Treatment of Hypothyroidism
Jacqueline Jonklaas
Historical Perspective on the Treatment of Hypothyroidism
The medicinal use of animal organs, glands, and viscera originated in ancient times. Seventhcentury Chinese reportedly used both dried thyroid glands and seaweed for the treatment of goiter (1). Thyroid hormone administration is thus one of the oldest endocrine therapies. Use of thyroid extract was formally reported in the English literature in the latter part of the 19th century, beginning with a description of subcutaneous injection of an extract of sheep thyroid glands by George Murray in 1891 (2). Treatment of hypothyroidism with orally administered thyroid extract was described shortly thereafter (3,4). The bioactive substance, initially named thyroxin, was subsequently isolated in 1914 by Kendall (5). Synthesis of thyroxine (T4) and its use for treating two patients with hypothyroidism was reported in 1927 (6). By 1949 the sodium salt of levothyroxine (LT4), which is better absorbed than T4 itself, had been synthesized (7). It was probably not until the 1970s that synthetic LT4 had replaced desiccated porcine or bovine thyroid preparations as the most commonly employed therapy. On the basis of a study of drugs prescribed and dispensed during the period 1960 to 1988, 84% of individuals taking thyroid hormone were taking LT4 by the end of this period (8). Currently, LT4 is considered to be the treatment of choice for hypothyroidism (9), and most of the patients with hypothyroidism in the United States are treated with LT4 as a single agent. Replacement of thyroid hormone in an individual diagnosed with hypothyroidism, when first considered, appears to be a simple undertaking. However, the clinician today faces multiple issues including what type of thyroid hormone preparation to use, how to consistently maintain therapeutic efficacy, what constitutes euthyroidism, and what are the appropriate therapeutic endpoints for each of the patient groups being replaced with thyroid hormone.
Table 46.1 Percentage Change in LT4 Dose Achieved By Titrating Commonly Available Doses | |||||||||||||||||||||||||||||||||||||||||||||||||||||||
---|---|---|---|---|---|---|---|---|---|---|---|---|---|---|---|---|---|---|---|---|---|---|---|---|---|---|---|---|---|---|---|---|---|---|---|---|---|---|---|---|---|---|---|---|---|---|---|---|---|---|---|---|---|---|---|
|
Thyroid-Stimulating Hormone as an Indicator of Thyroid Status
The classic endocrine negative feedback loop between the pituitary and the thyroid gland is characterized by an inverse relationship between the serum concentrations of
thyroid-stimulating hormone (TSH) and thyroid hormones. TSH is the most robust, sensitive, and accepted clinical indicator of thyroid status (10). LT4 is known to be a medication with a narrow therapeutic to toxic ratio (11). There are significant clinical consequences when LT4 treatment is excessive or inadequate, so avoidance of long-term iatrogenic hypothyroidism or hyperthyroidism is therefore desirable (see section The TSH Goal of Thyroid Hormone Therapy). Thus, the goal of LT4 treatment is generally to maintain patients with a stable and normal TSH level (9,10,12). Serum TSH levels are exquisitely sensitive to changes in circulating thyroid hormone levels (10,11) and maintenance of a patient’s prescribed LT4 dose should result in fairly consistent TSH levels. Conversely, changes in LT4 dose will result in perturbations in a patient’s serum TSH level. This was shown in a study in which individual patients experienced significant changes in their TSH levels with small changes in their LT4 dose (11). Fine-tuning of serum TSH levels is possible because of the range of LT4 dosages that can be obtained in the United States. These doses range from 25 to 300 mcg, with 12 different doses being available. The percentage change in LT4 dose, as one makes incremental or decremental changes using the 10 different LT4 tablets shown (Table 46.1), ranges from 9% to 50%. A patient’s serum TSH can thus be regulated by titrating the LT4 dosage, until the desired TSH endpoint is achieved. Continued therapy with this correct dose should then maintain the desired TSH, if other potentially interfering factors are held constant.
thyroid-stimulating hormone (TSH) and thyroid hormones. TSH is the most robust, sensitive, and accepted clinical indicator of thyroid status (10). LT4 is known to be a medication with a narrow therapeutic to toxic ratio (11). There are significant clinical consequences when LT4 treatment is excessive or inadequate, so avoidance of long-term iatrogenic hypothyroidism or hyperthyroidism is therefore desirable (see section The TSH Goal of Thyroid Hormone Therapy). Thus, the goal of LT4 treatment is generally to maintain patients with a stable and normal TSH level (9,10,12). Serum TSH levels are exquisitely sensitive to changes in circulating thyroid hormone levels (10,11) and maintenance of a patient’s prescribed LT4 dose should result in fairly consistent TSH levels. Conversely, changes in LT4 dose will result in perturbations in a patient’s serum TSH level. This was shown in a study in which individual patients experienced significant changes in their TSH levels with small changes in their LT4 dose (11). Fine-tuning of serum TSH levels is possible because of the range of LT4 dosages that can be obtained in the United States. These doses range from 25 to 300 mcg, with 12 different doses being available. The percentage change in LT4 dose, as one makes incremental or decremental changes using the 10 different LT4 tablets shown (Table 46.1), ranges from 9% to 50%. A patient’s serum TSH can thus be regulated by titrating the LT4 dosage, until the desired TSH endpoint is achieved. Continued therapy with this correct dose should then maintain the desired TSH, if other potentially interfering factors are held constant.
Choice of Thyroid Hormone Therapy
Monotherapy with Levothyroxine
LT4 is a convenient therapy for hypothyroidism. Monotherapy with this product is the most commonly employed treatment for hypothyroidism today. LT4 is chemically stable with relatively modest product deterioration during storage. The manufacturing process is expected to maintain consistent potency and minimize batch-to-batch variability through standardization and quality control. Absorption after oral administration is acceptable and the half-life of a week allows once daily dosing. LT4, as the sodium salt of T4, is actually a prohormone and is converted into the active triiodothyronine (T3), which binds to either the alpha or beta forms of the thyroid hormone receptor. The T3-receptor complex interacts with target genes and regulates gene transcription. The different isoforms of the receptor allow tissue specific actions of T3 to ensue. The ratio of T4 to T3 in thyroid glandular secretion is approximately 15:1 (13). Multi-compartment analyses in humans with normal thyroid function show that 100 mcg of T4 and 29 mcg of T3 are produced daily (14). T4 is secreted by the thyroid gland itself. T3 primarily results from deiodination of T4 to T3 in the liver and peripheral tissues (23 mcg), with the remainder (6 mcg) being generated from thyroidal production, in proportions of 80% and 20%, respectively.
When LT4 therapy is administered to individuals with hypothyroidism and little or no endogenous thyroid function, T3 is generated solely by deiodination of T4 in peripheral tissues. Relatively stable serum concentrations of both T4 and T3 are achieved (15,16). It is clear that in athyreotic patients taking LT4, the serum T4 levels required to maintain a normal TSH are higher than the T4 levels seen in euthyroid subjects (17,18,19,20). This results in a higher T4/T3 ratio in LT4-replaced patients. However, there are conflicting data regarding whether comparable T3 concentrations are achieved. Several studies (18,19,21,22), including one using patients as their own controls (20), suggest that serum T3 levels are maintained in LT4-treated patients (see Fig. 46.1). Other studies have demonstrated that serum T3 levels were lower in patients taking LT4 (17,23).
![]() Figure 46.1 A: Serum TSH at four time points for patients treated for post-surgical hypothyroidism (TSH 1 and 2 are prior to thyroidectomy and TSH 3 and 4 are while taking replacement LT4). B: Serum total T3 at four time points for patients treated for post-surgical hypothyroidism (T3 1 and 2 are prior to thyroidectomy and T3 3 and 4 are while taking replacement LT4). Reproduced with permission from ref. 20. |
Levothyroxine Products
Many different LT4 products are available that are thought to have different absorption because of different excipients (color agents and fillers) (24,25,26). Bioequivalence is a measure of absorption or bioavailability that the Food and Drug Administration (FDA) uses to predict therapeutic efficacy. Bioavailability is determined by studying the pharmacokinetics of large oral doses of LT4 in volunteers who have normal endogenous thyroid function. Doses of 400 to 600 mcg of the LT4 products to be tested are administered and the maximum serum concentration (Cmax), time to Cmax, and area under the concentration–time curve (AUC) are determined (Fig. 46.2A) (27). Two products are studied in a cross-over design. The AUC and Cmax are logarithmically transformed. If the two 90% confidence intervals from the natural logarithms of the AUC at 48 hours and the Cmax are both within the 80% to 125% range, the products are deemed bioequivalent by the FDA (Fig. 46.2B), and can be substituted for each other. Measures of systemic exposure (AUC, Cmax) are used in this testing, rather than using clinical endpoints such as serum TSH levels (28). Representatives of the major endocrine societies have expressed their concern about this methodology (29,30). The concerns voiced include the inability to study serum TSH because of the use of supraphysiologic doses of LT4 and the fact that the situation does not reflect steady state conditions. In addition, bioequivalence
methodology appears not to be capable of detecting LT4 doses with 12.5% difference from each other (26). Thus, referring to Table 46.1, an increase in dose from 100 to 112 mcg (12%) and a decrease in dose from 100 to 88 mcg (12%) may not be detected. Differences in dosage strengths in the 25% range are known to produce clinically relevant changes (11), and potentially result in iatrogenic hyperthyroidism or hypothyroidism.
methodology appears not to be capable of detecting LT4 doses with 12.5% difference from each other (26). Thus, referring to Table 46.1, an increase in dose from 100 to 112 mcg (12%) and a decrease in dose from 100 to 88 mcg (12%) may not be detected. Differences in dosage strengths in the 25% range are known to produce clinically relevant changes (11), and potentially result in iatrogenic hyperthyroidism or hypothyroidism.
Combination Therapy
An additional issue with respect to treating patients with hypothyroidism is whether tissue levels, rather than simply serum levels, of T3 are normal in patients receiving replacement with T4 alone. A relative T3 deficiency might prompt consideration of combination therapy. If combination therapy with both T4 and T3 is considered, options for delivering such therapy include use of liotrix, which contains T4 and T3 in a 4:1 ratio, and addition of liothyronine to LT4 in doses and ratios selected by the prescribing physician. If the intention were to replicate the molar ratio of T4:T3 achieved by endogenous thyroid functioning in humans, the prescribed ratio would be approximately 15:1 (13,14).
A study performed in rodents showed that with intravenous infusion of T4 alone, tissue levels of T3 were lower in some tissues than in control animals (31). However, with an infusion of both T4 and T3, levels of T3 could be normalized in all tissues (32). A study in euthyroid patients undergoing thyroidectomy, who were subsequently treated with T4 monotherapy, showed that serum T3 concentrations were maintained in the pre-thyroidectomy range (20). However, patient satisfaction, patient performance, and tissue indices of thyroid status were
not examined. Many studies of hypothyroid patients examining the issue of combination therapy have been performed since an initial study in 1970 (33). For example, in one trial of combination therapy, LT4 alone was compared with combination therapy by substituting 50 mcg of T4 with 7.5 mcg of T3 (34). This study demonstrated no beneficial changes in body weight, serum lipid levels, hypothyroid symptoms as measured by a health-related quality of life questionnaire, and standard measures of cognitive performance. The available studies (33,34,35,36,37,38,39,40,41,42,43) had variable designs with respect to the outcomes studied, the use of cross-over or parallel groups, blinding methodology, and the ratio of T4 to T3 employed. However, autoimmune hypothyroidism was the predominant diagnosis in most. With minor exceptions, or exceptions within subgroups (35,40,43), these studies failed to demonstrate a benefit of T3 combination therapy. The exceptions included patient preference for combination therapy (35,39,40) and improved mood scores and measures of cognition (35,40,43). Two meta-analyses of the 11 or so accumulated studies have also concluded that there is no advantage to combination therapy (44,45). An additional study published after the completion of these meta-analyses, however, did report that combination therapy had a favorable effect on quality of life and was preferred by patients (46). Simulation studies of thyroid hormone replacement after thyroidectomy using a nonlinear feedback system on the basis of human data suggest that either combination therapy with T4/T3 or T4 therapy alone could each normalize plasma and tissue T3 levels. The daily T4 doses that were predicted were 103 mcg and 162 mcg, respectively (16).
not examined. Many studies of hypothyroid patients examining the issue of combination therapy have been performed since an initial study in 1970 (33). For example, in one trial of combination therapy, LT4 alone was compared with combination therapy by substituting 50 mcg of T4 with 7.5 mcg of T3 (34). This study demonstrated no beneficial changes in body weight, serum lipid levels, hypothyroid symptoms as measured by a health-related quality of life questionnaire, and standard measures of cognitive performance. The available studies (33,34,35,36,37,38,39,40,41,42,43) had variable designs with respect to the outcomes studied, the use of cross-over or parallel groups, blinding methodology, and the ratio of T4 to T3 employed. However, autoimmune hypothyroidism was the predominant diagnosis in most. With minor exceptions, or exceptions within subgroups (35,40,43), these studies failed to demonstrate a benefit of T3 combination therapy. The exceptions included patient preference for combination therapy (35,39,40) and improved mood scores and measures of cognition (35,40,43). Two meta-analyses of the 11 or so accumulated studies have also concluded that there is no advantage to combination therapy (44,45). An additional study published after the completion of these meta-analyses, however, did report that combination therapy had a favorable effect on quality of life and was preferred by patients (46). Simulation studies of thyroid hormone replacement after thyroidectomy using a nonlinear feedback system on the basis of human data suggest that either combination therapy with T4/T3 or T4 therapy alone could each normalize plasma and tissue T3 levels. The daily T4 doses that were predicted were 103 mcg and 162 mcg, respectively (16).
Rat and human thyroid physiology differ with respect to the molar ratio of T4:T3, the proportion of thyroid hormones bound to transport proteins, and presumably the regulation of tissue thyroid hormone concentration by deiodinases. Putting aside species differences, the rodent studies cited above (31,32) might suggest that local production of T3 is regulated in a tissue-specific manner, and that specific tissues may have differential dependence on circulating T3 levels on the basis of their deiodinase activity (see discussion in Chapter 7). The type 2 deiodinase enzyme is responsible for the conversion of T4 to T3 in many tissues, and several polymorphisms in the type 2 deiodinase gene have been described in humans. The Thr92Ala polymorphism, which is associated with a threonine to alanine substitution at codon 92, does not lead to altered thyroid hormone concentrations. A recent analysis, which examined the impact of combination therapy according to the type 2 deiodinase polymorphism status of the treated patients, suggested that individuals harboring the Thr92Ala polymorphism had a poorer psychological response to LT4 therapy and a better response to combination therapy with both T4 and T3 (47). A smaller study had previously failed to show this differential response, but may have been underpowered (48). Thus, it is possible that individuals with the Thr92Ala polymorphism, which is present in 16% of the population, may account for the subset of patients who do not derive the expected benefit from T4 monotherapy. A prospective trial designed to test this hypothesis has not yet been reported.
Thyroid Extracts (Thyroid USP)
Desiccated thyroid extracts, described as “the cleaned, dried, and powdered thyroid gland,” derived from domesticated animals (hog, beef, or sheep) are preferred by some patients. Thyroid extracts from porcine sources are the most commonly available. The United States Pharmacopeia (USP) requires thyroid extracts to contain approximately 38 mcg (±15%) of T4 and 9 mcg (±10%) of T3 for each 60 to 65 mg (1 grain) tablet. As an animal protein–derived product, thyroid extracts have a characteristic odor and in theory could be antigenic. Although allergy or sensitivity to thyroid extract may be anecdotally described, this problem has rarely been reported (49). Such products may be associated with problems such as variable supply and batch-to-batch variability in the non-thyroid hormone constituents of the animal glands. In addition, it is difficult to maintain euthyroidism in treated patients due to the high proportion of T3 in the thyroid USP preparations (T4:T3 ratio of 4:1). Even though desiccated thyroid is inexpensive, its limitations, particularly the non-physiologic proportion of T3, preclude it from being considered a drug of choice for hypothyroid patients.
Liothyronine Therapy
Options available for T3-based therapy are the previously mentioned liotrix and thyroid USP, which both contain T3 and T4 in a ratio of approximately 1:4, and liothyronine sodium, which is T3 alone. T3 is well absorbed in the gastrointestinal tract and liothyronine is available in three dosages of 5, 25, and 50 mcg. The major disadvantage of T3 therapy is that, due to its short half-life of 1 day, its use is associated with peaks and troughs in its serum concentration (15), unless it is administered multiple times daily. This is in contrast to the steady T3 concentrations that are achieved with T4 therapy (15,16). Peak serum levels, which are attained within 4 hours of administration, can be associated with hyperthyroid symptoms such as anxiety, tremors, and palpitations. Recently, a small trial compared serum TSH concentrations achieved with either T4 or T3 therapy each delivered three times daily (50). Relatively stable T4 and T3 concentrations and similar TSH concentrations could be achieved, but a lengthy adjustment period, with a mean duration of 32 weeks, was required to achieve the stated TSH goal of 0.5 to 1.5 mIU/L, perhaps suggesting that adherence to a thrice daily regimen was difficult. This study demonstrated that 40 mcg T3 or 115 mcg T4 (1:3 ratio) produced similar mean TSH concentrations of 1.4 and 1.2 mIU/L.
Table 46.2 Drugs That May Alter the Levothyroxine Dose Required By A Patient By Affecting T4 Metabolism or Transport | ||||||||||||||||||||||||||||||||
---|---|---|---|---|---|---|---|---|---|---|---|---|---|---|---|---|---|---|---|---|---|---|---|---|---|---|---|---|---|---|---|---|
|
Table 46.3 Patient Characteristics That May Affect the Levothyroxine Dose Required By A Patient | ||||||||||||||||||||||||||||||||||||
---|---|---|---|---|---|---|---|---|---|---|---|---|---|---|---|---|---|---|---|---|---|---|---|---|---|---|---|---|---|---|---|---|---|---|---|---|
|
Sustained Release Liothyronine Therapy
Therapy with sustained release T3 is postulated to be an attractive option (51,52) as it might ensure adequate T3 concentrations at the tissue level, albeit bypassing the opportunity for regulation by type 2 deiodinases. Use of T4/T3 combination therapy containing an in-house sustained release T3 preparation was described in a 2004 publication (53). Subjects had similar TSH values during T4 therapy and during combination therapy, and the combination therapy containing the sustained release T3 achieved steady maintenance of concentrations of T3 over a 10-hour period. There do not appear to be further descriptions of sustained release T3 therapy in the scientific literature since this report.
Compounded Thyroid Hormones
It is possible to obtain various thyroid hormone preparations from compounding pharmacies. Such pharmacies are described by the FDA as being engaged in “combining, mixing, or altering of ingredients to create a customized medication for an individual patient.” They thus mix drugs for various specific needs, including provision of the exact dose required by the patient. For example, a compounding pharmacy may mix T3 with methylcellulose and dispense tablets designed to have a sustained release profile. Compounding pharmacies are regulated by their respective states, but the issue of whether there is also federal jurisdiction over compounding pharmacies has been argued in the courts without a clear resolution (54).
Liquid Preparations
A LT4 preparation has recently become available in which the T4 is dissolved in glycerin and contained within a gelatin capsule. This preparation is reported by the manufacturer to have 100% absorption from the gastrointestinal tract, compared with 40% to 80% absorption reported by manufacturers of other LT4 preparations. The dissolution of this preparation appears to be relatively unaffected by pH (55). Therefore, its absorption, in theory, might be less affected by the altered pH of the gastric environment associated with food consumption, gastritis, and use of proton pump inhibitors than other LT4 products (see section Factors Affecting Absorption of Levothyroxine). Nevertheless, under the fasting conditions of FDA-approved bioequivalence methodology this product appears to satisfy criteria for bioequivalence to a brand name tablet (56).
Factors Affecting Levothyroxine Dose
Many factors can affect the LT4 dose required to normalize a particular patient’s TSH. Some of these factors are drugs that affect LT4 metabolism and transport (see Table 46.2). Other factors are characteristics that are particular to each patient (see Table 46.3). In addition, many drugs can also affect LT4 dose requirement by virtue of their effect on LT4 absorption (see Table 46.4). This latter group of drugs is discussed in the next section (Factors AffectingAbsorption of Levothyroxine).
Table 46.4 Drugs and Conditions That May Alter the Levothyroxine Dose Required By A Patient By Affecting Levothyroxine Absorption | |||||||||||||||||||||||||||||||||||||||
---|---|---|---|---|---|---|---|---|---|---|---|---|---|---|---|---|---|---|---|---|---|---|---|---|---|---|---|---|---|---|---|---|---|---|---|---|---|---|---|
|
Etiology
It is well established that the etiology of a patient’s hypothyroidism, which is closely linked to the amount of residual thyroid function, affects the dose of LT4 that will result in a normal TSH in a treated patient (57,58). Patients who are athyreotic as a consequence of thyroidectomy generally require a higher LT4 dose than patients with Hashimoto’s thyroiditis. Patients who have received radioiodine therapy for Graves’ hyperthyroidism may have a variable need for LT4, depending on whether they have remaining functional thyroid tissue.
Medications
The medications that may necessitate an adjustment in LT4 dose by virtue of altering T4 metabolism or changing the concentration of T4-binding globulin are shown in Table 46.2 (59). T4 and T3 are primarily metabolized by deiodination, but are also metabolized by conjugation with glucuronates and sulfates in the liver. The major enzymes involved in the conjugation are the mixed function oxidases and uridine diphosphate glucuronosyltransferases. Drugs that have either been shown to increase hepatic metabolism of T4 and T3 via their induction of these enzymes, or are presumed to have this effect, include phenobarbital (60), phenytoin (61), carbamazepine (62), rifampin (63), sertraline (64), and imatinib (65). Patients taking these medications may need to have their LT4 doses increased substantially above the usual replacement doses (59). Hypothyroid patients receiving treatment may also require an increased dose of LT4 to maintain a normal serum TSH when therapy with amiodarone is initiated (66). In this case the mechanism is not thought to be related to altered conjugation, but is postulated to be due either to decreased conversion of T4 to T3 in the pituitary and other tissues, or to decreased entry of T3 into pituitary thyrotrophs.
If drugs that increase the serum T4–binding globulin concentrations, and thus increase total T4 levels, are started in hypothyroid patients receiving LT4, their TSH levels may rise above the normal range, thus signaling the need for a higher dose of LT4 to compensate and restore the steady state. Examples of drugs that increase serum T4–binding globulin from insignificant to significant degrees (59) include estrogen (67), tamoxifen (68), raloxifene (69), clofibrate (70), opioids (71), mitotane (72), fluorouracil (73), and capecitabine (74). With respect to the classic case of estrogen treatment in post-menopausal women, such therapy was associated with an increase in mean TSH concentration from 0.9 mIU/L to approximately 3.2 mIU/L 12 weeks after initiation of estrogen therapy (67). Other drugs, such as androgens (75), anabolic steroids (76), and glucocorticoids (77) may have the opposite effect and decrease the serum T4–binding globulin concentrations, and thus typically necessitate a decrease in LT4 dose (59).
TSH Goal of Levothyroxine Therapy
Another factor affecting the LT4 dose that a patient requires is the TSH goal of their therapy. Patients who are being treated to achieve a normal TSH require a lower dose of LT4 than those with differentiated thyroid cancer being treated to achieve subnormal TSH values. The latter patient group requires higher doses (18,58,78). Normalization of TSH, in most studies, is achieved by doses in the range of 1.6 to 1.8 mcg LT4 per kilogram, whereas TSH suppression is achieved by doses of approximately 2.0 to 2.2 mcg/kg.
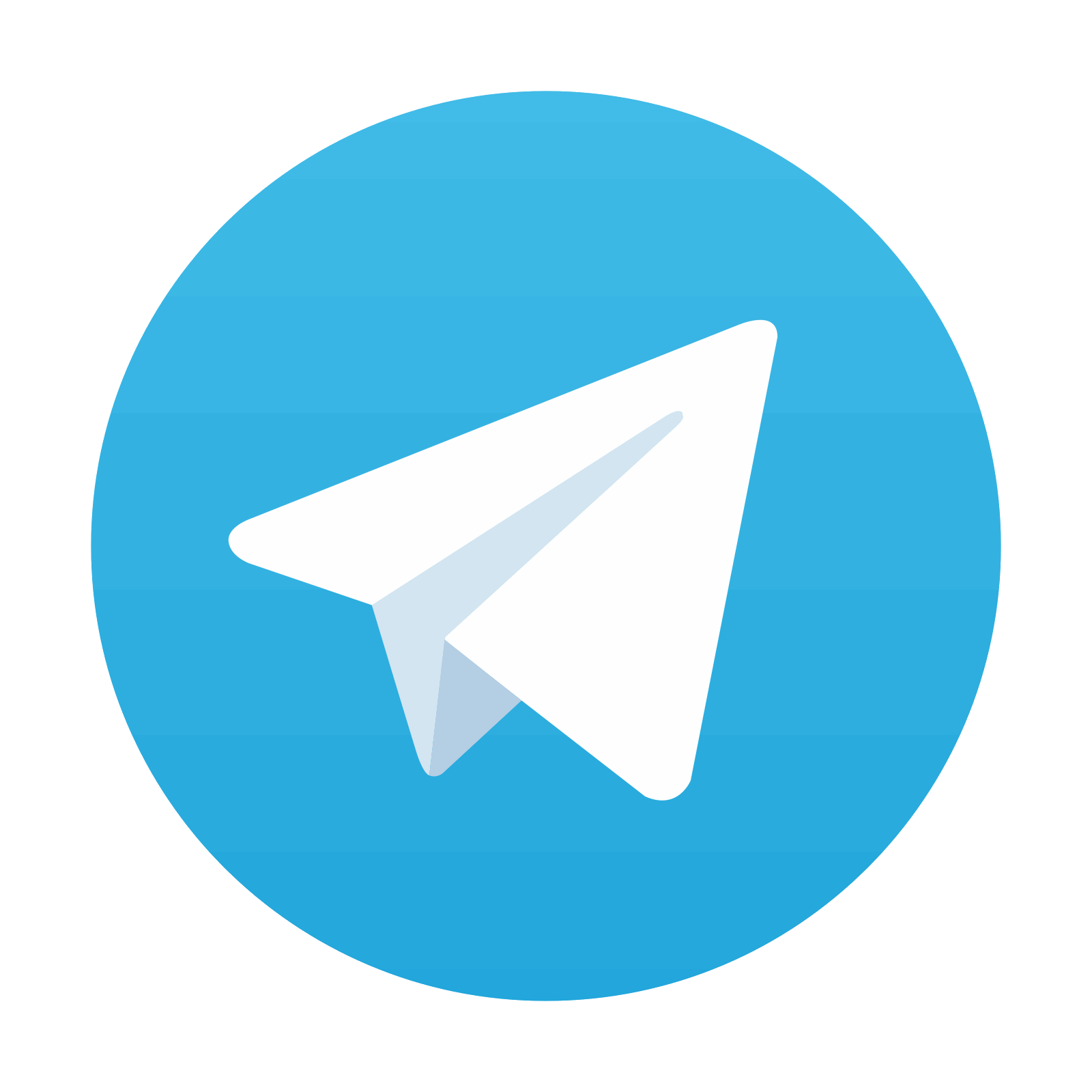
Stay updated, free articles. Join our Telegram channel

Full access? Get Clinical Tree
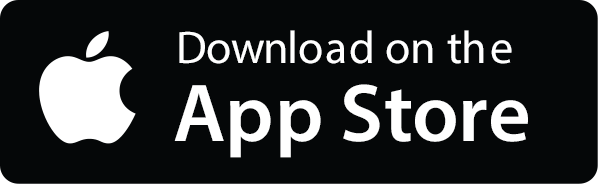
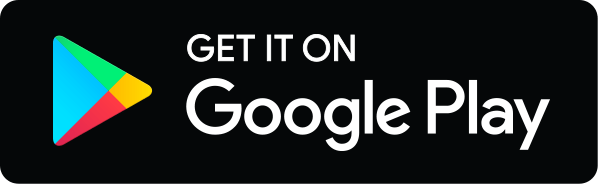