will then accumulate in the liver, specifically in the microsomes.17 Warfarin is a racemic mixture of S and R enantiomers, which are present in almost equal proportions. Warfarin has a half-life of 36 to 42 hours, which is the result of different metabolism rates of the two enantiomers. The R enantiomer is less potent, is metabolized primarily by two microsomal cytochrome enzymes (CYP1A2 and CYP3A4) to 6- and 8-hydroxywarfarin, and has a half-life of 45 hours. The S enantiomer is 2.7 to 3.8 times more potent than the R enantiomer, is metabolized primarily by the CYP2C9 enzyme of the P450 system to 7-hydroxywarfarin, and has a half-life of 29 hours (FIGURE 106.1B).18 The inactive hydroxywarfarins are excreted renally.
Table 106.1 Level of evidence for drug, food, and dietary supplement interactions with warfarin | ||||||||||||||||||||||||||||||||||||||||||||||||||||||||||||||||||||||||||||||||||||||||
---|---|---|---|---|---|---|---|---|---|---|---|---|---|---|---|---|---|---|---|---|---|---|---|---|---|---|---|---|---|---|---|---|---|---|---|---|---|---|---|---|---|---|---|---|---|---|---|---|---|---|---|---|---|---|---|---|---|---|---|---|---|---|---|---|---|---|---|---|---|---|---|---|---|---|---|---|---|---|---|---|---|---|---|---|---|---|---|---|
|
Table 106.2 Distribution of CYP2C9 and VKOR polymorphisms in different racial populations* | ||||||||||||||||||||||||||||||||||||||||||||||||||||||||||||||||
---|---|---|---|---|---|---|---|---|---|---|---|---|---|---|---|---|---|---|---|---|---|---|---|---|---|---|---|---|---|---|---|---|---|---|---|---|---|---|---|---|---|---|---|---|---|---|---|---|---|---|---|---|---|---|---|---|---|---|---|---|---|---|---|---|
|
(FIGURE 106.3).64 With the wide use of the standardized INR, several of its limitations have become apparent (Table 106.3). These include decreased precision when insensitive thromboplastins are used; there is an incorrect assignment of an ISI value by the manufacturer; a different reagent-instrument combination is used than that recommended by the manufacturer; and if an incorrect control PT value is used.45
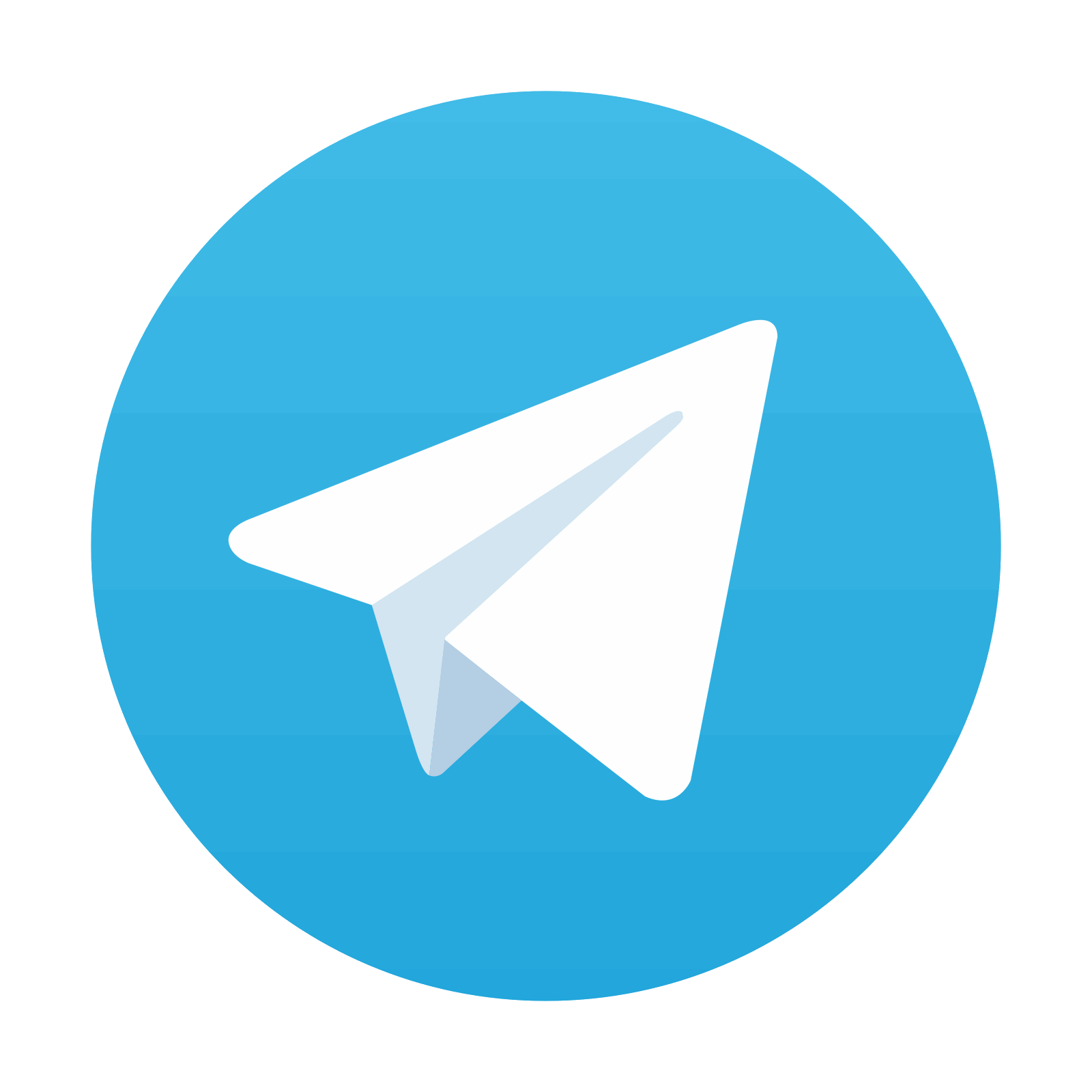
Stay updated, free articles. Join our Telegram channel

Full access? Get Clinical Tree
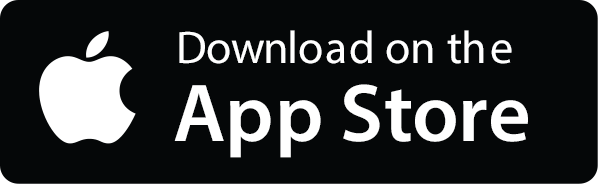
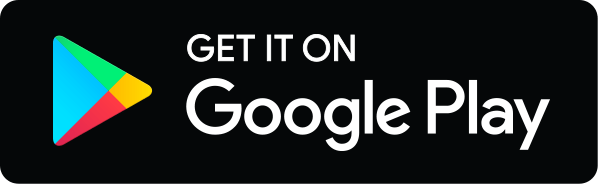
