(1)
Mayo Clinic, Jacksonville, Florida, USA
7.1 Introduction
The vitreous humor is the most abundant ocular tissue, comprising the majority (4 ml) of the eye’s volume. Often referred to as the “vitreous gel,” a reference to both its consistency and biomechanical composition, the vitreous extends from the posterior lens capsule back to the retina’s internal limiting membrane (ILM). For centuries, ophthalmologists believed that the vitreous could not be safely manipulated, altered, or removed without precipitating catastrophic consequences such as retinal tears, retinal detachments, suprachoroidal hemorrhages, or endophthalmitis. In retrospect, it has become obvious that physicians lacked a sufficient understanding of vitreous anatomy and physiology and did not possess the proper technology with which to safely and effectively handle the vitreous [60].
The gel-like consistency of the vitreous impedes the diffusion of substances such as vascular endothelial growth factor out of the retina and the movement of oxygen from anterior structures into the retina. The high viscosity of the vitreous (up to six times that of water) slows the resorption of hemorrhage that may result from insufficiently treated proliferative diabetic retinopathy (PDR), and it impedes the elimination of intravitreally administered drugs, thereby improving the effectiveness and duration of pharmacologic therapy. Trial designers recognize the importance of an intact vitreous since they routinely exclude eyes that have already undergone vitrectomy from participating in intravitreal drug trials.
An intact vitreous provides a scaffold for fibrovascular growth in various proliferative retinopathies. Vitreomacular traction (VMT) due to partial vitreous contraction and collapse without complete separation of the posterior hyaloid from the ILM upregulates VEGF production, promotes the formation of macular edema, and limits the efficacy of intravitreal pharmacotherapy.
In eyes with diabetic retinopathy (DR), the vitreous is viewed as both an enemy that contributes to the disease and as an ally that potentiates treatment by providing a reservoir for drug placement. Successfully changing the vitreous by inducing a vitreous detachment or removing most of it has become an important therapeutic approach for many retinal and vitreoretinal interface diseases. Pars plana vitrectomy with the removal of VMT has been used for two decades to treat diabetic macular edema (DME) (Chap. 3).
This chapter will discuss pharmacologic approaches to eliminate the adverse effects of VMT on the formation of diabetic retinopathy.
7.2 Vitreous Anatomy
The vitreous comprises about 80% of the ocular volume [82], and its transparent matrix is composed primarily (98–99%) of water [6]. The gel structure is maintained by a complex branching network of collagen fibrils held apart by hyaluronic acid and other macromolecules. Type II collagen is the most common vitreous protein with highest concentrations found at the vitreous base and in the posterior vitreous cortex [41]. The collagen fiber network gives the gel strength and stability, which enables it to absorb shock from blunt trauma and resume its previous shape after being subjected to distorting forces.
Hyaluronic acid, the most common glycosaminoglycan within the vitreous, stabilizes the collagen network [7, 78]. The hyaluronic acid-collagen matrix correctly spaces fibrils to optimize transparency and decrease light scatter, and endows the vitreous with viscoelastic properties [7, 8, 13]. The vitreous is nearly acellular except for a small number of hyalocytes, phagocytic cells found mostly at the vitreous base and posterior pole [4, 8, 78, 94]. Hyalocytes also synthesize collagen fibrils and hyaluronic acid.
The interface between the vitreous and adjacent structures is composed of vitreous cortical fibrils and the basal laminae of the other tissue [30]. Changes at the vitreous base usually result in retinal tears and detachments, but the interface between the posterior hyaloid and macula is more important in patients with DR. The ILM of the retina consists of the footplates or basal laminae of the Müller cells [79] together with types I and IV collagen, proteoglycans, fibronectin, and laminin [36, 47, 52, 53]. It is not clear which of these molecules must be dissolved to facilitate a posterior vitreous detachment, and even “linker molecules” like lectins, integrins, and chondroitin sulfate may ultimately be responsible for the attachment of the vitreous cortex to the ILM. The ILM varies in thickness from 50 μm at the vitreous base to 300 μm at the equator to 1890 μm over the posterior pole before it thins to only 10–20 μm at the fovea [25, 30, 37]. The firmest vitreous adhesions occur where the ILM is thinnest – at the vitreous base and the fovea.
7.3 Posterior Vitreous Detachment
The vitreous both liquefies and aggregates as it ages. Vitreous liquefaction, or syneresis, begins at the age of 4 years, whereas aggregation of fibrils, or synchysis, begins in midlife and continues into old age (Fig. 7.1) [48, 49]. Changes in hyaluronic acid and its interaction with the collagen fibrils create areas of liquefaction that alternate with areas of increased collagen fiber concentration within the residual gel [45, 59]. Liquefaction in the central vitreous overlying the macula enables incident light to produce free radicals that weaken the residual collagen and decrease the concentrations of glycosaminoglycans and chondroitin sulfate [51]. This promotes posterior hyaloid separation from the ILM (posterior vitreous detachment).
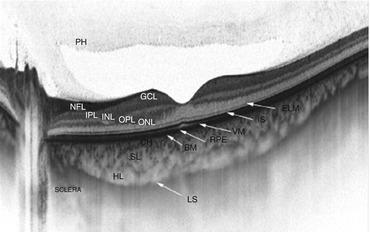
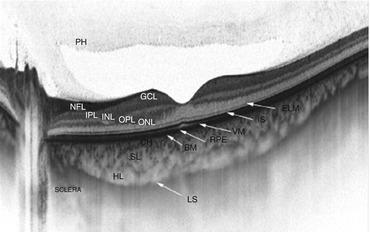
Fig. 7.1
This swept-source optical coherence tomography image shows excellent detail in the vitreous, retina, and choroid. The gel-like consistency of the vitreous appears hyperreflective (top of image). A large preretinal bursa (posterior to the “PH”) due to liquefaction of the vitreous is present over the macula. PH posterior hyaloid, NFL nerve fiber layer, GCL ganglion cell layer, IPL inner plexiform layer, INL inner nuclear layer, OPL outer plexiform layer, ONL outer nuclear layer, ELM external limiting membrane, IS inner segment/outer segment line, VM cone outer segments, RPE retinal pigment epithelium, CH choriocapillaris, SL Sattler’s layer, HL Haller’s layer, LS junction between choroid and sclera
Most posterior vitreous detachments (PVDs) result from a combination of vitreous synchysis and weakening of the adhesions between the ILM and posterior hyaloid. Liquefied premacular vitreous slowly migrates into the potential space between the posterior hyaloid and ILM, and since the adhesions have been weakened, the two surfaces slowly begin to separate, beginning in the peripheral macula (Fig. 7.2). Saccadic eye movements and continued loss of adherent molecules cause further cleavage with progressive enlargement of the preretinal space that marches toward the fovea. A final collapse of the vitreous gel with complete separation of the posterior hyaloid from the fovea and optic disc characterizes a PVD [22, 58, 77].
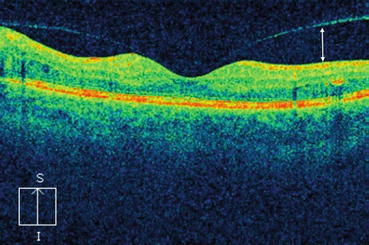
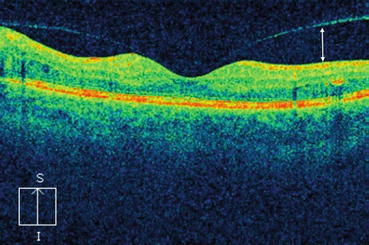
Fig. 7.2
This optical coherence tomography scan shows the age-related separation between the posterior hyaloid and the internal limiting membrane (white arrow). This begins in the peripheral macula and moves toward the fovea before the posterior hyaloid completely detaches from the macula
7.4 Anomalous Posterior Vitreous Detachment
Incomplete separation of the posterior hyaloid because of residual vitreomacular adhesion constitutes an anomalous PVD [84]. If the residual vitreous adhesion causes distortion of the macula by elevating the ILM, this is termed vitreomacular traction. Excessive gel liquefaction is seen in many systemic conditions including diabetes [80] and together with strong vitreomacular adhesion may cause symptomatic vitreomacular traction or even macular holes. In patients with diabetic retinopathy, this can cause diabetic macular edema (DME).
Diagnosing an anomalous vitreous detachment can sometimes be done with slit-lamp biomicroscopy and a magnifying fundus lens (Fig. 7.3), but spectral domain optical coherence tomography (SD-OCT) is an easier and more accurate method of visualizing the partially detached posterior hyaloid [31, 54, 75, 97]. Correctly differentiating between a complete PVD and a completely attached posterior hyaloid can be difficult with OCT, and ultrasound is a more accurate tool to discriminate between these two conditions.
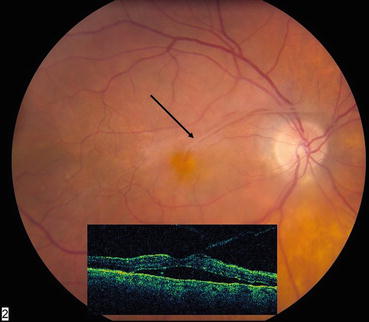
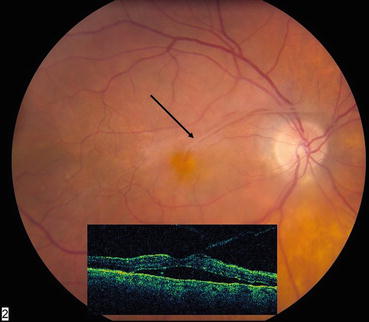
Fig. 7.3
In the color photograph, the taut, opaque posterior hyaloid can be seen just above the fovea (arrow). The optical coherence tomography scan (insert) shows the anomalous posterior vitreous detachment and the resultant traction macular detachment
7.5 Association Between Posterior Hyaloid and Diabetic Retinopathy
Several studies have investigated the association between integrity of the vitreous and the development and progression of DR. Retinal vessels are normally excluded from the vitreous because development of neovascularization on the retinal surface – proliferative diabetic retinopathy (PVR) – requires a scaffold of collagenous material [21]. Elevated glucose levels in the vitreous induce the formation of advanced glycation end products [85] and promote cross-linking of collagen and the development of excess vitreous liquefaction, without promoting dehiscence of the posterior cortical vitreous. Intravitreal proteins that normally inhibit the development of neovascularization may lose this ability because they undergo nonenzymatic glycation [38, 81]. In eyes with DR, growth factors that are synthesized in the retina diffuse into the vitreous and encourage the development of neovascularization [33, 34, 61]. Oxygen transport from the ciliary body to the inner retina may be impeded, which worsens retinal ischemia and further increases the synthesis of growth factors.
Diabetes-mediated breakdown of the blood-retinal barrier leads to an extracellular accumulation of serum proteins, which increases the concentrations of fibronectin and other chemokines at the vitreoretinal surface by tenfold [53]. These molecules stimulate the migration and adhesion of proliferating vascular endothelial cells [9] and fibroblasts to the posterior hyaloid. Contraction of these cells, in the presence of an anomalous PVD, exerts tangential traction on the retina, further upregulates VEGF, and exacerbates DME [70]. Traction also decreases interstitial pressure, which, according to Starling’s Law, promotes passage of fluid out of the vascular lumens [15].
A complete PVD should provide some protection against the development of neovascularization [65, 66], but since islands of residual cortical vitreous remain attached to the ILM, such protection is generally incomplete. Five studies that included over 2000 eyes have looked at the association of PDR and BDR development according to the status of the posterior hyaloid [2, 46, 69, 90, 91]. A pooled analysis of these data found that eyes with complete PVDs had a significantly lower prevalence of PDR (OR 0.1, 95% CI, 0.05–0.18), and the development of a PVD is frequently followed by resolution of DME [42, 100]. Two of the studies found that eyes with partial PVDs had six times the progression rate as those without a PVD and 15 times the progression rate as those with complete PVDs [2, 46, 69, 90, 91].
Removal of the posterior hyaloid may help resolve DME by relieving traction and releasing trapped preretinal growth factors. A liquefied vitreous may accelerate the removal of vascular endothelial growth factor from the inner retina. When surgeons propose treatments for DR that involve the induction of a PVD, they need to remember that a partial PVD may actually worsen the retinopathy.
7.6 Treatment of Vitreomacular Traction
Spectral domain OCT imaging has demonstrated that vitreomacular adhesion (VMA) occurs in the majority of patients over the age of 50 years. Vitreomacular adhesion that distorts the inner retinal contour is termed vitreomacular traction (Fig. 7.4). Macular changes may be limited to loss of the foveal depression, to macular splitting or schisis, or to traction foveal detachment. Patients usually experience decreased central visual function though some may be asymptomatic. The natural history of this process is highly variable, as some eyes achieve complete vitreoretinal separation, others progress to partial or full-thickness macular holes, and a third group remains stable for years.
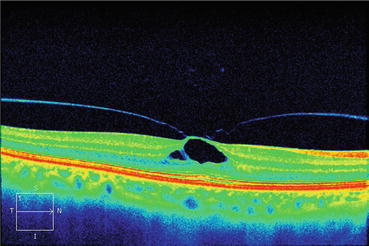
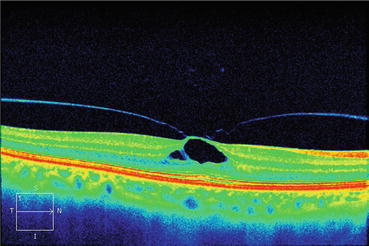
Fig. 7.4
This optical coherence tomography scan shows that the partially detached posterior hyaloid is exerting traction on the inner limiting membrane. The traction causes splitting of the inner retina
Creating a complete PVD to relieve an anomalous partial vitreous detachment has been a topic of considerable interest to retinal surgeons over the last 5 years. Surgical intervention for eyes with symptomatic VMT can be done in two ways. Pars plana vitrectomy removes the core vitreous, after which the posterior hyaloid is detached with aspiration. Despite what appears to be complete removal of the posterior cortical vitreous, islands of cortex frequently remain attached to the ILM. These may sequester growth factors and promote fibrovascular growth. Many surgeons perform a dye-assisted peeling of the ILM to remove all vitreous fragments and minimize the chance of postoperative angiogenesis or traction.
Vitrectomy with ILM removal effectively and predictably removes traction but not without associated risks. Many phakic patients develop cataracts within 2 years that require removal. The risks of retinal tears, retinal detachments, and endophthalmitis are relatively low but still of concern. If the adhesive forces between the posterior cortical vitreous and the ILM are sufficiently strong, vitrectomy can convert VME into a full-thickness macular hole.
Pharmacologic vitreolysis involves the use of drugs that liquefy the vitreous (synchysis) and weaken the adhesion between the posterior hyaloid and the ILM [83]. Intravitreal hyaluronidase was used as early as 1946 [72] and collagenase was used in 1973 [67]. Chondroitinase, dispase, plasmin, and tissue plasminogen activator (tPA) have all been tested in animals and used sporadically in humans [67, 71, 93, 99]. Results with intravitreal hyaluronidase and dispase were disappointing because of insufficient vitreoretinal separation or partial digestion of the inner retina [32, 44, 50, 55, 56, 68, 93]. Only the fibrinolytic enzymes plasmin and tissue plasminogen activator (tPA) showed some success in PVD induction when injected into human eyes [3, 12, 17, 57, 63, 95]. Successful induction of PVDs has been achieved after intravitreal injections of ocriplasmin (Jetrea®, Thrombogenics, Leuven, Belgium) into eyes with symptomatic vitreomacular traction (Fig. 7.5) or stage 2 macular holes (Fig. 7.6). Eyes that responded best to ocriplasmin had the following characteristics: age >65 years of age, vitreomacular adhesion <1500 μm, phakic lens status. and the absence of epiretinal membrane. Macular holes of <400 μm diameter with persistent vitreomacular adhesion also responded well to ocriplasmin.
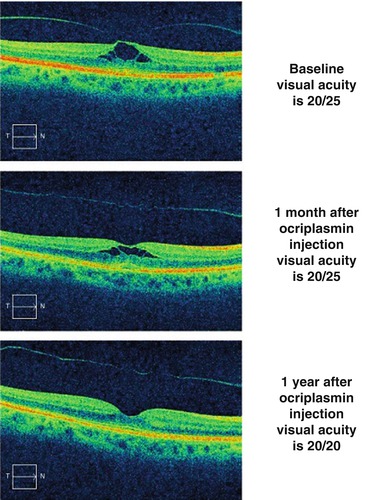
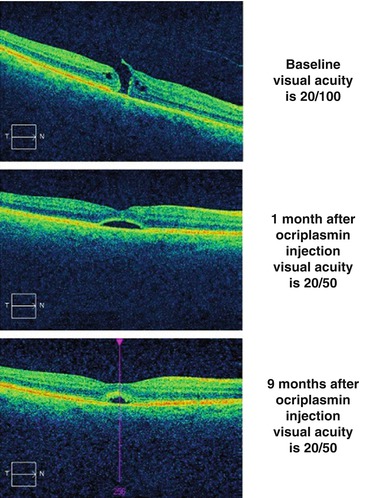
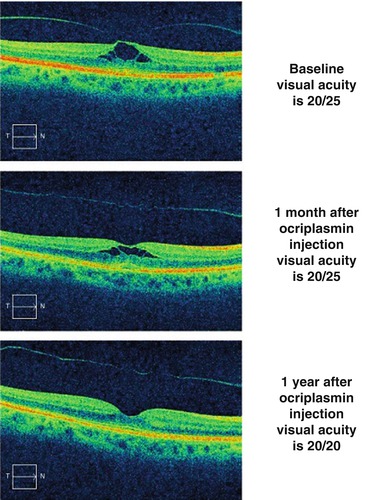
Fig. 7.5
The top optical coherence tomography scan shows vitreomacular traction in a symptomatic patient with visual acuity of 20/25. One month after the intravitreal injection of ocriplasmin (middle scan), the contour of the internal limiting membrane has inverted, and the splitting of the inner retina has diminished. One year after the ocriplasmin injection (bottom scan), the macula appears normal and the posterior hyaloid has completely separated
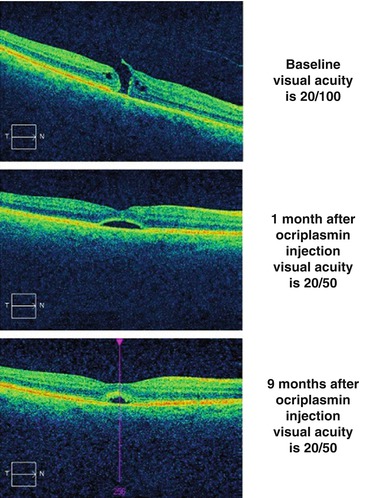
Fig. 7.6
The top scan shows a full-thickness macular hole with persistent traction on an inner retinal flap. One month after the intravitreal injection of ocriplasmin (middle scan), the hole has closed, but the photoreceptor layer is still separated from the retinal pigment epithelium (RPE). Nine months after the intravitreal injection of ocriplasmin (bottom scan), the photoreceptors have moved closer to the RPE
Many surgeons believe that pharmacologic vitreolysis produces a more complete and less traumatic PVD than does surgery [3]. Vitreolysis trials with ocriplasmin and other pharmacologic agents in patients with DME are underway. Table 7.1 lists the drugs that have been used to induce posterior vitreous detachments in animals and humans.
Table 7.1
The drugs that have been investigated for the induction of posterior vitreous detachment (PVD)
Drugs used to induce posterior vitreous detachments | ||
---|---|---|
Drug | Characteristics | Clinical data |
Streptokinase | • Binds to and activates plasminogen • Used to dissolve clots causing myocardial infarctions and pulmonary emboli | • Intravitreal injections used to dissolve post-vitrectomy fibrin • Used to induce PVDs in rabbit eyes • Pilot study showed creation of PVDs in humans |
Hyaluronidase | • Cleaves glycosidic bonds of hyaluronic acid • Mimics liquefaction by lowering vitreous viscosity | • Mixed ability to induce PVDs in rabbits • Caused retinal necrosis in rabbits and monkeys |
Nattokinase | • Activates plasminogen activator and inactivates an inhibitor | • Induces PVDs in rabbit eyes • Causes retinal hemorrhages and changes in electroretinogram |
Chondroitinase | • Degrades chondroitin sulfate and depolymerases hyaluronic acid • Has liquefactant and interfactant properties | • Detached posterior hyaloid in monkeys after 5 to 15 min |
Plasmin | • Nonspecific protease from plasminogen activation • No activity against type IV collagen | • In DR pilot study (7 eyes), vitreoretinal interface weakened • PVD induced in 10 of 25 DME eyes with improved VA and CRT • PVD induced in 4 of 12 eyes; most had improved VA |
Tissue plasminogen activator (tPA) | • Catalyzes conversion of plasminogen to plasmin | • Induces PVDs in rabbits • PVDs reported in case studies and retrospective series • Prospective, randomized trial failed to show development of PVD |
Autologous plasmin enzyme (APE) | • Easier and less expensive to isolate than plasmin | • 85% of eyes found to have PVD 1 h after injection • Compared to controls, decreased DME and improved VA after 1 month |
Vitreosolve | • Nonenzymatic agent comprised of urea | • Phase III trial failed to show difference between vitreosolve and controls |
Ocriplasmin | • Truncated version of plasmin • Acts on several molecules at vitreoretinal interface | • Phase III MIVT trials, 26.5% with vitreomacular traction achieved PVD • Fewer than 20% of DME eyes achieved vitreoretinal separation in MIVI-II • Phase III trial for DME currently underway |
7.6.1 Streptokinase
Streptokinase, an enzyme produced by several Streptococci sp., binds to and activates human plasminogen. Streptokinase is a fibrinolytic that is used to dissolve clots in patients with acute myocardial infarctions [86] and pulmonary emboli [63]. No adverse effects have been noted after the intravitreal injection of 1000 IU.
Intravitreal streptokinase has been used to dissolve post-vitrectomy fibrin in patients with advanced PDR [11]. Posterior vitreous detachments have been induced in rabbit eyes after the injection of 1500 IU, and only mild toxic effects on the retina have been noted [19]. Pilot studies have been performed to evaluate the efficacy of streptokinase-plasmin injections into human eyes for the induction of PVDs [14]. Though the drug has demonstrated potential for vitreolysis, further studies are required to establish efficacy.
7.6.2 Hyaluronidase
Hyaluronidase cleaves glycosidic bonds of hyaluronic acid and other glycosaminoglycans. This mimics liquefaction by lowering the viscosity of the vitreous. Commercially available hyaluronidase includes a bovine testicular protein enzyme (Wydase®), which has been used in ophthalmology for over 40 years as a spreading or diffusing agent with local anesthetics.
Hyaluronidase has been used in experimental intravitreal models, but its ability to cause a PVD remains unclear. Intravitreal bovine hyaluronidase has produced mixed results in rabbits [35, 44], but retinal necrosis has been noted in both rabbit and monkey eyes [29, 36]. Hyaluronidase has been suggested as a drug to induce a PVD, but since it does not weaken the vitreoretinal interface, there is little evidence that it would work.
7.6.3 Nattokinase
Nattokinase is a 275-amino acid serine protease produced by Bacillus subtilis [64]. Nattokinase has potent fibrinolytic activity as a plasminogen activator and by inactivating a plasminogen activator inhibitor, and it hydrolyzes collagen fibrils [88, 89]. Nattokinase induces a PVD in rabbit eyes 30 min after injection but also causes retinal hemorrhage and ERG changes [92].
7.6.4 Chondroitinase
Chondroitinase degrades chondroitin sulfate and depolymerases hyaluronic acid. A low dose failed to induce PVD in pigs but higher doses detached the posterior hyaloid in monkeys within 5 to 15 min [32]. Chondroitinase remains an attractive molecule for vitreolysis because of both its liquefactant and interfactant properties, but much more studies need to be done to determine its clinical efficacy.
7.6.5 Plasmin
Plasmin is a nonspecific protease resulting from plasminogen activation. Plasmin mediates fibrinolysis and also acts on numerous glycoproteins such as laminin and fibronectin. Plasmin is not believed to alter the intact ILM because it has no activity on type IV collagen. Intravitreal plasmin can create posterior vitreous detachments (dose dependent) in rabbit and pigs [23, 40, 43], and 0.4 IU can induce complete PVDs in postmortem human eyes [24]. Intravitreal autologous plasmin injections have been used as primary or adjunctive therapy for the treatment of pediatric macular holes [62] and advanced diabetic retinopathy [7–9]. It can be prepared in vitro by hydrolyzation with urokinase.
In a pilot study, seven eyes with advanced diabetic retinopathy (six with traction retinal detachment and one with treatment resistant DME) received intravitreal plasmin. The vitreoretinal interface was weakened in all eyes leading to either spontaneous PVD or easier surgical dissection. All eyes achieved resolution of DME without the need for additional laser [99].
In a prospective interventional case study, 25 eyes with clinically significant DME and vitreomacular traction received 0.2 IU/0.2 ml of autologous plasmin. PVD was achieved in ten (41.3%) eyes – complete in six and partial in four. All ten eyes had vitreous separation from the fovea. Visual acuity improved by one Snellen line in four eyes. The mean foveal thickness improved from 480 to 226 μm (P = 0.05) [20].
A prospective study evaluated the efficacy of intravitreal plasmin in 12 eyes with SD-OCT-diagnosed VMT that were injected over the course of 4 years. Five eyes were injected once and seven eyes each received three injections. Four eyes (33%) developed PVDs, two after single injections and two after multiple injections. Central macular thickness improved significantly (P = 0.016), and seven eyes experienced BCVA improvement of at least one line (P = 0.017). The only complication was an immediate elevation in intraocular pressure in one eye [74].
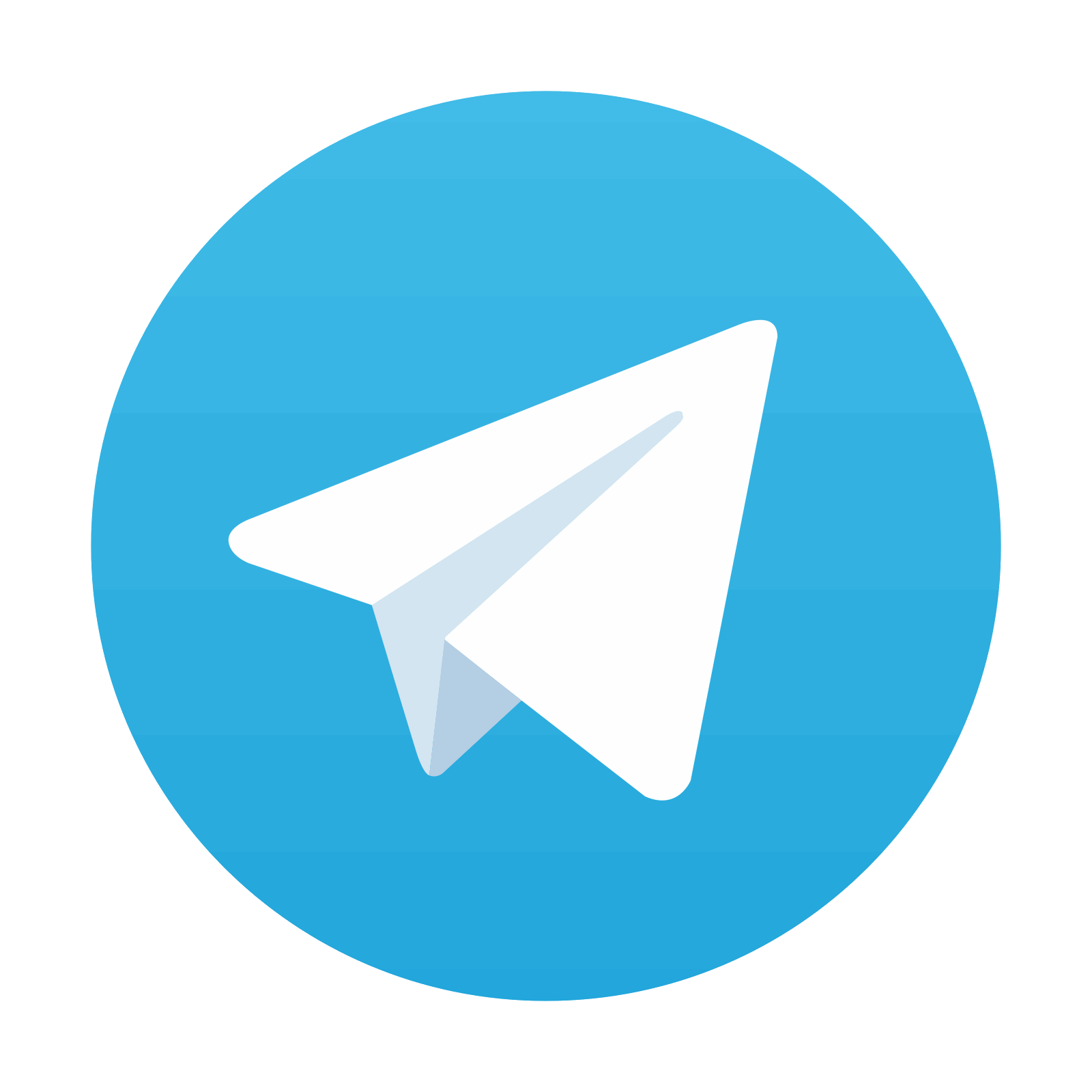
Stay updated, free articles. Join our Telegram channel

Full access? Get Clinical Tree
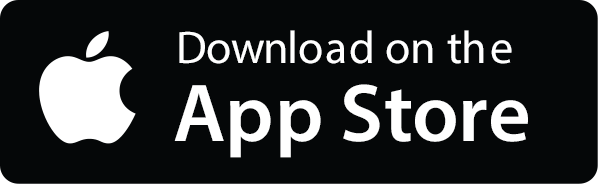
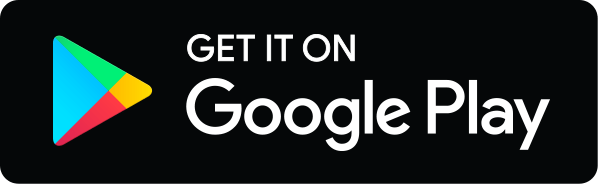