© Springer Science+Business Media New York 2017
William B. Coleman and Gregory J. Tsongalis (eds.)The Molecular Basis of Human Cancer10.1007/978-1-59745-458-2_1919. Viral Mechanisms in Human Carcinogenesis
(1)
McArdle Laboratory for Cancer Research, Department of Oncology, University of Wisconsin School of Medicine and Public Health, 1400 University Avenue, Madison, WI 53706, USA
Keywords
Viral mechanismsCarcinogenesisVirally induced cancerRNA tumor virusesDNA tumor virusesHuman papillomavirusesMerkel cell polyomavirusEpstein–Barr virusKaposi’s sarcoma-associated herpesvirusHuman T-cell leukotropic virus type 1Hepatitis B virusHepatitis C virus19.1 General Principles of Virally Induced Cancer
Infectious agents, including viruses, bacteria, and parasites, are thought to be the etiologic agents in approximately 20 % of human cancers. Human oncogenic viruses include hepatitis B and hepatitis C viruses (associated with hepatocellular carcinoma), Epstein–Barr virus (associated with B-cell lymphomas, nasopharyngeal, and gastric carcinomas), human papillomaviruses (associated with cervical carcinoma, other anogenital cancers, and a subset of head and neck cancers), human T-cell lymphotropic virus I (HTLV-1; associated with Adult T-cell lymphomas), human herpesvirus type 8 (associated with Kaposi’s sarcoma and primary effusion lymphomas), and the newest identified human tumor virus, Merkel cell carcinoma-associated polyomavirus. The initial recognition that viruses cause cancer arose from studies of animal viruses. In the early twentieth century, transmittable agents were demonstrated to cause tumors in chickens and in rabbits. The respective agents were later identified to be Rous sarcoma virus (RSV) , the first studied RNA tumor virus, and Shope papillomavirus, the first studied DNA tumor virus. Much of our understanding of viral oncogenesis derived initially from the study of such animal viruses. In recent years, much attention has been focused on the study of human tumor viruses as etiological roles of such viruses in human cancers have been elucidated. The study of virally induced cancers in animals and in humans has provided many basic insights into cancer, the most important of which is the identification and functional characterization of two fundamental types of genes important in cancer: oncogenes and tumor-suppressor genes. From these collective studies several generalizable principles of virally induced cancer have arisen. First, the onset of virally associated cancer is characterized by long latent periods following initial infection. This suggests that the viruses alone are not sufficient to cause cancer. Also, given this long latency, oncogenic viruses must be able to persist in the host for long periods of time. Second, the cancers induced by viruses do not constitute a natural part of the viral life cycle. Rather, virally associated cancer appears to be a dead-end street for most viruses—by-products of the natural infection that provide no advantage to the virus evidenced by the fact that it is uncommon for progeny virus to be produced in the associated tumors. The third principle is that viral properties that contribute to the induction of tumors often play critical roles in the life cycle of the virus. In the following sections are described the basic properties of RNA and DNA tumor viruses and the major insights gained regarding how they contribute to cancer.
19.2 RNA Tumor Viruses
The field of tumor virology initially arose from the study of retroviruses that cause tumors in birds and mammals other than humans. The oncogenic retroviruses can be divided into three classes: (1) transducing retroviruses, (2) insertionally mutagenic retroviruses, and (3) true oncoretroviruses. Transducing retroviruses are ones that acquire genetic information from the host genome. RSV is one example. Most other examples also were isolated from avian hosts, although others have been isolated from feline, murine, and simian hosts. The cellular genetic information being transduced is a cellular proto-oncogene which, as present in and expressed from the transducing retrovirus, has oncogenic activity, i.e., it contributes to tumor formation in vivo and cellular transformation in tissue culture. The study of transducing retroviruses led to the discovery of more than a dozen oncogenes, including src, ras, and myc. The discovery of these virally encoded oncogenes, and their detailed study helped define numerous cellular signaling pathways that play fundamental roles in normal cell physiology and when altered, in carcinogenesis. It was not recognized until the 1970s that these viral oncogenes actually had been picked up by the virus from the host organism through genetic recombination, which arises as a by-product of reverse transcription. This led to the recognition that animals encode oncogenes. The cellular copies of these genes were initially referred to as proto-oncogenes because it was recognized that the viral copies of these genes had undergone genetic changes that led to the increased expression and/or activity of the encoded gene product. These changes led to the oncogenic nature of the virally transduced gene. In contrast, the cellular homologs do not contribute to cancer in their wild-type state. For example, the virally encoded src and ras genes have amino acid substitutions at positions in these proteins that lead to their constitutive activity in signal transduction pathways, and the virally encoded myc gene is missing regions of the cellular gene that normally lead to a tight regulation of its expression at the transcriptional and post-transcriptional levels. No examples of transducing retroviruses have been found for human cancers; rather, most such viruses were identified from the evaluation of laboratory stocks of retroviruses that infect laboratory animal species. Generally, these transducing, oncogenic retroviruses are replication-defective because of the disruption of one or more essential viral gene by the insertion of the cellular proto-oncogene. The rare exception is RSV, which is a replication-competent virus in which the transduced cellular src gene did not disrupt function of any viral gene. How a transducing oncogenic retrovirus arises remains unclear. It likely results from a provirus that integrates close to a cellular proto-oncogene. This could result in the formation of a chimeric RNA of viral and cellular origin which, when packaged into progeny retrovirus and on reverse transcription, undergoes recombination with a co-packaged, intact viral transcript to produce the actual transducing viral genome.
Insertionally mutagenic retroviruses are ones that, as a consequence of insertion of the proviral genome into the host chromosomes, alter the structure and/ or expression of a cellular proto-oncogene or tumor suppressor gene. These retroviruses are also known as the weakly oncogenic retroviruses since they only rarely cause cancers in laboratory animals, reflective of the low likelihood that the provirus insertion occurs at or near a cellular proto-oncogene or tumor suppressor gene. There are several mechanisms by which insertion of provirus can lead to activation of a cellular proto-oncogene. First, the virus may integrate upstream of the cellular gene in the same sense orientation such that read-through transcription from the viral promoter leads to the generation of chimeric viral:cellular mRNAs that can encode the cellular proto-oncogene. Second, the viral transcriptional enhancer can upregulate the activity of the cellular gene’s own transcriptional promoter. Finally, the virus can disrupt the integrity of the cellular mRNA leading to the loss of negative regulatory elements such as mRNA instability elements in the mRNA encoding the cellular protooncogene. Examples of each of these three cases exist in the literature. Many of the insertionally mutagenic retroviruses cause lymphomas/leukemia in their natural host. In these tumors, the provirus is found nearby to the c-myc locus, leading to increased expression of the c-myc gene product. Insertionally mutagenic retroviruses can also integrate within alleles of tumor suppressor genes and disrupt their expression, thereby leading to cancer. The identification of such events is much less frequent than those that lead to the activation of cellular proto-oncogenes, presumably because of haplosufficiency.
True oncoretroviruses are ones that, through virally encoded activities, alter the host cell or the host cell environment, thereby making cells more susceptible to cancer development. There are only a few cases of true oncoretroviruses. One is HTLV-1, which causes T-cell lymphomas in humans.
19.3 DNA Tumor Viruses
Whereas the study of RNA tumor viruses was largely responsible for the discovery of oncogenes, the study of DNA tumor viruses led to the discovery and elucidation of the function of cellular tumor suppressor genes, most notably p53 and pRb. Much of the initial knowledge about DNA tumor viruses came from the study of SV40, a simian virus. SV40 efficiently transforms cells in tissue culture and can cause tumors in hamsters, but not its natural host. Several viral gene products were found expressed in these transformed/tumorigenic cells and were identified because hamsters made antibodies against them. Most notable of these was the SV40 large tumor antigen (TAg). This protein is one of the most potent oncogenic factors known [1]. Its oncogenic activity is multifactorial, largely arising from its ability to bind and inactivate the cellular tumor-suppressor proteins p53 and pRb. p53 is now recognized to be functionally disrupted in most human cancers. The protein was originally identified by virtue of its association with SV40 TAg. Initial cloning and functional analysis of p53 led to its designation not as a tumor suppressor gene but an oncogene. This was due to the fact that the initial p53 clones were isolated from cell lines, i.e., populations of cells that had acquired an immortalized phenotype. In these cell lines the p53 gene had undergone mutations that cause the encoded gene product to possess dominant-negative activity; that is, it could inactivate the normal p53 protein when put back into cells. It was not until the late 1980s that the wild-type p53 gene was recognized to be a tumor-suppressor gene [2]. p53 is thought to play an important role in orchestrating the cellular responses to certain forms of stress, including insult from DNA-damaging agents. Activation of p53 leads either to growth arrest, which is believed to allow cells time to repair damaged DNA, or apoptosis (programmed cell death). Without functional p53, cells have a greater propensity for undergoing genetic alterations, some of which can contribute to tumorigenesis. It is now understood that when SV40 TAg binds the wild-type p53 protein, it inactivates the latter’s function [1]. SV40 TAg also can bind the gene product of the retinoblastoma tumor susceptibility locus (Rb) [3]. Rb and the related pocket proteins, p107 and p130, are critical regulators of the cell cycle. In the G1 stage of the cell cycle pRb binds to and inactivates a family of transcription factors, E2Fs, that regulate the activity of cellular genes involved in DNA synthesis and cell cycle control. Normally, pRb’s activity is regulated by cyclin-associated kinases, which phosphorylate pRb and lead to its dissociation from E2Fs. TAg upon binding to pRb or its relatives p107 and p130 can also cause this dissociation and thereby lead to cell cycle deregulation.
The insights gained from the study of SV40 provided many insights about how human papillomaviruses cause carcinoma of the anogenital tract and head/neck region in humans, and potentially also shed light on the oncogenic activities of the recently discovered Merkel cell carcinoma associated polyomavirus. However, it would be an oversimplification to think that targeting p53 and pRb are the only means by which DNA tumor viruses transform cells or cause tumors in organisms. Much like what was learnt from the study of transducing oncoretroviruses that express mutated forms of cellular proto-oncogenes, there are many varied cellular pathways targeted by DNA tumor viruses that, when altered, contribute to transformation in tissue culture and tumorigenesis in vivo.
19.4 Human Tumor Viruses
19.4.1 Human Papillomaviruses
Papillomaviruses are small DNA viruses that infect the epithelial lining of their host and for the most part cause benign proliferative lesions such as warts that support production of progeny virus. There are over 100 defined human papillomavirus genotypes that can be subdivided into two classes, those that primarily infect cutaneous epithelia such as the epidermis lining the hands and feet, and those that primarily infect mucosal epithelia including that lining the anogenital tract and the oral cavity. The latter, mucosotropic HPVs, are the most common sexually transmitted pathogens in humans; greater than half the human population have been exposed to these HPVs in their lifetime. A subset of these mucosotropic HPVs that cause human cancers such as cervical cancer, anal cancer, and head and neck cancers. The recent generation of highly efficacious, prophylactic vaccines against two of the most common of these cancer-causing HPV genotypes, HPV16 and HPV18, provides the potential of reducing the incidence of human cancers caused by these viruses. However, their impact on global health is significantly limited by their availability, largely for socio-economical reasons.
Papillomaviruses are non-enveloped icosahedral viruses that contain a double-stranded DNA genome of about 8000 bp that encodes early and late genes. These viruses infect the poorly differentiated basal cells within stratified epithelium wherein the viral genome is established as a low copy, nuclear plasmid and a subset of viral genes are expressed The papillomavirus life cycle is intricately tied to the differentiation program of the host tissue. Progeny virus are only produced when the infected basal cell undergoes cell division and a daughter cell initiates terminal differentiation. This triggers amplification of the viral genome and expression of structural genes that encode the capsid proteins resulting in the production of progeny virus. The virus must manipulate the differentiating epithelia to facilitate its own life cycle. Many of these manipulations to the differentiating host cell are caused by the same viral genes that contribute to cancer.
Professor Harald zur Hausen and his colleagues at the German Cancer Center in Heidelberg are responsible for first uncovering the etiological role of HPVs in human cancer when they reported the presence of DNA of specific HPV genotypes in human cervical cancers [4]. This revelation earned Dr. zur Hausen the Nobel Prize in Medicine. A causal role of these same HPVs has since been established in other anogenital cancers including that of the vagina, vulva, penis, and anus, as well as the head and neck region, particularly the oropharynx. In many of these cancers, the viral DNA is found integrated into the cellular genome and no progeny virus is produced. The viral integration event almost always leads to a disruption in the E2 translational open reading frame (ORF). The E2 ORF encodes proteins involved in viral transcription and replication and its disruption leads to derepression of the viral transcriptional promoter that directs expression of the viral oncogenes, E6 and E7 [5]. Integration also disrupts an mRNA instability element, and this also facilitates increased expression of E6 and E7 [6]. Even in cancers where the genome remains extrachromosomal, E6 and E7 expression is reported.
E6 and E7 are directly implicated in oncogenesis. E7 can cooperate with E6 or the activated ras oncogene to transform fibroblasts in tissue culture, and E6 and E7 contribute to the immortalization of epithelial cells in tissue culture [7]. The expression of E6 and E7 was shown to be required for the continued proliferation of HPV-positive cervical and head/neck cancer cell lines; thus, E6 and E7 are thought to be required for the onset as well as persistence of HPV-associated cancers. When the expression of E6 or E7 is directed to stratified squamous epithelia of transgenic mice, including the epidermis, the mice exhibit thickening of the skin and spontaneously develop squamous cell carcinomas [8]. When treated persistently with exogenous estrogen, these same mice, which also E6 and E7 in the epithelia lining the cervix and vagina, develop cervical and vaginal cancers (whereas nontransgenic animals do not) [9]. Furthermore, these mice are more susceptible than nontransgenic mice to head and neck cancers when treated with an oral carcinogen, again owing to the expression of viral oncogenes in the epithelia lining the oral cavity, oropharynx, and esophagus [10].
Even though E6 and E7 are well accepted to be the main oncogenes for HPV, more recent evidence implicates the E5 viral protein as a possible contributor to carcinogenesis, at least in the cancers where its expression is retained (integration of the viral genome in the host chromosome often leads to a reduced expression of E5). E5 is the main transforming oncogene of bovine papillomavirus (BPV). BPV E5’s transforming activity correlates with its ability to activate the PDGF receptor in a ligand independent manner [11]. E5’s role in HPV-associated cancers is less clear. In tissue culture HPV E5 acts as a weak oncogene. Nevertheless, a large fraction of cervical cancers still express E5. In recent studies, transgenic mice expressing E5 were found to be more susceptible to skin and cervical cancers implicating its role in HPV-associated human cancers [12]. The mechanism underlying HPV E5’s role in cancers remains unclear.
As is the case with SV40 and adenoviruses, papillomaviruses have the ability to inactivate tumor suppressors. E7 binds to and induces the degradation of the tumor suppressor, pRb [7, 13]. The inactivation of pRb allows E2F transcription factors to be constitutively active, which likely accounts in large part for the increased proliferation seen in E7-expressing cells/tissues. The regions of E7 required for its inactivation of pRb have been mapped to conserved regions (CR) 1 and 2, so named because of their similarity to pRb-binding regions in the adenovirus oncoprotein E1A [7]. The CR1 and CR2 domains of E7 are both necessary for E7’s induction of tumors in animals and transformation of cells. An exception is that when the CR1 and CR2 domains of E7 are mutated in the context of the whole papillomavirus genome, cells transfected with the genome still undergo transformation [14]. This suggests that E7 possesses transforming activities mediated by other activities besides inactivation of pRb. In vivo studies have confirmed that E7’s carcinogenic phenotypes both in the cervix and the head and neck of the mouse cannot be attributed merely to its inactivation of pRb. E7 also inactivates proteins related to pRb, p107 and p130, which like pRB modulate the activities of E2F transcription factors. Studies now underway suggest that inactivation of both pRb and either p107 or p130 increases the susceptibility of mice to cervical and head/neck cancers in mice. E7 also can bind to and inactivate p21 and p27, inhibitors of cyclin-associated kinases that play critical roles in the control of the cell cycle [15, 16]. E7’s inactivation of p21 has now been demonstrated to contribute to its oncogenic potential in the cervix [17]. Thus E7 causes cancer at least in part through its ability to disrupt the function of multiple cellular factors that are involved in the regulation of the cell cycle. E7 is known to associate with over 100 cellular factors in addition to the ones described above. Which of these interactions also contribute to E7’s oncogenic potential remains unclear [18].
The discovery of the ability of E6 to inactivate the tumor suppressor, p53, was prompted by the oncogenic similarities between papillomaviruses and SV40 and adenovirus. E6 was found to form a tertiary complex with p53 and a cellular ubiquitin ligase, E6-AP [19, 20]. This leads to the ubiquitination of p53, which is then targeted for proteasomal degradation. The p53 tumor suppressor is involved in the G1 to S phase transition of the cell cycle and is induced in response to cellular stresses such as DNA damage. Although the importance of p53 in other cancers suggests that it is important in papillomavirus-associated cancers, inactivation of p53 cannot fully substitute for the activities of E6. For example, E6 was found to abrogate differentiation of skin epithelial cells in tissue culture. However, when the p53 in these cells was inactivated by overexpressing a dominant-negative form of p53, the cells could still differentiate. Also, p53-null mice differ from E6 transgenic animals in that they do not display thickened skin or spontaneously develop skin tumors. The alpha-helix-binding domain of E6, through which E6 associates with E6AP has been shown to be important for E6 oncogenic activities in mice, suggesting that the inactivation of p53 contributes to this process. Consistent with these results, E6 is unable to cause cervical cancers on an E6AP-null background [21].
E6 has been shown to bind a variety of protein partners, including E6-BP, paxillin, E6-TP, bak, and the human homolog of Drosophila disc large (DLG) tumor-suppressor gene [22]. The ability of E6 to associate with proteins correlates with its ability to induce aberrant DNA synthesis, transform cells in tissue culture, and contribute to carcinogenesis in mice. The binding partner responsible for those phenotypes has not been identified but candidates include Dlg, Scribble, and MUPP1, all of which have been reported to bind E6 through the PDZ domain. Interestingly, E6 causes destabilization of these PDZ proteins much like it does p53, and this is at least in part mediated by E6AP.
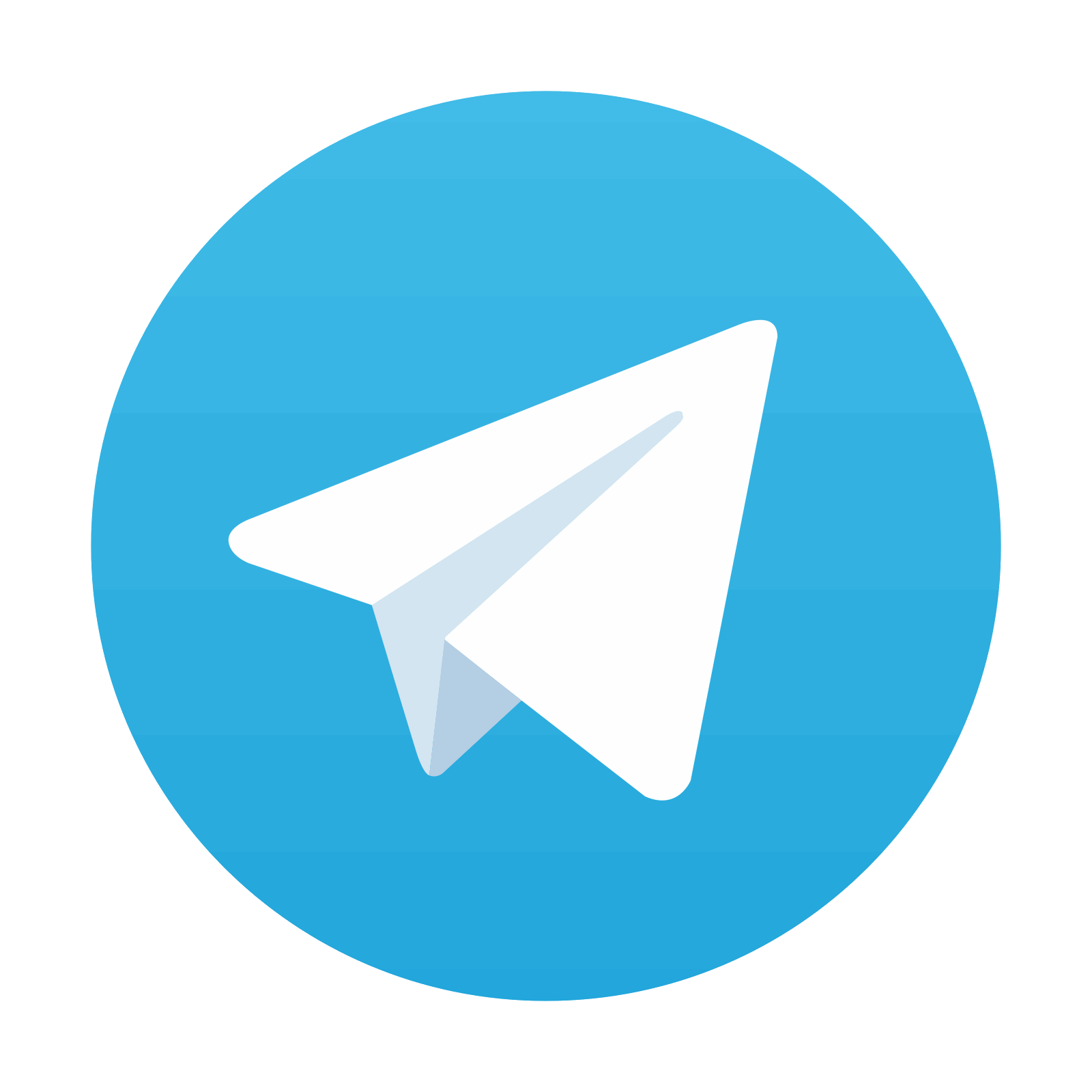
Stay updated, free articles. Join our Telegram channel

Full access? Get Clinical Tree
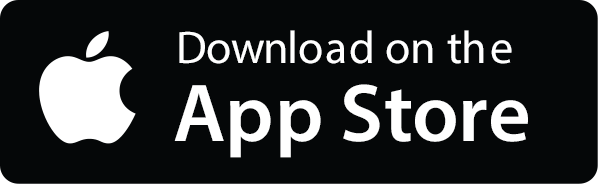
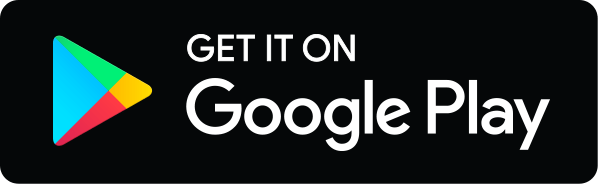