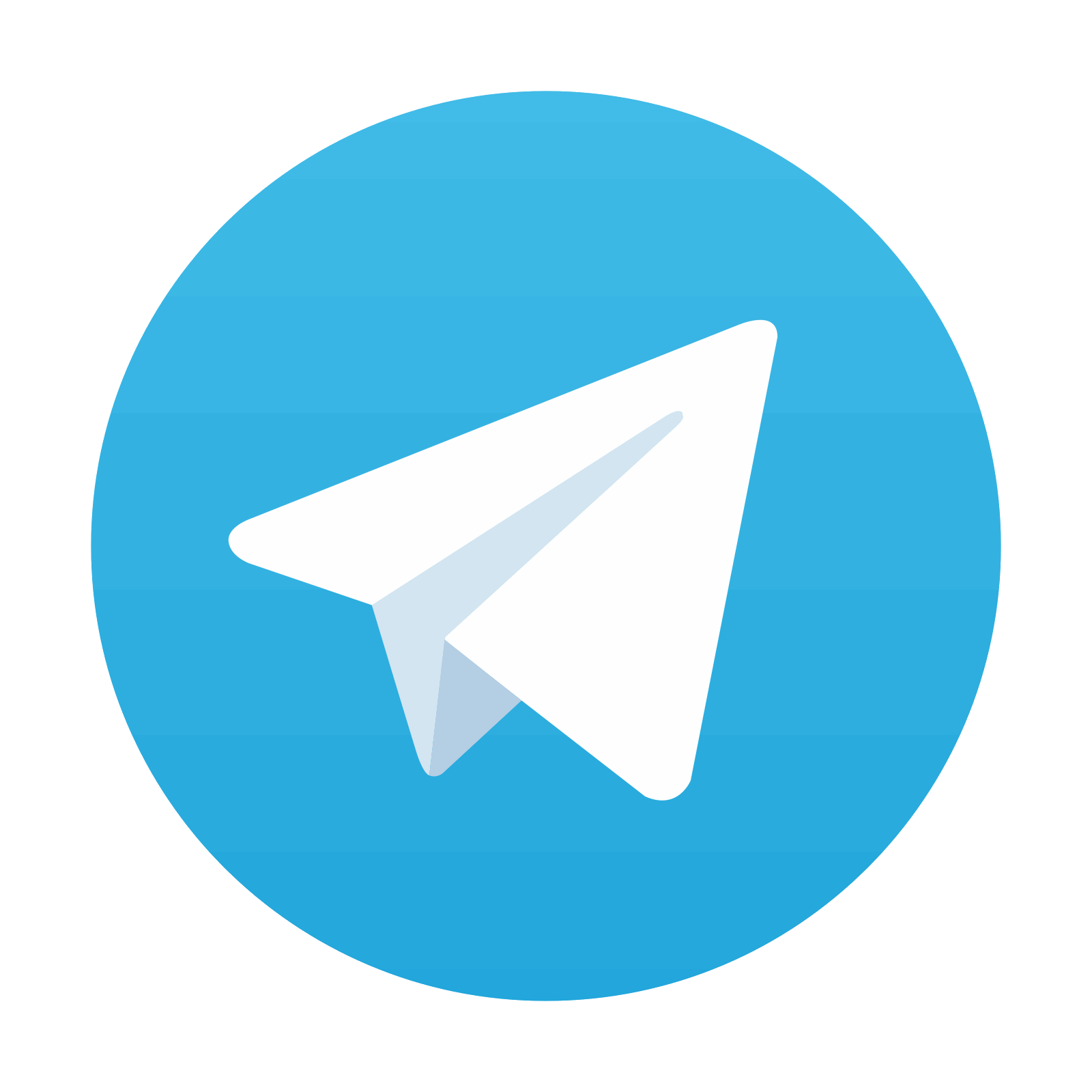
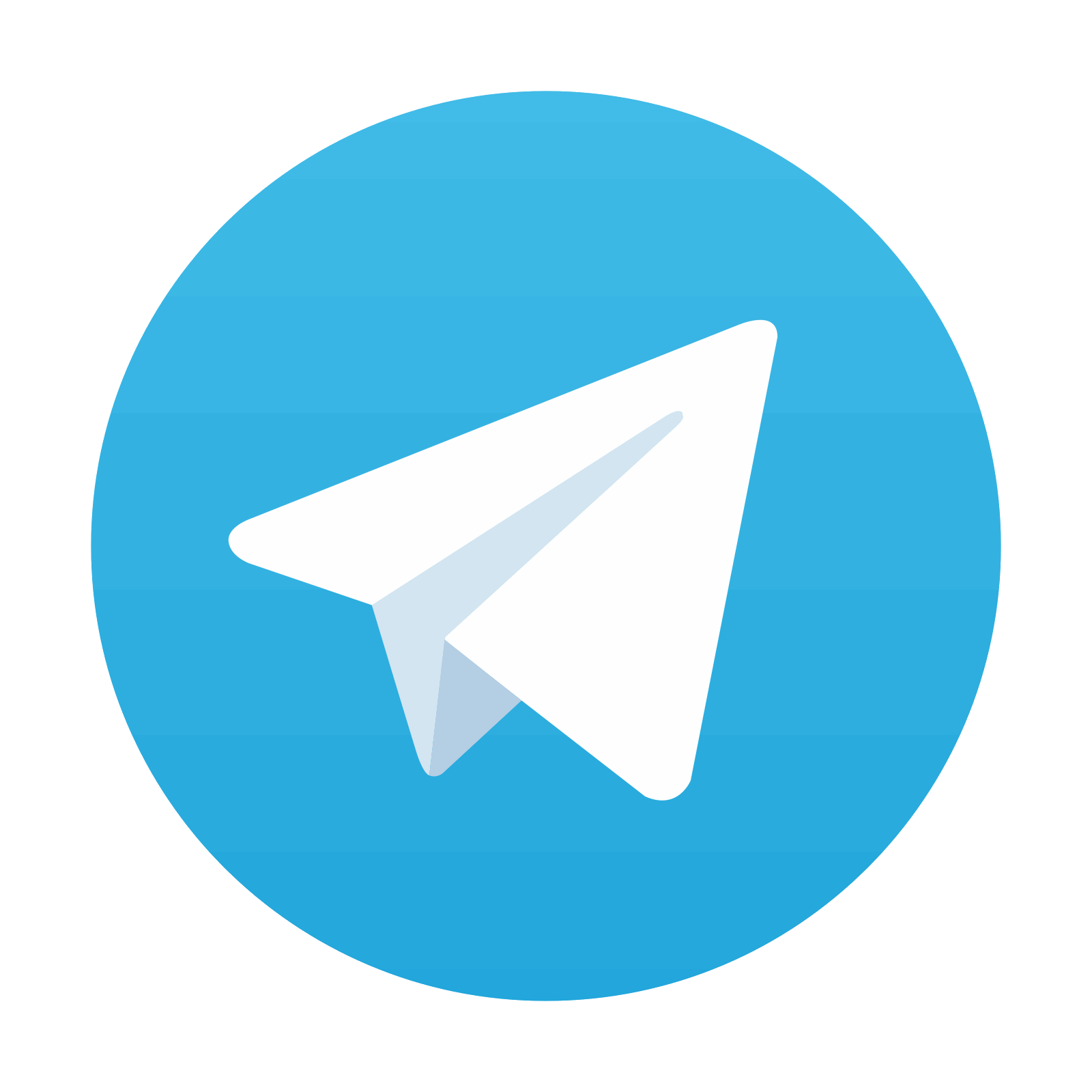
Stay updated, free articles. Join our Telegram channel

Full access? Get Clinical Tree
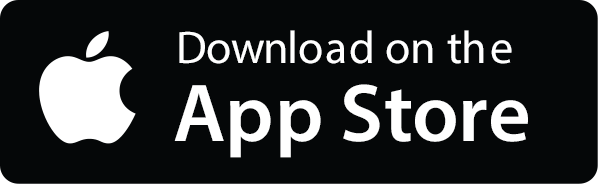
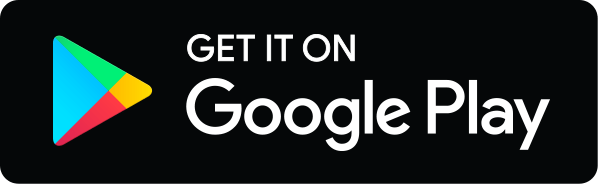