(1)
Mayo Clinic, Jacksonville, Florida, USA
3.1 Introduction
The lower prevalence of metabolic syndrome throughout the early part of the twentieth century meant that a greater proportion of patients suffered from type 1 diabetes and the total number of patients affected with type 2 diabetes was much lower than it is today. The introduction of insulin in 1922 extended survival for patients with type 1 diabetes mellitus (DM), but life expectancy remained poor because many died of disease-related complications. Since few patients lived long with DM, the incidence of diabetic retinopathy (DR) was low and early diagnosis and treatment were a low priority. In addition, only a small number of ophthalmologists served the population and their ability to accurately diagnose retinopathy was far more limited than it is today.
The development of DR was frequently accompanied by a steady decline in vision often with progression to legal blindness. Most physicians believed that tight glucose control decreased the incidence and progression of retinopathy but conclusive evidence did not emerge until completion of the Diabetes Complications and Control Trial and the United Kingdom Prospective Diabetes Study in the 1990s. Despite best attempts to control glucose, success was limited by inadequate dietary guidelines, lack of home glucose monitoring systems and hemoglobin A1c testing, and a limited choice of medications.
Several treatments for vision-threatening DR were tried, but efficacy was never demonstrated for some of them and significant side effects plagued others. This chapter begins by briefly discussing a couple of DR treatments that were tried and abandoned during the latter half of the twentieth century [46, 61, 131]. Most of the chapter focuses on the use of laser photocoagulation and vitrectomy for the treatment of diabetic macular edema (DME) and proliferative diabetic retinopathy (PDR). Laser and vitrectomy were introduced in the 1960s and 1970s, respectively, and still play an important role in the treatment of DR. Recent technological advances in the forms of micropulsed and navigated laser and small gauge vitrectomy have made these treatments easier to apply with potentially less damage to surrounding tissues. Current research with these modalities attempts to determine how they fit into today’s pharmacology-based treatment regimens.
3.2 Pituitary Ablation
Evidence supporting the notion that pituitary ablation alters the course of DM surfaced in the 1930s after pituitary glands had been removed from dogs that had undergone pancreatectomies [56] and cats that had undergone hypophysectomies and adrenalectomies [81]. Some observers believed that a “fundamental neural mechanism” was responsible for stabilization of retinopathy [156], while others suggested that pituitary ablation decreases or eliminates growth hormone, adrenocorticotrophin, and thyroid-stimulating hormone, which directly or indirectly through their downstream effects worsen DR [51]. Additionally, vision-threatening DR was rarely observed in growth hormone-deficient diabetic dwarfs. Support for pituitary ablation as a treatment for DR originally came from a case report (1953) of a woman whose DR regressed after she developed postpartum pituitary necrosis.
Few studies have compared the results of pituitary ablation for DR with control patients. In one report, 4 of 19 patients that underwent radioactive yttrium ablation of the pituitary experienced improved vision after 1 year, compared to 0 of 22 controls. Neovascularization of the retina decreased in 30% of ablated patients but in none of the controls [60]. In another report, 9 of 33 patients that underwent surgical resection of the pituitary stalk experienced decreased vision, compared to 19 of 33 controls. Retinopathy improved in 16 ablated patients but in only 9 controls [125]. In a review of 385 ablated patients, retinopathy was stabilized or improved in one-half [9]. Data from one series of patients suggested that improvement in retinopathy does not start until 12 months after ablation, so the authors recommended that ablation be performed early in the proliferative phase of retinopathy [5]. Pooled results from a large symposium reported that 75% of 708 patients had stabilized vision during the first 6 months after ablation [137]. Long-term visual results after ablation have not been well established, but up to one-third of patients with 5-year follow-ups experienced progression of retinopathy after an initial response to surgery [33].
Pituitary ablation was performed via surgical removal or resection, irradiation with yttrium or proton beam, or cryohypophysectomy (Fig. 3.1) [77, 122]. Surgeons believed that a more rapid and complete hypophysectomy had a greater effect on retinopathy [61]. Complications of pituitary ablation included cranial nerve palsies, cerebrospinal fluid rhinorrhea, meningitis, and herpes simplex encephalitis [133]. Following ablation, patients required lifelong hormonal replacement with corticosteroids and possibly thyroid and sex hormones.
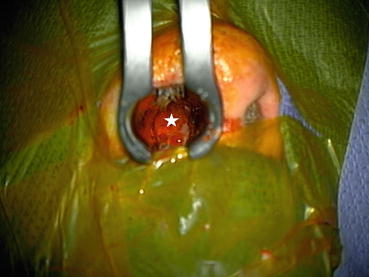
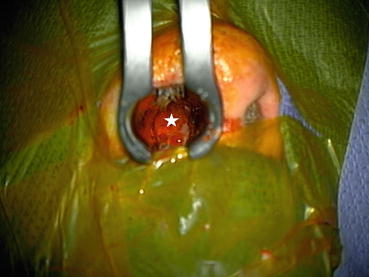
Fig. 3.1
This intraoperative photograph shows exposure of the pituitary gland (beneath the white star) via a trans-nasal approach. Trans-nasal hypophysectomies are no longer performed for diabetic retinopathy but are frequently employed for large pituitary tumors
Predicting which patients with DR would respond favorably to ablation was often difficult. Vascular lesions associated with both background and proliferative retinopathy were often observed to regress, but fluorescein angiography demonstrated that ischemia improved slowly [69]. Contraindications to pituitary ablation included retinal detachment, preretinal fibrosis, advanced age with cardiovascular disease, and the inability to tolerate long-term, complex hormonal replacement. The ideal patient was below the age of 40 years with good renal function, but 75% of patients failed to meet the surgical criteria.
Pituitary ablation was performed on hundreds of patients, but firm evidence supporting its efficacy was never obtained. Due to its lack of established efficacy and unacceptable complication rates, pituitary ablation had been discontinued at some medical centers by the mid-1960s. After the introduction and widespread use of laser photocoagulation, nearly all centers had discontinued pituitary ablation by the mid-1970s.
3.3 Interferon
Interferon is a pluripotent endogenous protein with antiviral, antiproliferative, and immunogenic activity that has been shown to limit endothelial cell motility and proliferation in vitro [38, 147]. Recombinant α-interferon has been used to treat pulmonary hemangiomatosis and human immunodeficiency-related Kaposi sarcoma [134, 153]. Since interferon only mildly interferes with glucose tolerance, it would be unlikely to interfere with metabolic control in patients with DM [151].
In a 4-month prospective study, 3 patients with proliferative diabetic retinopathy were treated with systemic interferon [131]. Subjects experienced mild fatigue, nausea, myalgias, leukopenia, thrombocytopenia, and transient ataxia. Fasting hyperglycemia and postprandial hyperglycemia increased from 2 to 6% despite a 17 to 68% increase in insulin dosage. During treatment with interferon, DR was stable without new hemorrhages, but all patients had new retinal bleeding after interferon was discontinued.
Interferon has also been evaluated for the treatment of neovascular age-related macular degeneration [48], but since these studies failed to show a therapeutic effect, no further testing of interferon in patients with DR was performed.
3.4 Laser Photocoagulation
Damage to the retina by excessive light has been recognized for centuries. Socrates warned against directly viewing solar eclipses and advised his pupils to indirectly view the reflected image of the eclipse off of a pool of water. Solar maculopathy was first described in the seventeenth century [50], and it has been suggested that the artist Degas suffered solar damage to the macula when standing watch on a sunny day. Hundreds of solar burns occurred in Europe during a solar eclipse in 1912.
These events represent the results of a photochemical effect on the retina and not the thermal or photocoagulative effect that occurs with photocoagulation. These observations, however, inspired researchers to develop light-based devices to photocoagulate the retina. Experimental photocoagulation of rabbit eyes with a concave mirror and convex lens was first performed in 1867 [18] and duplicated in 1882 [21]. A carbon arc lamp produced the same effect in1893 [154].
The first experimental photocoagulation of the human retina (1927) was performed in an eye scheduled for enucleation because of a choroidal melanoma. Sunlight focused on the retina for 10 min caused hyperemia and retinal edema [88]. The first instrument specifically designed for therapeutic photocoagulation of the retina (1949) focused the sun’s rays with a Galilean telescope and mirror [93]. This instrument was relatively unreliable because it depended on weather conditions. A next-generation device used a high-intensity carbon arc [94]. Though much more reliable, the carbon arc released soot and undesirable particles. The next light source was the xenon lamp, which produced a bright light with long-duration burns of moderate intensity over proliferating blood vessels [95, 96]. The xenon lamp was an effective photocoagulator for years, but it was eventually replaced by the ruby laser, the argon blue-green and green lasers, the krypton laser, the tunable dye laser, and most recently the infrared diode and frequency-doubled diode laser (Table 3.1). These lasers produce monochromatic light with small spot sizes and better control of power and pulse duration. They enable physicians to treat not only proliferative retinopathy with broad areas of photocoagulation but also macular edema with precise placement of small spots. The diode lasers are highly efficient as they derive power from standard electrical outlets without the need for specially installed, high voltage outlets.
Table 3.1
Commonly used laser systems are listed
Common types of laser used for retinal photocoagulation | |
---|---|
Long-duration laser systems | |
Argon | Argon gas in replaceable tube Wavelengths in blue (488 nm) and blue-green (514 nm) part of spectrum Use has diminished because of high-power requirements and need to periodically replace tube |
Krypton | Krypton gas in replaceable tube Wavelengths in red (627 nm) part of spectrum Use has diminished because of high-power requirements and need to periodically replace tube |
Tunable dye | Dye allows modification of wavelength including 577 nm, highest uptake of hemoglobin |
Frequency-doubled YAG (solid state) | Solid-state laser uses 120 volt power output Spots at wavelength of 532 nm Device has long lifespan |
NAVILAS | Computer-guided system delivers spots directly to spots identified on fluorescein angiography Some surgeons believe that NAVILAS delivery may be better than surgeon directed photocoagulation |
Micropulse laser systems | |
Visible and infrared | Subthreshold laser delivery. Repeated delivery may be performed. Some surgeons suggest that subfoveal delivery is safe Has not been subjected to randomized, multicenter, masked trials |
Each of these instruments produces a collimated beam of coherent light that is transmitted through the ocular media to the retina where it is absorbed by retinal chromophores – xanthophyll, hemoglobin, and melanin. Light absorption produces a photothermal effect that heats the surrounding tissues and induces inflammation and edema that eventually causes localized tissue necrosis. High-intensity laser pulses produce burns that affect all levels of the retina, retinal pigment epithelium (RPE), and choroid, whereas low-energy pulses affect primarily the photoreceptors and RPE but spare the inner retina [17, 43]. Longer wavelengths penetrate deeper into the choroid because they are absorbed poorly by xanthophyll chromophores. Histopathologic studies confirm that the resultant scars vary according to wavelength, but the clinical importance of this has not been proven.
While physicians were evaluating laser photocoagulation, retinal photography was also being developed. High-quality, stereoscopic color fundus photographs enabled physicians to identify microaneurysms and areas of diffuse macular thickening. Stereoscopic photos were critical for enrolling patients in prospective studies for the treatment of DME. Fluorescein angiography showed that DME develops from both leakage of microaneurysms and diffuse leakage from incompetent capillary beds.
3.4.1 Techniques for Performing Laser Photocoagulation
When choosing to perform laser photocoagulation of the retina, ophthalmologists can choose among different machines and techniques. Several manufacturers produce user-friendly, reliable lasers that produce excellent retinal burns. Most current laser systems have abandoned the use of Nobel gases (argon and krypton) and tunable dyes and have migrated to diode technology. Diodes are reliable, durable, and portable and require minimal servicing, and unlike the old gas-generated lasers, diodes work with 120 volt electrical outlets, thereby making installation easier and use in the clinic and operating rooms safer. They produce a 532 nm beam that is moderately absorbed by oxyhemoglobin and minimally absorbed by retinal xanthophylls.
The most common laser delivery methods include the slit-lamp biomicroscope, indirect laser ophthalmoscope, and intraocular probe. In the outpatient or clinic setting, slit-lamp delivery systems are most commonly used, particularly for the treatment of DME (Fig. 3.2). The retina is visualized through a high-diopter condensing lens or a contact lens which may be flat (for the macula), may invert images (such as the panfundus or Mainster lenses that are generally used for panretinal or scatter photocoagulation), or may be mirrored (for treatment of the peripheral retina). Panfundus and Mainster lenses magnify the spot size by factors of 1.75 and 2.2, respectively. Topical anesthesia is usually sufficient for most treatments though retrobulbar anesthesia improves pain control and limits ocular motility.
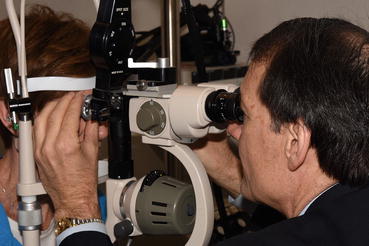
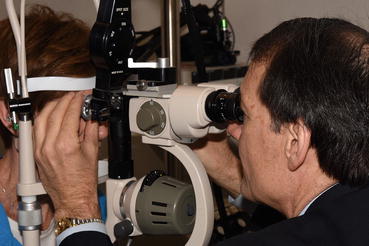
Fig. 3.2
Laser treatment of diabetic macular edema and proliferative diabetic retinopathy is most commonly performed with the slit-lamp delivery system. The surgeon directly visualizes the retina through a contact lens and focuses the laser beam on microaneurysms, areas of capillary leakage, and areas of peripheral retina
Macular edema is usually treated with the slit-lamp delivery system through a flat lens. Microaneurysms are focally treated with 50–100 μm spots and sufficient power to blanch them. A complete or modified grid pattern of spots is used to treat broader areas of capillary leakage (Fig. 3.3). A typical laser treatment includes 50–100 μm spot sizes, power to produce light burns with only mild blanching of the retina and pigment epithelium, and separation of burns by 2 spot diameters. Spots are not placed within 500 μm of the fovea during the initial treatment, but if edema persists and visual acuity remains decreased, subsequent spots can be placed to within 300 μm of the fovea but outside of the foveal avascular zone. If the foveal avascular zone is larger than normal because of capillary non-perfusion, spots should be kept further from the fovea to avoid areas of perifoveal non-perfusion.
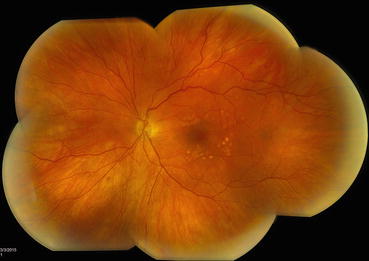
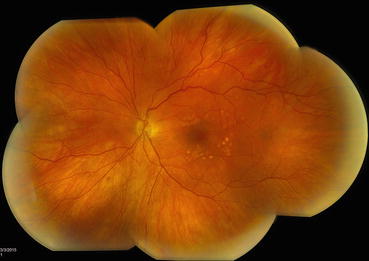
Fig. 3.3
This composite photograph shows scarring from a previous modified grid laser pattern in the macula. Note that the spots are not confluent and they do not completely encircle the macula
Treatment recommendations have changed since the older ETDRS guidelines when spots were larger, closer together, and more powerful (Fig. 3.4). The new guidelines produce a subtler treatment pattern that is believed to cause fewer permanent scotomas. Since the spacing between spots is increased, there remains sufficient space for repeat laser treatments, if needed.
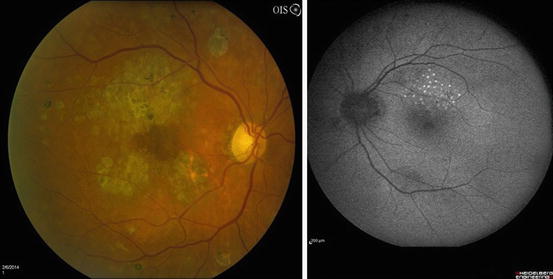
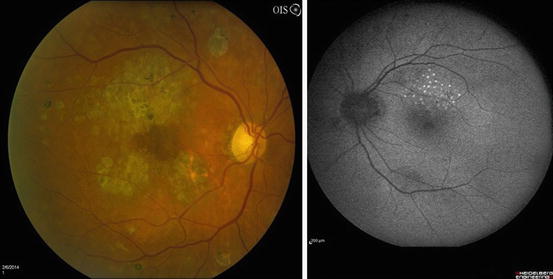
Fig. 3.4
The figure on the left shows confluent laser spots resulting from large, intense laser spots that may have been placed too close together. These spots had been placed according to the original ETDRS guidelines. The figure on the right shows a treatment pattern that was done in accordance with the new modified treatment guidelines. The spots are smaller, less intense, and spaced farther apart. This decreases the likelihood of spot enlargement or “laser creep”
Treatment of non-center-involving edema, even in the anti-VEGF drug era, usually includes focal treatment of microaneurysms and a modified grid pattern to areas of edema. Some studies suggest that focal treatment of microaneurysms may not be required as treatment of the RPE may produce the desired effect [115]. Extrafoveal edema often resorbs after laser and the effect may be durable, thereby preventing the subsequent need for a series of anti-VEGF injections. If necessary, laser treatments can be repeated at 3–4-month interval. When a retreatment is planned, fluorescein angiography may help identify areas of persistent leakage.
Complications of focal/grid laser are unusual but can be serious. Laser photocoagulation permanently damages photoreceptors and retinal pigment epithelium, and large, heavily pigmented laser scars may cause paracentral scotomas. Densely applied treatment patterns decrease color vision and contrast sensitivity. Laser spots can enlarge and encroach on the fovea – referred to as “laser creep.” In one series of patients with DME, enlarging laser scars reached the fovea in 11 of 203 eyes [127]. Laser scars in the posterior pole expand more than those in the periphery possibly because the greater density of photoreceptors facilitates heat transfer [87]. Surgeons must be careful not to use too much energy when using small spot sizes because of the risk of an RPE rupture. Rupture can be followed by choroidal neovascularization with accompanying subretinal hemorrhage, exudation, and disciform scarring [80, 82].
Several pathophysiologic mechanisms have been proposed to explain why macular laser improves DME. Laser is believed to increase local oxygen tension [135], decrease autoregulatory vasoconstriction [45], decrease the entire area of abnormal leakage [155], restore the outer blood-retinal barrier [42, 89], decrease the production of vasoactive cytokines (primarily VEGF), and increase phagocytosis by the RPE and retinal glial cells. RPE cells at the margins of burns may modulate the production of cytokines through their interactions with photoreceptors [28, 100].
Proliferative diabetic retinopathy has been treated with scatter or panretinal photocoagulation (PRP) for the past 40 years. Moderate-intensity spots of 350–500 μm in diameter and 0.075–0.2 s. in duration are delivered through an inverting, panfundus contact lens or 3-mirror contact lens to the mid-peripheral retina. Spots are spaced 1 diameter apart and are applied from just outside the major vascular arcades to the far peripheral retina (Fig. 3.5). Complete treatment usually consists of 1200–3000 spots, but more may be delivered either primarily or for resistant cases. In the Diabetic Retinopathy Study (DRS), photocoagulation was applied directly to patches of new blood vessels, but since this does not adequately address the underlying cause of neovascularization and fails to promote regression, this strategy has been abandoned. Panretinal photocoagulation can also be performed with an indirect ophthalmoscopic delivery system, which enables physicians to more completely treat the far peripheral retina (Fig. 3.6). Treatments are often divided into 2–3 sessions to improve patient comfort. Many physicians choose to photocoagulate the inferior retina first so that if the post-laser course is complicated by a vitreous hemorrhage, the view of the superior retina is less likely to be obstructed and the course of treatment can be completed. Smaller spot sizes and longer wavelengths (such as 810 nm) penetrate vitreous hemorrhages better.
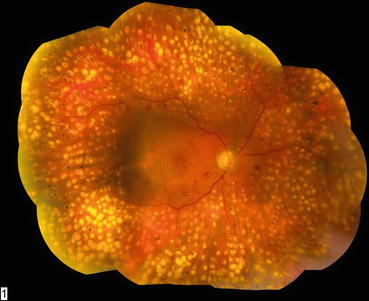
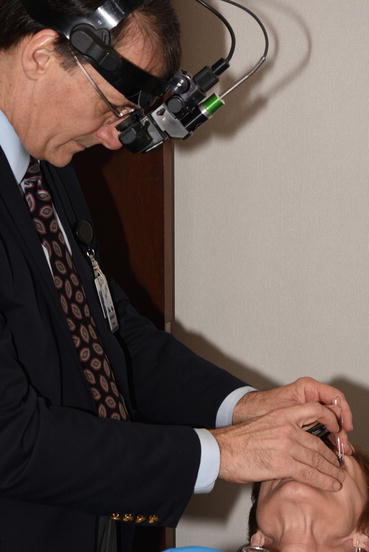
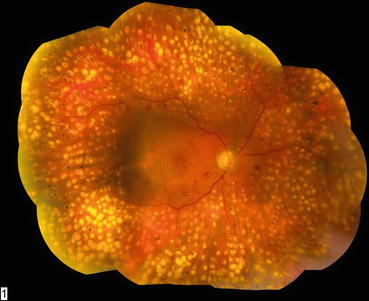
Fig. 3.5
This composite fundus photograph shows mature laser scars following a completed panretinal photocoagulation treatment. The treatment has been extended posteriorly to the vascular arcades to achieve maximum effect
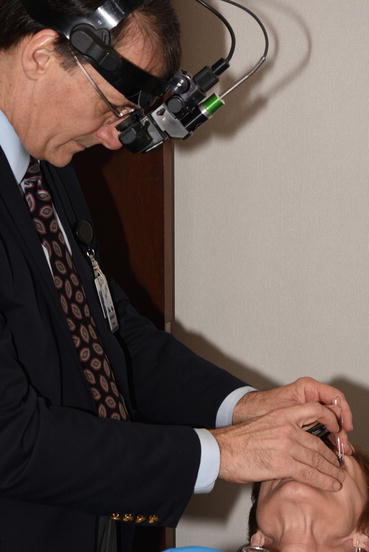
Fig. 3.6
Panretinal photocoagulation can be delivered through the indirect ophthalmoscope with the patient in the supine position. Since ultra-widefield angiography has shown that broad areas of peripheral retinal non-perfusion may contribute to the development of neovascularization, many surgeons advocate the routine use of indirect ophthalmoscopic laser delivery in all cases of proliferative diabetic retinopathy
Preretinal neovascularization usually regresses within 3 weeks of adequate PRP (Fig. 3.7) [24], and patients who have a favorable early response usually have a better visual outcome than those who do not [149]. Persistent or progressive neovascularization despite the performance of a standard course of PRP may respond to additional laser. Indirect laser-assisted treatment with up to 8000 spots [150] has been described. Ultra-widefield fluorescein angiography can visualize persistent neovascularization and identify untreated areas of peripheral retinal ischemia. These images can be used to guide the placement of supplemental photocoagulation.
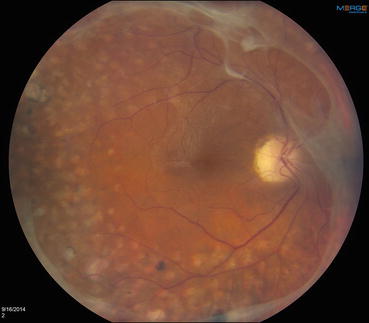
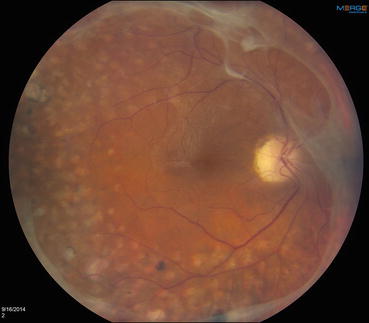
Fig. 3.7
This photograph of the posterior pole shows panretinal photocoagulation scars that have been extended to the vascular arcades. A near-complete ring of preretinal fibrosis extends around the macula, and an epiretinal membrane is causing mild macular wrinkling. This represents successful post-laser regression of what were previously active neovascular fronds
Panretinal photocoagulation that spares the posterior retina and preserves the paracentral visual field is sufficient to cause neovascular regression in many cases [7]. Ultra-widefield fluorescein angiography has subsequently shown that most retinal non-perfusion is found in the mid- and far periphery, thereby validating the logic of this approach.
Exudative choroidal detachments may complicate intensive, widespread PRP, but this rarely occurs after split-session treatments [57]. High-intensity burns can rupture Bruch’s membrane and lead to retinal and vitreous hemorrhages and the ingrowth of choroidal neovascular membranes [11]. Peripheral visual field loss and night blindness can follow high-intensity PRP. Worsening of preexisting vitreoretinal traction can occur after intense photocoagulation [92], but treatment with divided sessions usually avoids this complication.
Broad areas of mid-peripheral and peripheral retina must usually be ablated to successfully involute neovascularization. Physicians have long appreciated that the severity of neovascularization correlates with the amount of retinal ischemia [98], but the biochemical processes responsible for angiogenesis were not understood. The recent discovery of vascular endothelial growth factor (VEGF) [16, 32], the understanding of its pivotal role in angiogenesis [10, 31, 47, 138], and its detection in the vitreous in eyes with PDR suggest that it plays an important role in eyes with PDR.
Several lines of evidence associate VEGF upregulation with retinal neovascularization. Tissue hypoxia is the most important upregulator of VEGF synthesis [26], and advanced DR is characterized by broad areas of capillary closure with retinal ischemia. The cell’s oxygen sensor, hypoxia-inducible factor (HIF)-1α, is stabilized when oxygen tension is low, enabling it to upregulate the synthesis of VEGF (Fig. 3.8) [44, 148]. Administration of exogenous VEGF into monkey eyes induces retinal neovascularization and other findings typical of DR [144]. Panretinal photocoagulation is thought to improve overall retinal oxygen tension by either destroying large areas of ischemic retina or increasing oxygen diffusion from the choroid into the remaining retina. Improved retinal oxygenation downregulates VEGF and involutes immature neovascular vessels.
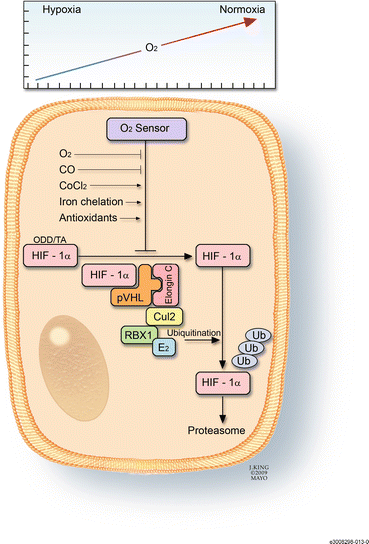
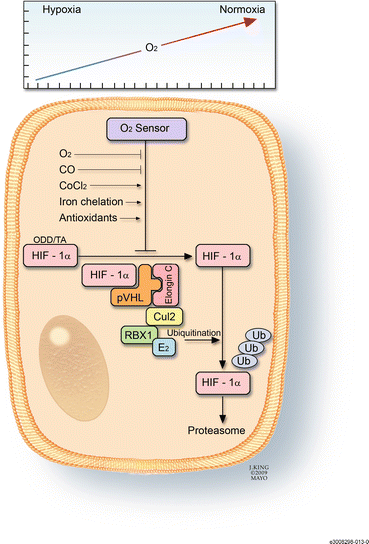
Fig. 3.8
This drawing shows the mechanism by which hypoxia-inducible factor (HIF)-1α acts as the cell’s oxygen sensor. In the presence of sufficient oxygen, HIF-1α binds to the von Hippel-Lindau complex, undergoes ubiquination, and is eventually degraded in the proteosomes. This process can be affected by factors such as carbon monoxide, iron chelators, and antioxidants
3.5 Treatment of Background Diabetic Retinopathy
The Early Treatment of Diabetic Retinopathy Study (ETDRS) produced level I evidence supporting the use of laser photocoagulation for patients with clinically significant macular edema (CSME) due to diabetic retinopathy (Table 3.2). The ETDRS was designed to test the theory that laser photocoagulation is superior to observation for patients with CSME [29]. Diabetic patients were eligible for enrollment if they had biomicroscopic evidence of CSME, which was defined as follows:
- 1.
Retinal thickening within 500 μm of the fovea
- 2.
Hard exudates within 500 μm of the fovea that were accompanied by adjacent thickening
- 3.
Retinal thickening of 1 disc diameter in area part of which was within 1 disc diameter of the fovea
Table 3.2
The definitions of clinically significant macular edema (CSME) and diabetic macular edema (DME) are listed
Clinically significant macular edema is diagnosed if ANY of the following is met: |
1. Thickening at or within 500 μm of the fovea |
2. Hard exudates within 500 μm of the fovea with adjacent retinal thickening |
3. An area of macular thickening at least 1 disc area in size, any part of which is within 1 disc diameter of the fovea |
**To make the diagnosis of CSME, macular thickening is diagnosed by slit-lamp biomicroscopy |
Diabetic macular edema is diagnosed if the following is met: |
1. Thickening within 2 disc diameters of the fovea |
For clinical purposes, DME is classified as: |
1. Center-involving or non-center-involving |
2. Diffuse or focal |
Eligible eyes had baseline visual acuities that ranged from 20/20 to 20/200. Fluorescein angiography (FA) was performed at baseline, but angiographic findings were not used to define CSME (Fig. 3.9). Angiography served two main purposes in patients with diabetic macular edema. First, it identified microaneurysms and areas of diffuse capillary leakage, information that helped physicians accurately place laser spots. Second, the FA identified areas of capillary non-perfusion, information that helped physicians set appropriate visual expectations with the patients and helped them avoid treating areas of non-perfusion.
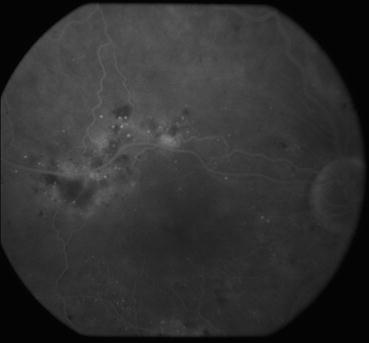
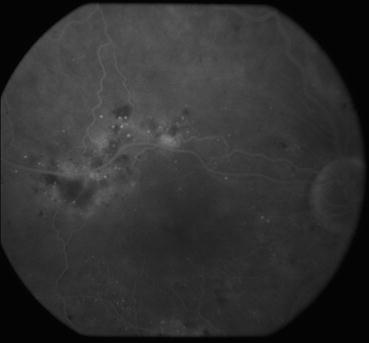
Fig. 3.9
This venous phase frame from a fluorescein angiogram shows areas of dye leakage in the superior macula from microaneurysms and capillary beds. Macular edema is usually found in areas that display these findings
Enrolled eyes were randomized to observation or fluorescein angiography-guided laser photocoagulation. Treatment was applied focally to microaneurysms and in a grid pattern to areas of diffuse capillary leakage. Microaneurysms were treated directly with spot sizes of 50–100 μm and durations of 0.05–0.1 s until either darkening or whitening of the microaneurysms was observed; areas of diffuse capillary leakage received a grid pattern of laser with moderate-intensity burns, spot sizes of 100 μm separated by 100 μm, and duration of 0.05–0.5 s. Spots were not applied within 500 μm of the foveal center, and treatment could be extended out past 3000 μm from the fovea. Eyes were eligible for retreatment on a quarterly basis to areas of persistent or new leakage that were untreated or judged to be incompletely treated. Argon laser was used during the ETDRS, but other wavelengths are likely to be as effective [116]
Overall in the ETDRS, 30% of laser-treated patients experienced some improvement in BCVA. Patients receiving laser had a 50% decrease in their risk of moderate vision loss (15 letters) over the course of 3 years. Unfortunately, only 3% of laser-treated patients improved by +15 letters (three lines) at 3 years and 40% of laser-treated eyes had persistent CSME at 12 months [30]. Nonetheless, the trial results suggested that all eyes with CSME should be treated with laser, including those with 20/20 visual acuity.
Persistent DME following laser photocoagulation is not uncommon as the closure rate of microaneurysms with focal laser is only 70% [78], and the need for repeat treatment to areas of diffuse capillary leakage is common. Unless the underlying disease process is halted, new areas of macular thickening frequently develop.
3.6 Treatment of Proliferative Diabetic Retinopathy (PDR)
Randomized clinical trials have validated the efficacy of panretinal photocoagulation for proliferative diabetic retinopathy, and many of these were summarized in a 2007 systematic review [102]. An early British trial used the xenon arc photocoagulator [12] to treat PDR, and this was soon followed by the National Eye Institute-sponsored Diabetic Retinopathy Study (DRS; ClinicalTrials.gov number, NCT00000160). The DRS was one of the first multicenter, randomized studies in medicine and served as a model for subsequent collaborative trials [139]. The DRS enrolled 1758 patients with visual acuities of 20/100 or better in each eye and with either proliferative diabetic retinopathy in at least one eye or severe nonproliferative diabetic retinopathy in both eyes. Each patient was randomly assigned to undergo panretinal photocoagulation with either the xenon arc photocoagulator or argon laser in one eye, with the other eye serving as the untreated control. Treatment consisted of 800–1600 burns of 0.1 s duration and new blood vessels were treated directly. Panretinal photocoagulation reduced the risk of severe vision loss (defined as visual acuity of 20/800 or worse at two consecutive 4-month visits) from 14.0 to 6.2% over a 2-year period [139] and from 33.0 to 13.9% over a 5-year period [142].
There were no significant differences between the xenon arc and argon groups regarding the primary outcome, but eyes treated with argon maintained better visual acuities and fewer visual field and night vision defects. Panretinal photocoagulation reduced the overall incidence of severe vision loss by at least 50% [140]. Eyes at high risk of vision loss included those with moderate neovascularization of the disc (covering at least ¼ of the disc area) and those with any neovascularization of the disc (NVD) or elsewhere (NVE) that was accompanied by preretinal or vitreous hemorrhage [141].
The ETDRS (NCT00000151) enrolled 3711 patients with mild-to-severe nonproliferative or early proliferative diabetic retinopathy in both eyes. Each patient was assigned to undergo panretinal photocoagulation in one eye, while treatment in the fellow eye was deferred until the development of high-risk PDR. Rates of severe vision loss at 5 years were 2.6% following early treatment and 3.7% following deferred treatment [30]. The ETDRS showed that performing PRP in eyes with advanced pre-proliferative diabetic retinopathy generally does not change the visual outcome. The Diabetic Retinopathy Clinical Research Network published guidelines for the treatment of proliferative diabetic retinopathy [23]. Treatment should be initiated promptly when an eye develops high-risk PDR as delaying treatment increases the short-term risk of vision loss because vitreous hemorrhage may develop.
Treatment of coexistent diabetic macular edema and high-risk PDR is particularly challenging. Following PRP, 25–43% of eyes may develop DME or experience an increase in macular leakage with resulting loss of visual acuity [54, 90, 91]. The mechanism responsible for the development of DME after PRP is not well understood, but the accumulation of leukocytes in the posterior pole and the increased release of permeability promoting chemokines and cytokines (including VEGF) due to laser damage may play a role [40, 113, 146]. The ETDRS previously recommended macular laser photocoagulation for DME either before or at the time of PRP [1, 30]. Macular laser photocoagulation performed at these times decreased the risk of moderate visual acuity loss by 50%, but patients experienced minimal visual improvement and only 3% experienced three-line improvements in VA at 3 years. Unfortunately, 12% of eyes lost three lines of VA at 3 years, and 40% of eyes with baseline macular edema had persistent edema at 12 months.
These treatment guidelines were formulated before the introduction of retinal pharmacotherapy, and recent studies have demonstrated that the intravitreal injection of triamcinolone acetonide or anti-VEGF agents prior to PRP minimizes the exacerbation of DME and in many cases with pre-existing edema actually improves VA. Furthermore, pharmacotherapy is synergistic with panretinal photocoagulation in promoting the regression of retinal neovascularization [4, 14, 145, 163]. In a randomized, comparative study, intravitreal triamcinolone was superior to bevacizumab at resolving macular edema and preventing PRP-induced VA loss in eyes with preexisting macular edema, while both drugs were effective at preventing the development of edema in eyes with dry maculas [15]. Recent findings suggest that ranibizumab monotherapy effectively treats PDR through 2 years [157], so anti-VEGF injections may be increasingly used to treat both DME and PDR over extended periods of time, thereby delaying or even avoiding the need for macular laser or PRP.
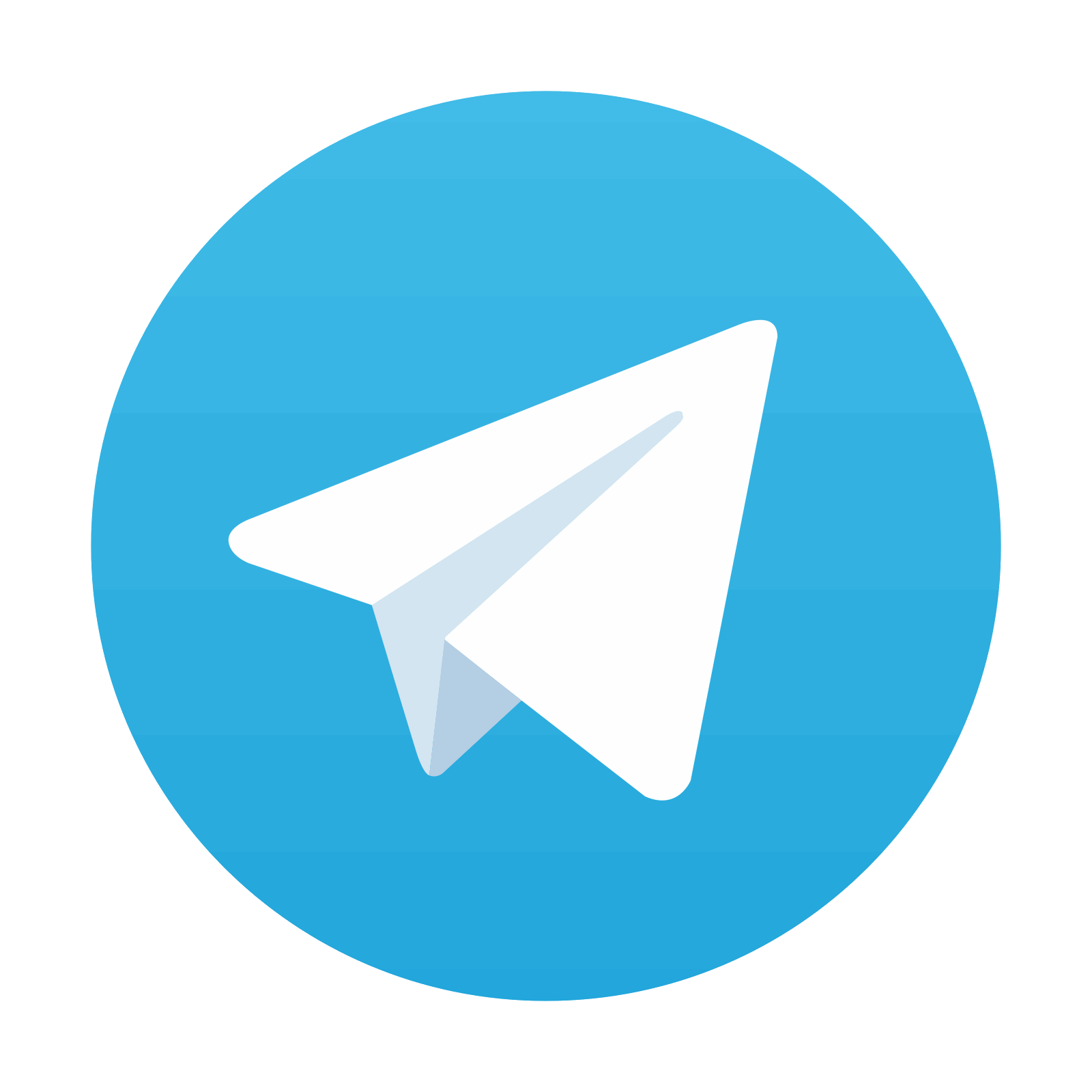
Stay updated, free articles. Join our Telegram channel

Full access? Get Clinical Tree
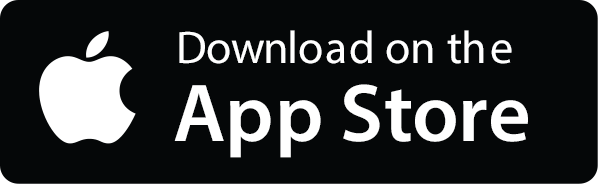
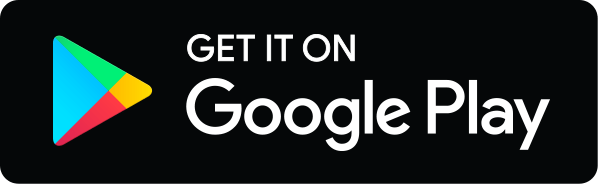