Fig. 1
HCV genome
The single ORF is surrounded by 5′ and 3′ untranslated regions. Viral proteins have specific functions as shown in Table 1.
Table 1
Key functions of structural and nonstructural HCV proteins
Viral protein | Function |
---|---|
Core | Regulation of translation, replication and viral assembly |
E1 | Viral envelope transmembrane proteins. Viral particle adsorption and endocytosis mediated through membrane receptors |
E2 | |
p7 | Ionic channel in the endoplasmic reticulum needed for infectious viral particle assembly |
NS2 | Protease (NS2–3 cleavage in the precursor polyprotein) |
NS3 | Protease (precursor polyprotein cleavage downstream of NS3 – NS3/NS4A/NS4B/NS5A/NS5B), ATPase, helicase |
NS4A | NS3 protease cofactor |
NS4B | Essential for viral replication. Induces a membranous web within the rough endoplasmic reticulum during replication |
NS5A | Multifunctional phosphoprotein. Harbors the IFN-α sensitivity determining region (ISDR) |
NS5B | RNA polymerase RNA dependent |
1.7 Diagnosis
Serological and molecular tests are available for the diagnosis of hepatitis C [29]. Positive serological results require quantification of HCV RNA in order to differentiate between chronic hepatitis C and resolved past infection. In cases of acute hepatitis C, serological screening by itself is insufficient because the anti-HCV antibodies appear approximately 8 weeks after infection. However, HCV RNA is detectable in at most 2 weeks and therefore molecular testing is critical to the diagnosis of acute hepatitis C, also essential for the indication and duration of treatment success [31].
1.7.1 Serological Assays
The third generation of enzyme immunoassays (ELISA), which includes an NS5 antigen and/or the substitution of a highly immunogenic epitope of NS3 region, allows the detection of anti-HCV antibodies approximately 4–6 weeks after infection with sensitivity higher than 99 %. The newest generation of immunoassays available (“fourth generation”) simultaneously detects HCV capsid antigen as well as antibodies to the core, NS3, NS4, and NS5 regions of the virus. These assays have further reduced the window period of HCV detection to 17 days [11].
False-positive results are more frequent in patients with rheumatic factors and in populations with low prevalence of hepatitis C, for example, blood and organ donors. False-negative results may occur in patients on hemodialysis or in severely immunocompromised patients or those with hematologic malignancies. Immunoblot assays can be used as supplemental assays to confirm serological reactivity by ELISA, but are now more and more replaced by molecular tests [36]. Recent studies have also suggested that the higher the anti-HCV antibody titer in patient’s serum, the more chances of it being true positive than false positive and that repeating this samples with another ELISA test would be enough to confirm its serological status [11].
1.7.2 Molecular Assays
There are various assays from different commercial suppliers for the detection of HCV RNA. Currently, all these assays are carried out using real-time PCR with a very low limit of sensibility and a broad range of quantitation (10–100,000,000 mUI/mL). These assays are particularly useful for diagnosis, particularly for the indication and follow-up of HCV patients under treatment. Similar assays are also available for HCV detection in blood donors [19].
HCV genotypes must be determined, since most of the treatment regimens are differentiated according to the viral genotype. The determination of genotype and subtype of virus can be made based on direct sequencing of genomic regions of HCV (e.g., the 5′ UTR, core, and/or NS5B), reverse hybridization assay, and real-time PCR or sequencing [19].
There are other assays to determine the polymorphisms on the interleukin 28B (or interferon lambda 3) gene or resistance to new drugs utilized for the treatment that have been developed but are not routinely utilized even in most developed countries [5].
1.7.3 Treatment
New therapeutic regimens for hepatitis C utilizing direct-acting antiviral (DAA) drugs that can specifically inhibit viral proteins such as protease (NS3), NS5A, and RNA polymerase (NS5B) have been recently approved, some of them without the use of pegylated interferon or even ribavirin [5]. These treatments are highly effective with more than 90 % of response rates in almost all population groups, even in the presence of HIV coinfection and/or liver cirrhosis. Decisions to shorten, extend, or stop treatment with these regimens may require frequent and accurate quantification of serum HCV RNA levels [19]. This area is constantly changing and should be updated on the websites from international or national liver disease societies, such as AASLD/IDSA (http://www.hcvguidelines.org/) or EASL (http://www.easl.eu/).
2 Hepatocelular Carcinoma
2.1 Epidemiology
Primary malignant liver tumors correspond to the 6th leading cause of cancer and 3rd leading cause of cancer death worldwide. Hepatocellular carcinoma (HCC) corresponds to 85–90 % of primary liver cancers. The estimated global incidence of HCC is 500 thousand to 1 million new cases/year, leading to >500,000 deaths per year worldwide. Today HCC is the most common complication and the leading cause of death in patients with compensated liver cirrhosis. The vast majority of the cases of HCC are associated with liver cirrhosis. It is estimated that chronic infection with hepatitis B virus (HBV) and hepatitis C virus (HCV) are associated to more than 80 % of HCC cases worldwide [15].
HCC is found throughout the world with a very heterogeneous distribution, which is probably related to its etiological factors, such as HBV, HCV, and exposure to aflatoxin B1. Sub-Saharan Africa and East Asia comprise the majority of cases (>80 %) and are considered high-incidence areas. China accounts for approximately 50 % of all cases of HCC worldwide. Japan also has a high incidence of HCC with a case index of approximately 40 per 100,000 people. HCC in Africa is likely underestimated, because of lack of screening and access to medical care in rural areas, and is a major cause of death in the black African population [17].
Conversely, North and South America, as well as Europe, have a comparatively low incidence of HCC. Although the overall incidence of HCC in the United States is lower than in other parts of the world, the age-adjusted incidence rate tripled from 1975 to 2005 from 1.6/100,000 to 4.9/100,000. This increase is likely a result of the increasing prevalence of HCV from unscreened blood transfusions and IV drug use in the 1960s and 1970s, although there are other likely contributing factors. Europe has a slightly higher incidence (2–4 times) of HCC than the United States. The Mediterranean countries (Italy, Spain, and Greece) have incidence rates ranging from 10 to 20 per 100,000 individuals. These countries also attribute approximately two-thirds of their cases to chronic HCV infection [17]. In Latin America, hepatitis C was shown to be the leading risk factor for HCC, found in 38 % of 240 cases [21].
2.2 HCV and HCC
The increased risk of HCC development in HCV-infected patients comes from the development of liver fibrosis and cirrhosis as a result of chronic inflammation. This inflammation leads to the distortion of hepatic architecture and impairment of cellular functions as well as the microcirculation of the liver. The annual risk of developing HCC in patients with cirrhosis is in the range from 1 to 4 %, and about 1 to 3 % of patients chronically infected with HCV develop HCC after 30 years [32]. Unlike HBV, HCV is unable to integrate into the host genome. Instead, in HCV, viral proteins such as HCV core protein and their evoked host response have been implicated in apoptosis, signal transduction, reactive oxygen species (ROS) formation, transcriptional activation, and immune modulation through upregulation of interleukin 1 (IL-1), IL-6, and tumor necrosis factor α (TNF-α), contributing to malignant transformation [24].
There is an increased incidence of HCC observed in the Western population, most of which are related to this virus and for its increasing frequency in an aging population. The association between HCV and HCC is probably resulting from the combination of the independent effect of HCV in hepatocarcinogenesis and immunological indirect effects and the development of cirrhosis. HCV does not have a reverse transcriptase activity and its replication cycle does not lead to integration into the host genome. HCV is a virus that replicates only in the cytoplasm and the main hypothesis for carcinogenesis associated with HCV is through indirect ways, especially by the effects of chronic inflammation and hepatocyte injury. This hypothesis is supported by the fact that the presence of cirrhosis is almost a prerequisite for the development of HCC [10].
On the other hand, for many proteins of HCV, a role in the development of HCC in experimental studies involving cell culture systems and animal models was described. The core protein of HCV is involved in the assembly of the viral particle and generation of complete virions. However, this protein is also involved in cell signaling, transcriptional activation, apoptosis, lipid metabolism, and cell transformation. Indeed, it affects the modulation of cellular gene products and several regulatory pathways involved in cell proliferation, cell cycle control, and tumor formation [18]. In models of transgenic mice, the core protein can induce HCC, although the exact mechanism by this occurs has not yet been fully clarified [23].
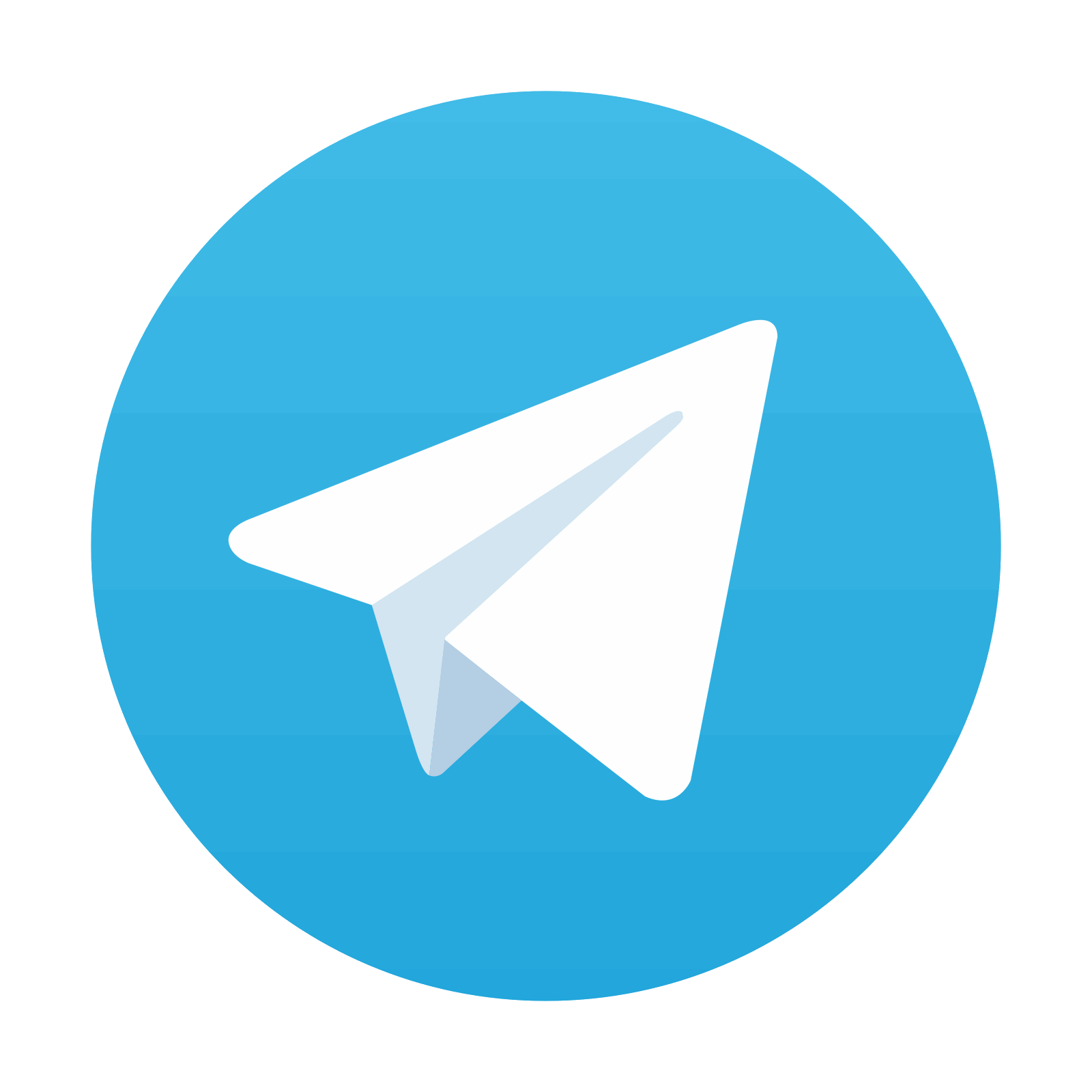
Stay updated, free articles. Join our Telegram channel

Full access? Get Clinical Tree
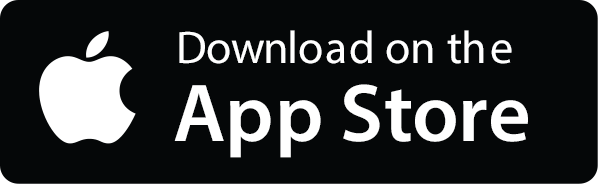
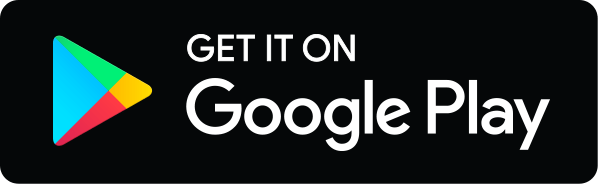