Alloimmunity in pregnancy is the basis for two of the major complications of pregnancy in transfusion medicine: hemolytic disease of the fetus and newborn (HDFN), and fetal and neonatal alloimmune thrombocytopenia (FNAIT). Use of Rh(D) immune globulin has dramatically reduced the incidence of HDFN in Rh(D)-mismatched pregnancies. Treatment of HDFN may involve intrauterine transfusion, with fetal and neonatal survival rates of 70% to 90%. Treatments for FNAIT include immune globulin, steroids, or in severe cases, intrauterine platelet transfusions. Transfusion medicine is central to the management of pregnancy-associated complications such as postpartum hemorrhage, parvovirus B19 infection, hemoglobinopathies, and aplastic anemia.
In 1901, Landsteiner proposed the existence of defined blood groups based on the observation that cross-mixing of red blood cells and sera from different healthy individuals sometimes led to red blood cell agglutination. Landsteiner’s finding of reproducible isoagglutination patterns among healthy individuals led to the elucidation of the ABO blood group, eventually allowing the development of safe and routine blood transfusions. Since the discovery of the ABO system, 30 other blood groups encompassing 308 blood group antigens have been identified.
A blood group system is defined as the set of red cell antigens produced by the alleles of a single genetic locus. The blood group antigens represent a heterogeneous collection of red cell surface molecules with diverse functions in membrane structure and physiology. Blood group antigens exert influence over a variety of human diseases, from thrombophilia to malarial infection. Clinically, the most significant property of blood group antigens is their immunogenicity, with alloimmunization occurring in antigen-negative recipients of allogeneic blood product transfusions and in pregnant women following exposure to fetal antigens. The major blood groups are those whose antigens have the most potent immunogenicity; in humans, these are ABO and Rh.
Pregnancy poses a special immunologic challenge in that maternal immunity offers fetal protection, yet the fetus itself represents an alloantigen from the perspective of the maternal adaptive immune system. Maternal IgG antibodies, but not other isotypes, cross the placental barrier, and confer immunity to the fetus throughout fetal life and for the first few months postpartum. To mitigate the potential immunologic risks associated with maternal IgG antibodies bearing specificity against fetal antigens, the fetus uses several protective mechanisms, including expression of nonclassic human leukocyte antigen (HLA) molecules with limited polymorphism, creation of a “placental sink” to trap detrimental maternal antibodies via fetal trophoblastic Fcg receptors, modulation of placental complement activity, and suppression cytotoxic Th1-type responses in favor of humoral Th2-type responses within the placental milieu. The maternal/fetal immunologic relationship may endure beyond birth, as microchimerisms containing fetal DNA remain detectable in maternal blood for years.
Alloimmunity in pregnancy is the basis for two of the major complications of pregnancy in transfusion medicine: hemolytic disease of the fetus and newborn (HDFN) and fetal and neonatal alloimmune thrombocytopenia (FNAIT). Other roles for transfusion medicine in pregnancy include management of obstetric hemorrhage, parvovirus B19 infection, hemoglobinopathies, and aplastic anemia.
Hemolytic disease of the fetus and newborn
HDFN (formerly erythroblastosis fetalis) encompasses a spectrum of fetal and neonatal disease, characterized by jaundice, hepatosplenomegaly, hemolytic anemia, and hydrops (fetal anasarca). In the early part of the twentieth century, the biologic basis for HDFN was postulated to be maternal alloantibodies against unidentified fetal antigens. In 1939 Levine and Stetson reported the landmark case of a 25-year-old G 2 P 1 female who developed preeclampsia, delivered a macerated fetus at 33 weeks’ gestation, and sustained a profound hemolytic anemia following transfusion of her husband’s ABO-matched blood for postpartum hemorrhage. Similar isoagglutination reactions involving ABO-matched donor/recipient pairings had been reported earlier in recipients of multiple blood transfusions. Work by Landsteiner, Wiener, and colleagues ultimately established Rh incompatibility as the biologic basis both for ABO-independent isoagglutination and for HDFN via inappropriate exposure of paternally derived fetal Rh antigens to an Rh-negative mother as a result of complications during pregnancy or delivery.
Rh Proteins and Rh(D) Phenotypes
The Rh blood group is the most polymorphic of the human blood groups, with 50 distinct blood group antigens. The 5 major Rh antigens—C, c, E, e, and D—represent the products of two genes on chromosome 1p, RHCE and RHD , which encode the 30 kDa RhCE and RhD transmembrane proteins, respectively. RhCE and RhD are expressed on the red cell surface as part of a 170 kDa complex that includes the related RhAG (Rh-associated glycoprotein) protein encoded by RHAG on chromosome 6p. The Rh and RhAG proteins function as ammonia transporters.
While maternal/fetal incompatibility in many different blood groups can lead to HDFN, antibodies to Rh(D) remain the most significant cause due to the unique immunogenicity of the Rh(D) protein. Several Rh(D) phenotypes have been characterized. D+ corresponds to the wild-type RHD gene sequence, although silent polymorphisms have been reported. D-negative corresponds to mutations that eliminate RhD production, including regulatory defects in RHD expression, deletions in RHD , or deletions of the entire RHCE-RHD locus (“Rh null disease”). Weak D arises from mutations encoding amino acid substitutions in transmembrane or intracytoplasmic portions of the RhD protein, generating reduced levels of RhD that retain the immunogenicity of the most critical extracellular epitopes. Partial D arises from mutations that encode amino acid substitutions on or near the red cell extracellular surface, where most of the immunogenic epitopes lie, resulting in impaired immunogenicity. Approximately 85% of the United States population is D+, including 83% of whites, 93% of blacks, 93% of Hispanics, 96% of Native Americans, and 98% of Asians. Less than 1% of the population harbors a weak or partial D phenotype.
Pathogenesis, Clinical Features, and Epidemiology of HDFN
Paternally derived fetal Rh(D) is synthesized in fetal red blood cells as early as 5 to 12 weeks’ gestation. Due to the immunologic privilege afforded by the placental barrier, maternal Rh(D) alloimmunization requires direct exposure of Rh(D) to the maternal circulation via one of the following mechanisms.
Fetomaternal hemorrhage (FMH, transplacental hemorrhage, or fetomaternal transplacental hemorrhage)
FMH is defined as entry of fetal blood cells into the maternal circulation before or during delivery. Minute hemorrhagic volumes of less than 0.5 mL fetal red cells are capable of inducing maternal alloimmunization. The risk of FMH increases over the course of pregnancy and is greatest at delivery, with 70% to 100% of postpartum mothers having detectable circulating fetal cells after birth, albeit usually at concentrations insufficient to induce clinically significant alloantibody production.
Transfusion of D+ blood into a D-negative mother
The risk of alloantibody formation following transfusion of Rh(D)-incompatible blood is directly proportional to the inoculating donor red cell dose. In transfusion studies of D-negative men injected with different volumes of D+ red cells, more than 40% developed anti-D antibodies following inoculation of 5 to 20 mL of cells, compared with more than 90% with inoculation volumes of 200 to 500 mL.
HDFN primarily affects second or later pregnancies. Initial exposure of fetal Rh(D) to a D-negative mother generates a primary, IgM-mediated antibody response, whereas reexposure produces a secondary (anamnestic), IgG-predominant response. Following Rh(D) alloimmunization and reexposure, maternal anti-D IgG antibodies enter the fetal circulation and bind to D+ fetal red cells. As the opsonized cells migrate through the fetal circulation, they are recognized by Fcg receptors on fetal hepatic and splenic macrophages, inducing erythrophagocytosis. Extravascular hemolysis ensues, leading to jaundice, kernicterus, hepatosplenomegaly (from extramedullary hematopoiesis), reticulocytosis, and hydrops (from hypoalbuminemia due to liver dysfunction and shifts in cardiac output due to impaired tissue oxygenation).
Prior to the introduction of Rh(D) immune globulin, clinically significant maternal anti-D alloantibodies developed in 13% to 16% of cases of maternal/fetal Rh(D) phenotypic incompatibility. Factors affecting the likelihood of maternal anti-D IgG antibody production and severity of HDFN include the following.
Maternal/fetal ABO incompatibility
In studies before the era of Rh(D) immune globulin, 2% of D-negative mothers with D+ fetuses and concurrent maternal/fetal ABO incompatibility became immunized to Rh(D), compared with 16% for ABO-compatible pairings. The protective effect of ABO-incompatibility in this setting is attributed to antigen clearance by maternal anti-A or anti-B IgM antibodies, leading to complement-mediated removal of opsonized A+ or B+ fetal red cells from the maternal circulation.
IgG subclass
HDFN can be caused by anti-D alloantibodies of either IgG1 or IgG3 subclass. IgG1 antibodies penetrate the placental barrier earlier than IgG3 antibodies, resulting in higher fetal concentrations and more severe fetal disease.
Weak or partial D phenotypes
Due to the preservation of immunogenic Rh(D) epitopes in mutations that give rise to weak D phenotypes, weak D mothers are usually tolerant to Rh(D), whereas partial D mothers are typically unsensitized. In practice, the risk of HDFN in D+ fetuses conceived in weak or partial D mothers is variable, although cases of HDFN have been reported.
Maternal anti-D IgG alloantibody titer
In 62% of cases, maternal anti-D alloantibody titer predicts onset and severity of HDFN.
In the era before Rh(D) immune globulin, HDFN was fatal in up to one-third of neonates. The advent of Rh(D) immune globulin as effective prophylaxis dramatically reduced the incidence of HDFN, from 45.1 cases per 10,000 live births in the United States in 1970 to 14.3 in 1983. With the addition of universal antenatal screening and use of intrauterine transfusion in cases of severe HDFN, D+ neonates born to D-negative, sensitized mothers in the modern era rarely display the full breadth of disease manifestations of HDFN. In a seminal epidemiologic study of HDFN conducted in 1986, jaundice was observed in 77% of cases and hydrops fetalis in only 4%; less than 1% of cases resulted in death. Fourteen percent of neonates were premature and 17% had low birth weights. A little over half of cases represented a second or third pregnancy. Only 16% occurred during a first pregnancy. Per 10,000 live births, Hispanics comprised 12.4 cases; whites, 10.5; blacks, 8.1; and Asians, 2.2.
Rh(D) Typing: Anti-D and Antihuman Globulin Tests
Two diagnostic tests are routinely used in Rh(D) typing. The anti-D test is a hemagglutinin-based assay that detects the presence of Rh(D) antigen on red blood cells. Anti-D antibody-containing reagent is incubated with a patient’s red cells. Agglutination occurs if Rh(D) is present, allowing for classification of the red cells as D+ or D-negative. The direct antiglobulin test (DAT, or “direct Coombs test”) detects the presence of anti-D IgG antibodies on the surfaces of red blood cells. Antihuman globulin (AHG) with specificity against the Fcg portion of IgG is added to donor red cells. Agglutination occurs if the red cells contain minute amounts of Rh(D)/anti-D IgG complexes.
Blood donors identified as D-negative by the anti-D test are routinely tested for weak expression of D by DAT. Donors who are negative by both the anti-D test and by DAT are classified as D-negative, whereas donors who are negative by the anti-D test and positive by DAT are classified as weak D. Classification of donor cells as D+, D-negative, or weak D ensures that red cells that are even weakly positive for D will not be transfused to D-negative recipients. By contrast, at many institutions transfusion recipients are typed for D using only the anti-D test, and further testing for weak D is not performed. This practice ensures that weak or partial D recipients will be typed as D-negative and will receive only D-negative blood, thereby reducing the risk that a woman with a partial D phenotype will become sensitized to Rh(D) and develop anti-D alloantibodies. Using similar reasoning, the AABB (formerly the American Association of Blood Banks) does not recommend routine maternal typing for weak or partial D phenotypes in pregnancy, although hospital practice in this regard varies considerably. On the other hand, a neonate born to a D-negative mother should be typed for weak D, to ensure appropriate Rh(D) immune globulin prophylaxis of the mother.
Diagnosis of HDFN and Determination of Maternal and Fetal Rh(D) Phenotypes
The diagnostic criteria for HDFN require maternal/fetal Rh(D) phenotypic incompatibility between a D-negative mother and a D+ fetus, and a positive fetal or neonatal DAT for the presence of anti-D IgG antibodies on fetal or neonatal red blood cells. Maternal Rh(D) phenotyping is performed using the anti-D test. The fetal Rh(D) phenotype is determined noninvasively if possible, beginning with paternal Rh(D) genotyping for RHD zygosity. Alternatively, the fetal RHD genotype can be analyzed by polymerase chain reaction (PCR) of maternal blood during the second trimester. In cases of uncertainty, the gold standard for fetal Rh(D) typing is amniocentesis, which can be performed at 16 to 18 weeks’ gestation, with analysis of amniotic fluid by PCR using primers for fetal RHD . Fetal red cell destruction may be assessed by spectrophotometry, which measures spectral absorption at 450 nm as a marker of amniotic fluid bilirubin and fetal hemolysis. Fetal blood may be obtained directly for Rh(D) phenotyping using chorionic villus sampling as early as 9 to 12 weeks’ gestation or, less commonly, cordocentesis, although the risks of FMH and fetal loss are higher with these procedures than with amniocentesis. Following delivery, a DAT is routinely performed on neonatal umbilical cord blood.
Evaluation of HDFN
Pregnancies complicated by maternal/fetal Rh(D) incompatibility should undergo surveillance for high-risk clinical, laboratory, and radiographic features that may increase the likelihood of HDFN or that may indicate development of HDFN.
FMH
Any number of invasive procedures or complications of pregnancy should prompt evaluation for FMH, including trauma, amniocentesis, chorionic villus sampling, cordocentesis, abortion, miscarriage, hydrops, fetal or neonatal anemia, or a documented or perceived decrease in fetal movements (the most common prenatal presentation of FMH). The rosette test is a rapid, qualitative screening assay for FMH, in which maternal blood is mixed with anti-D–containing reagent. After excess anti-D is washed away, D+ “indicator” red cells are added, which will form rosettes around antibody-coated, D+ fetal red cells. If the rosette test is positive, a Kleihauer-Betke test is typically performed to quantify FMH volume. The Kleihauer-Betke test identifies fetal red cells based on staining with erythrosin B, which binds fetal hemoglobin F (HbF). Adequate Kleihauer-Betke testing requires manual examination of 10,000 red cells, rendering the test highly labor intensive. The Kleihauer-Betke test may also overestimate the number of fetal red cells in patients with certain hemoglobinopathies (eg, sickle cell disease or β-thalassemia) in which endogenous HbF levels are increased. Flow cytometry , using fluorescent antibodies against HbF or other fetal red cell antigens, demonstrates superior efficiency and reproducibility over Kleihauer-Betke testing, and is increasingly being used at many academic centers for accurate quantitation of fetal red cells and approximation of FMH volumes.
Antibody titers in HDFN
Baseline maternal anti-D IgG antibody titers are measured early in pregnancy and at recurring intervals of 2 to 4 weeks beginning at 18 weeks’ gestation, with anti-D titers above 16 to 32 (the ”critical titer” at most institutions) prompting initiation of more intensive fetal monitoring for HDFN.
Intensive fetal monitoring for HDFN
Techniques for measuring fetal anemia in HDFN include amniotic fluid spectrophotometry, fetal cordocentesis, fetal ultrasound (allowing for visualization of fetal size and organomegaly), and Doppler ultrasonography of fetal middle cerebral artery peak systolic velocity (which correlates with fetal hemoglobin).
Prevention of HDFN: The Role of Prophylactic Rh(D) Immune Globulin
Prophylactic use of exogenous anti-D antibodies was initially developed on the basis of the protective effect of ABO mismatch in opsonizing and clearing D+ fetal red cells from the maternal circulation. In early clinical trials, the source of anti-D was whole serum from Rh(D)-immunized donors who had high titers of anti-D antibodies. Subsequent studies made use of anti-D immunoglobulin (“Rh(D) immune globulin”) harvested from pooled plasma. The approval of Rh(D) immune globulin in 1968 for widespread use in the United States and the United Kingdom heralded a marked decline in the incidence of maternal Rh(D) alloimmunization, HDFN, and fetal/neonatal morbidity.
Indications for Rh(D) immune globulin
Guidelines from the AABB, the US Preventive Service Task Force, the American Congress of Obstetricians and Gynecologists, and the Society of Obstetricians and Gynaecologists of Canada (SOGC) recommend that D-negative (or partial D) mothers receive Rh(D) immune globulin postpartum within 72 hours of delivery, unless postpartum Rh(D) typing of neonatal umbilical cord blood by the anti-D test and by DAT reveals the infant to be D-negative. Antepartum, Rh(D) immune globulin may additionally be administered at 28 weeks’ gestation in D-negative mothers with no detectable anti-D antibodies. Rh(D) immune globulin is indicated following abortion, amniocentesis, chorionic villus sampling, cordocentesis, ectopic pregnancy, molar pregnancy, or any other conditions associated with FMH. Partial efficacy may be seen with Rh(D) immune globulin administered up to 2 weeks after delivery.
Dosing of Rh(D) immune globulin
Administration of 20 μg Rh(D) immune globulin results in removal of approximately 1 mL of D+ fetal red cells from the maternal circulation. In North America the standard dose is 300 μg, with larger doses used when greater volumes of FMH are suspected. In the United Kingdom, Rh(D) immune globulin is given antenatally at 28 and 34 weeks at a dose of 100 μg, and within 72 hours postpartum at a dose of 100 μg. For sensitizing events before 20 weeks’ gestation, a 50-μg dose is given, whereas sensitizing events beyond 20 weeks are treated with a dose of 100 μg. In Australia, Rh(D) immune globulin is given antenatally at 28 and 34 weeks at a dose of 125 μg, and within 72 hours postpartum at a dose of 125 μg; for sensitizing events in the first trimester, a 50-μg dose is given, whereas sensitizing events in the third trimester are treated with a dose of 125 μg.
Complications of Rh(D) immune globulin
Rh(D) immune globulin has been linked to two prior outbreaks of hepatitis C transmission, and is no longer produced in the United Kingdom because of epidemiologic concerns regarding prion disease. Current formulations of Rh(D) immune globulin, however, undergo multiple viral inactivation steps and are considered to be extremely safe. While the predominant antibody isotype in Rh(D) immune globulin is IgG, small amounts of IgA and/or IgM may be present, and occasionally cause systemic reactions. Rh(D) immune globulin can cross the placental barrier, although the risk of fetal complications from this is small.
Possible mechanisms underlying the efficacy of Rh(D) immune globulin include macrophage clearance of anti-D IgG-opsonized fetal red cells, inhibition of T cells required for development of endogenous anti-D antibodies, inhibition of B-cell function, sequestration of Rh(D) epitopes through binding of exogenous anti-D antibodies (a phenomenon known as steric hindrance), and alteration of processing and presentation of Rh(D) in the adaptive immune response. Monoclonal anti-D antibodies, produced from human or murine cells, have been developed but have not been as effective as Rh(D) immune globulin in multiple clinical trials, possibly because of posttranslational modifications including aberrant glycosylation.
Treatment of HDFN
Rh(D) immune globulin is ineffective in cases where maternal anti-D alloantibodies have already formed. In such instances, intensive monitoring for the onset and progression of HDFN is indicated, with therapy directed at specific disease manifestations.
Neonatal hyperbilirubinemia
The major risks of fetal or neonatal hyperbilirubinemia are neurotoxicity and kernicterus. First-line treatment is neonatal phototherapy. Maternal injection of phenobarbital, which stimulates hepatic bilirubin conjugation, has also been used. In severe cases, postpartum neonatal exchange transfusion is effective at reducing neonatal bilirubin levels, although procedure-associated complications are seen in approximately 10% of patients (eg, bradycardia from calcium infusion, portal vein and other thromboses, infection, and necrotizing enterocolitis). Due to the immaturity of the neonatal immune system, neonatal transfusions carry a risk of transfusion-associated graft-versus-host disease (TA-GVHD). The use of irradiated red cells is therefore mandatory in this setting to destroy contaminating donor leukocytes. In light of these risks, the American Academy of Pediatrics recommends exchange transfusion only for neonates with severe hyperbilirubinemia or acute kernicterus.
Fetal anemia
Intrauterine transfusion (IUT) has significantly advanced the care of fetuses with severe HDFN, yielding fetal and neonatal survival rates of over 70% to 90%. IUT is indicated at 18 to 35 weeks’ gestation in fetuses with HDFN and severe anemia (defined as fetal hematocrit of less than 25% at 18–26 weeks’ gestation, or less than 30% after 26 weeks), with a target posttransfusion hematocrit of 35% to 40%. Two infusion routes are used: intraperitoneal (IPT), in which blood is injected into the fetal intraperitoneal space, or intravascular (IVT), in which blood is transfused directly into the fetal umbilical circulation. IVT is generally preferred over IPT based on data from case-control studies. IUT carries a risk of TA-GVHD, particularly in infants who undergo both IUT and postpartum neonatal exchange. IUT has also been associated with maternal alloantibody formation against donor and fetal antigens, even with adequate cross-matching. Donor blood must be O-negative, D-negative, CMV seronegative, and irradiated. Packed red cells are used to reduce transfusion volume. Alternative approaches to IUT include the use of autologous blood from maternal donors, which may be more effective than allogeneic blood at stabilizing fetal hemoglobin levels but carries a higher risk of TA-GVHD due to maternal/fetal HLA haploidentity, and intrauterine exchange transfusion via percutaneous umbilical cord sampling.
Maternal alloantibody titers
Plasmapheresis of maternal blood was historically used in severe cases of HDFN to reduce alloantibody titers, although this technique largely disappeared from use following the advent of IUT. Maternal administration of intravenous immunoglobulin (IVIG), with or without plasmapheresis, may also reduce maternal alloantibody titers and postpartum exchange transfusion requirements.
HDFN from Alleles Other than Rh(D)
Maternal/fetal phenotypic incompatibility in K antigen (of the Kell blood group) represents 10% of all cases of HDFN; incompatibility in Rh(c) represents 3.5%. Up to 40% of K-sensitized pregnancies develop HDFN, typically with severe disease manifestations. While fetal anemia is severe in anti-K HDFN, neonatal hyperbilirubinemia and fetal reticulocytosis are not as profound as in classic anti-D HDFN, suggesting that suppression of erythropoiesis rather than hemolysis may underlie the pathology of anti-K HDFN. Rarely, severe HDFN can also be seen with phenotypic incompatibility in Rh(E), Rh(c), or Colton antigens. Mismatches in Rh(C), Rh(e), and other antigens of the MNS, Duffy, Kidd, and Sciana blood groups are unlikely to cause HDFN, owing to reduced immunogenicity and antigenic frequency. Incompatibility in Le a or Le b of the Lewis group, or P1 of the P group, leads to a predominantly IgM response and does not cause HDFN. Finally, maternal/fetal ABO mismatch, while reducing the risk of HDFN from Rh(D) incompatibility, can itself cause HDFN. Such disease is usually mild, although severe forms have been reported.
Fetal and neonatal alloimmune thrombocytopenia
More than 60 years ago, a rare condition of “congenital thrombocytopenic purpura” or “thrombocytopenic purpura in the newborn” was first reported, characterized by neonatal petechiae, purpura, gastrointestinal bleeding, and neurologic abnormalities, occurring hours to days after birth. Studies of the related adult condition of posttransfusion purpura, in the context of the evolving model of HDFN, led to the identification of maternal antiplatelet alloantibodies with specificity against paternally derived fetal platelet antigens as the cause of neonatal thrombocytopenia without maternal thrombocytopenia (renamed “fetal/neonatal alloimmune thrombocytopenia,” or FNAIT). Large-scale studies have since established FNAIT as the single most common cause of neonatal thrombocytopenia, representing approximately 20% to 70% of all documented cases, with an incidence in the general population of 1 in 1000 to 1 in 3000 live births.
Pathogenesis of FNAIT
Following occult exposure of fetal platelets to the maternal circulation, maternal alloantibodies are generated against paternally derived fetal platelet antigens that are absent in the maternal host. Such antigens are expressed by the fetus as early as 16 weeks’ gestation and reach adult levels by 18 to 26 weeks. That the severity of FNAIT appears to increase with subsequent pregnancies suggests that a primary antibody response results from the initial platelet antigen exposure, followed by an anamnestic response on antigen reexposure. Maternal IgG antibodies against fetal platelet antigens cross the placental barrier, leading to complement-mediated destruction of fetal platelets. The fetal/neonatal Fc receptor (FcRn) has been suggested in murine models to mediate transplacental transfer of maternal antibodies in FNAIT and possibly other alloimmune or autoimmune diseases, including HDFN, lupus, and immune thrombocytopenia (ITP).
FNAIT differs from HDFN in several important respects. Whereas HDFN is predominantly a disease of second or later pregnancies, 25% to 60% of cases of FNAIT occur during first pregnancy. Fetal platelet antigens induce higher rates of maternal alloimmunization than Rh or other red cell antigens. Among first-time pregnancies with maternal/fetal platelet antigen incompatibility, maternal antiplatelet antibodies can be detected in up to 50%, although most antiplatelet alloantibodies that arise during pregnancy will not lead to FNAIT, as transient alloantibodies are frequently observed. Whereas initial maternal Rh immunization usually occurs at the time of delivery, maternal antibodies directed against fetal platelet antigens can be detected as early as 20 weeks’ gestation in a first-time pregnancy, or several weeks earlier for a second or later pregnancy.
Biology of Platelet Antigens in FNAIT: the GP Ia/IIa, GP Ib/IX/V, and GP IIb/IIIa Receptors
Twenty-four human platelet antigens (HPA) have been recognized by the International Society of Blood Transfusion (ISBT) and the International Society on Thrombosis and Haemostasis (ISTH). Eleven encode polymorphisms in surface glycoprotein (GP) IIIa, while 9 represent variants of GP Ia, GP Ib, or GP IIb. These glycoproteins constitute critical components of platelet cell surface receptors, whose interactions form the basis for platelet hemostatic function. Mutations in GP Ia, GP Ib, or GP IIIa associated with FNAIT are thought to disrupt areas of the proteins corresponding to key epitopes recognized by the adaptive immune response, as illustrated by biochemical and crystallographic studies of HPA-1a in complex with the major histocompatibility (MHC) Class I molecule HLA-DRB3*0101. The HPAs are numbered according to the order of their discovery; 12 are classified into 6 “biphenotypic” systems, each comprising one of two distinct antigenic phenotypes (eg, HPA-1a/1b, HPA-2a/2b, HPA-3a/3b, HPA-4a/4b, HPA-5a/5b, HPA-15a/15b). Such biphenotypic allelism is a central component of the pathogenesis of FNAIT, as the appearance of one allele in an individual leads to host antibodies directed against the other allele. Hence, most cases of FNAIT are associated with antigenic mismatch in 1 of the 6 biphenotypic HPAs, although many exceptions have been reported.
Among Caucasians, the HPA-1b allele is uncommon, occurring in only 2%. Approximately three-quarters of FNAIT cases in Caucasians arise as a consequence of maternal anti–HPA-1a alloantibodies in HPA-1b mothers carrying HPA-1a fetuses. Coexistence of maternal HPA-1a antibodies with the MHC Class I allele phenotype HLA-DRB3*0101 appears to be a prerequisite for development of anti-HPA-1a–mediated FNAIT. Such allelic restriction is thought to arise from binding interactions between HPA-1a peptides and HLA-DRB3*0101 within the antigen/MHC Class I complex that serve to enhance antigenicity of HPA-1a epitopes.
Phenotypic incompatibility in several other platelet antigens beyond HPA-1 can give rise to FNAIT, although disease penetrance and severity vary considerably across different populations. Among Caucasians and Asians, alloantibodies to HPA-5b are the most common maternal antibodies in pregnancy yet only rarely cause FNAIT. When FNAIT has been reported in association with anti–HPA-5b, the disease has been severe. The vast majority of FNAIT in Japan and east Asia is caused by antibodies to HPA-4b ; paradoxically, HPA-4a is the most prevalent allele in the Japanese population. Anti-HPA/MHC restrictions have been observed with some, but not all, of the other HPAs implicated in FNAIT. Finally, there have been multiple reports of FNAIT from anti-HLA antibodies arising in the context of maternal/fetal HLA incompatibility, although it remains controversial whether anti-HLA antibodies play a direct role in the pathogenesis of FNAIT, in part due to the ubiquity of the HLA antigens in contrast to the specificity of platelet effects seen in FNAIT.
Clinical Features of FNAIT
Petechiae, purpura, and ecchymoses may be seen in up to 90% of newborns with FNAIT. Neonatal platelet counts are less than 50 × 10 9 /L in 50% to 90% of cases. A minority of affected neonates have mucosal bleeding. The most feared complication is intracranial hemorrhage (ICH), occurring in approximately 10% to 20% of cases, and fatal in up to one-third. Most instances of ICH develop in utero, some as early as 16 to 20 weeks’ gestation. Major risk factors for ICH include severe neonatal thrombocytopenia (defined as a platelet count of <20 × 10 9 /L), multiparity, and history of fetal/neonatal ICH in prior pregnancies complicated by FNAIT.
Diagnosis of FNAIT
The diagnosis of FNAIT requires a fetal or neonatal platelet count less than 50 × 10 9 /L, maternal/fetal HPA incompatibility, and maternal antiplatelet alloantibodies with specificity against fetal platelet antigens. HPA typing is performed using enzyme-linked immunosorbent assay (ELISA) and other fluorescence-based assays. Paternal HPA testing can suffice for fetal testing in many cases. Antiplatelet alloantibodies are detected using the monoclonal antibody-specific immobilization of platelet antigens (MAIPA) test, an ELISA-based assay that uses preformed monoclonal antibodies against specific platelet antigens to determine host antiplatelet alloantibody specificity. To establish a diagnosis of FNAIT, other conditions associated with fetal or neonatal thrombocytopenia must be excluded, including congenital TORCH (Toxoplasmosis/Other/Rubella/Cytomegalovirus/Herpes simplex) and perinatal infections (eg, Escherichia coli , Group B Streptococcus , and Haemophilus influenzae ), autoimmune diseases (eg, lupus and ITP associated with maternal thrombocytopenia), severe HDFN, thrombosis, leukemia and other malignancies, aneuploidy (eg, trisomies in 13, 18, and 21), Wiskott-Aldrich syndrome, vascular anomalies (eg, Kassabach-Merritt and Klipel-Trenaunay-Weber syndromes), congenital thrombocytopenia syndromes, and fetal emergencies (eg, preeclampsia and HELLP syndrome [Hemolysis, Elevated Liver enzyme, Low Platelet]).
Prevention of FNAIT
Two large-scale studies in Scotland and Norway, encompassing more than 25,000 and 100,000 births, respectively, explored the feasibility of population-wide screening for HPA-1a. In both studies, pregnant women were screened for the HPA-1a allele; those negative for HPA-1a were additionally screened for the presence of serum anti–HPA-1a antibodies and subjected to further interventions if anti–HPA-1a antibodies were detected (eg, cesarean section to avoid the trauma of vaginal delivery, in the Norwegian study). From a cost perspective, the results were not entirely supportive of universal screening. However, a marked reduction in morbidity in comparison with historical controls was seen among severe cases of FNAIT in the Norwegian study (∼5% vs ∼20%). At present, efforts are under way to evaluate vaccination as a means of FNAIT prevention.
Therapies for FNAIT and Natural History After Delivery
First-line treatment for FNAIT is maternal infusion of IVIG at a dose of 1 g/kg per week, beginning at 12 to 20 weeks’ gestation, and continuing until delivery. Response rates to IVIG are 25% to 60%. For high-risk neonates (defined as those with severe thrombocytopenia or with a family history of a previous sibling with fetal ICH), either IVIG 1 g/kg/d plus a steroid (prednisone 0.5–1 mg/kg/d or methylprednisolone 1 mg every 8 hours) or IVIG at a dose of 2 g/kg/d is used, although the specific role of steroids is uncertain. A risk-adapted approach for high-risk neonates has also been reported, incorporating escalating doses of IVIG and steroids based on the gestational age at which prior siblings developed ICH. In cases of HPA-1a–associated FNAIT refractory to IVIG and steroids, IUT into the fetal umbilical vein may be performed, with a fetal mortality rate of 15%. IUT in treatment of high-risk FNAIT uses either HPA-1a–negative or maternal platelets that have been washed to remove alloantibodies prior to transfusion. Following any of these interventions, cordocentesis is performed to monitor fetal platelet counts, with a target platelet count of 100 × 10 9 /L. After delivery, neonatal thrombocytopenia may persist for up to several weeks, treatment of which is typically IVIG (1 g/kg) with or without steroids, with a target platelet count of 30 × 10 9 /L.
Fetal and neonatal alloimmune thrombocytopenia
More than 60 years ago, a rare condition of “congenital thrombocytopenic purpura” or “thrombocytopenic purpura in the newborn” was first reported, characterized by neonatal petechiae, purpura, gastrointestinal bleeding, and neurologic abnormalities, occurring hours to days after birth. Studies of the related adult condition of posttransfusion purpura, in the context of the evolving model of HDFN, led to the identification of maternal antiplatelet alloantibodies with specificity against paternally derived fetal platelet antigens as the cause of neonatal thrombocytopenia without maternal thrombocytopenia (renamed “fetal/neonatal alloimmune thrombocytopenia,” or FNAIT). Large-scale studies have since established FNAIT as the single most common cause of neonatal thrombocytopenia, representing approximately 20% to 70% of all documented cases, with an incidence in the general population of 1 in 1000 to 1 in 3000 live births.
Pathogenesis of FNAIT
Following occult exposure of fetal platelets to the maternal circulation, maternal alloantibodies are generated against paternally derived fetal platelet antigens that are absent in the maternal host. Such antigens are expressed by the fetus as early as 16 weeks’ gestation and reach adult levels by 18 to 26 weeks. That the severity of FNAIT appears to increase with subsequent pregnancies suggests that a primary antibody response results from the initial platelet antigen exposure, followed by an anamnestic response on antigen reexposure. Maternal IgG antibodies against fetal platelet antigens cross the placental barrier, leading to complement-mediated destruction of fetal platelets. The fetal/neonatal Fc receptor (FcRn) has been suggested in murine models to mediate transplacental transfer of maternal antibodies in FNAIT and possibly other alloimmune or autoimmune diseases, including HDFN, lupus, and immune thrombocytopenia (ITP).
FNAIT differs from HDFN in several important respects. Whereas HDFN is predominantly a disease of second or later pregnancies, 25% to 60% of cases of FNAIT occur during first pregnancy. Fetal platelet antigens induce higher rates of maternal alloimmunization than Rh or other red cell antigens. Among first-time pregnancies with maternal/fetal platelet antigen incompatibility, maternal antiplatelet antibodies can be detected in up to 50%, although most antiplatelet alloantibodies that arise during pregnancy will not lead to FNAIT, as transient alloantibodies are frequently observed. Whereas initial maternal Rh immunization usually occurs at the time of delivery, maternal antibodies directed against fetal platelet antigens can be detected as early as 20 weeks’ gestation in a first-time pregnancy, or several weeks earlier for a second or later pregnancy.
Biology of Platelet Antigens in FNAIT: the GP Ia/IIa, GP Ib/IX/V, and GP IIb/IIIa Receptors
Twenty-four human platelet antigens (HPA) have been recognized by the International Society of Blood Transfusion (ISBT) and the International Society on Thrombosis and Haemostasis (ISTH). Eleven encode polymorphisms in surface glycoprotein (GP) IIIa, while 9 represent variants of GP Ia, GP Ib, or GP IIb. These glycoproteins constitute critical components of platelet cell surface receptors, whose interactions form the basis for platelet hemostatic function. Mutations in GP Ia, GP Ib, or GP IIIa associated with FNAIT are thought to disrupt areas of the proteins corresponding to key epitopes recognized by the adaptive immune response, as illustrated by biochemical and crystallographic studies of HPA-1a in complex with the major histocompatibility (MHC) Class I molecule HLA-DRB3*0101. The HPAs are numbered according to the order of their discovery; 12 are classified into 6 “biphenotypic” systems, each comprising one of two distinct antigenic phenotypes (eg, HPA-1a/1b, HPA-2a/2b, HPA-3a/3b, HPA-4a/4b, HPA-5a/5b, HPA-15a/15b). Such biphenotypic allelism is a central component of the pathogenesis of FNAIT, as the appearance of one allele in an individual leads to host antibodies directed against the other allele. Hence, most cases of FNAIT are associated with antigenic mismatch in 1 of the 6 biphenotypic HPAs, although many exceptions have been reported.
Among Caucasians, the HPA-1b allele is uncommon, occurring in only 2%. Approximately three-quarters of FNAIT cases in Caucasians arise as a consequence of maternal anti–HPA-1a alloantibodies in HPA-1b mothers carrying HPA-1a fetuses. Coexistence of maternal HPA-1a antibodies with the MHC Class I allele phenotype HLA-DRB3*0101 appears to be a prerequisite for development of anti-HPA-1a–mediated FNAIT. Such allelic restriction is thought to arise from binding interactions between HPA-1a peptides and HLA-DRB3*0101 within the antigen/MHC Class I complex that serve to enhance antigenicity of HPA-1a epitopes.
Phenotypic incompatibility in several other platelet antigens beyond HPA-1 can give rise to FNAIT, although disease penetrance and severity vary considerably across different populations. Among Caucasians and Asians, alloantibodies to HPA-5b are the most common maternal antibodies in pregnancy yet only rarely cause FNAIT. When FNAIT has been reported in association with anti–HPA-5b, the disease has been severe. The vast majority of FNAIT in Japan and east Asia is caused by antibodies to HPA-4b ; paradoxically, HPA-4a is the most prevalent allele in the Japanese population. Anti-HPA/MHC restrictions have been observed with some, but not all, of the other HPAs implicated in FNAIT. Finally, there have been multiple reports of FNAIT from anti-HLA antibodies arising in the context of maternal/fetal HLA incompatibility, although it remains controversial whether anti-HLA antibodies play a direct role in the pathogenesis of FNAIT, in part due to the ubiquity of the HLA antigens in contrast to the specificity of platelet effects seen in FNAIT.
Clinical Features of FNAIT
Petechiae, purpura, and ecchymoses may be seen in up to 90% of newborns with FNAIT. Neonatal platelet counts are less than 50 × 10 9 /L in 50% to 90% of cases. A minority of affected neonates have mucosal bleeding. The most feared complication is intracranial hemorrhage (ICH), occurring in approximately 10% to 20% of cases, and fatal in up to one-third. Most instances of ICH develop in utero, some as early as 16 to 20 weeks’ gestation. Major risk factors for ICH include severe neonatal thrombocytopenia (defined as a platelet count of <20 × 10 9 /L), multiparity, and history of fetal/neonatal ICH in prior pregnancies complicated by FNAIT.
Diagnosis of FNAIT
The diagnosis of FNAIT requires a fetal or neonatal platelet count less than 50 × 10 9 /L, maternal/fetal HPA incompatibility, and maternal antiplatelet alloantibodies with specificity against fetal platelet antigens. HPA typing is performed using enzyme-linked immunosorbent assay (ELISA) and other fluorescence-based assays. Paternal HPA testing can suffice for fetal testing in many cases. Antiplatelet alloantibodies are detected using the monoclonal antibody-specific immobilization of platelet antigens (MAIPA) test, an ELISA-based assay that uses preformed monoclonal antibodies against specific platelet antigens to determine host antiplatelet alloantibody specificity. To establish a diagnosis of FNAIT, other conditions associated with fetal or neonatal thrombocytopenia must be excluded, including congenital TORCH (Toxoplasmosis/Other/Rubella/Cytomegalovirus/Herpes simplex) and perinatal infections (eg, Escherichia coli , Group B Streptococcus , and Haemophilus influenzae ), autoimmune diseases (eg, lupus and ITP associated with maternal thrombocytopenia), severe HDFN, thrombosis, leukemia and other malignancies, aneuploidy (eg, trisomies in 13, 18, and 21), Wiskott-Aldrich syndrome, vascular anomalies (eg, Kassabach-Merritt and Klipel-Trenaunay-Weber syndromes), congenital thrombocytopenia syndromes, and fetal emergencies (eg, preeclampsia and HELLP syndrome [Hemolysis, Elevated Liver enzyme, Low Platelet]).
Prevention of FNAIT
Two large-scale studies in Scotland and Norway, encompassing more than 25,000 and 100,000 births, respectively, explored the feasibility of population-wide screening for HPA-1a. In both studies, pregnant women were screened for the HPA-1a allele; those negative for HPA-1a were additionally screened for the presence of serum anti–HPA-1a antibodies and subjected to further interventions if anti–HPA-1a antibodies were detected (eg, cesarean section to avoid the trauma of vaginal delivery, in the Norwegian study). From a cost perspective, the results were not entirely supportive of universal screening. However, a marked reduction in morbidity in comparison with historical controls was seen among severe cases of FNAIT in the Norwegian study (∼5% vs ∼20%). At present, efforts are under way to evaluate vaccination as a means of FNAIT prevention.
Therapies for FNAIT and Natural History After Delivery
First-line treatment for FNAIT is maternal infusion of IVIG at a dose of 1 g/kg per week, beginning at 12 to 20 weeks’ gestation, and continuing until delivery. Response rates to IVIG are 25% to 60%. For high-risk neonates (defined as those with severe thrombocytopenia or with a family history of a previous sibling with fetal ICH), either IVIG 1 g/kg/d plus a steroid (prednisone 0.5–1 mg/kg/d or methylprednisolone 1 mg every 8 hours) or IVIG at a dose of 2 g/kg/d is used, although the specific role of steroids is uncertain. A risk-adapted approach for high-risk neonates has also been reported, incorporating escalating doses of IVIG and steroids based on the gestational age at which prior siblings developed ICH. In cases of HPA-1a–associated FNAIT refractory to IVIG and steroids, IUT into the fetal umbilical vein may be performed, with a fetal mortality rate of 15%. IUT in treatment of high-risk FNAIT uses either HPA-1a–negative or maternal platelets that have been washed to remove alloantibodies prior to transfusion. Following any of these interventions, cordocentesis is performed to monitor fetal platelet counts, with a target platelet count of 100 × 10 9 /L. After delivery, neonatal thrombocytopenia may persist for up to several weeks, treatment of which is typically IVIG (1 g/kg) with or without steroids, with a target platelet count of 30 × 10 9 /L.
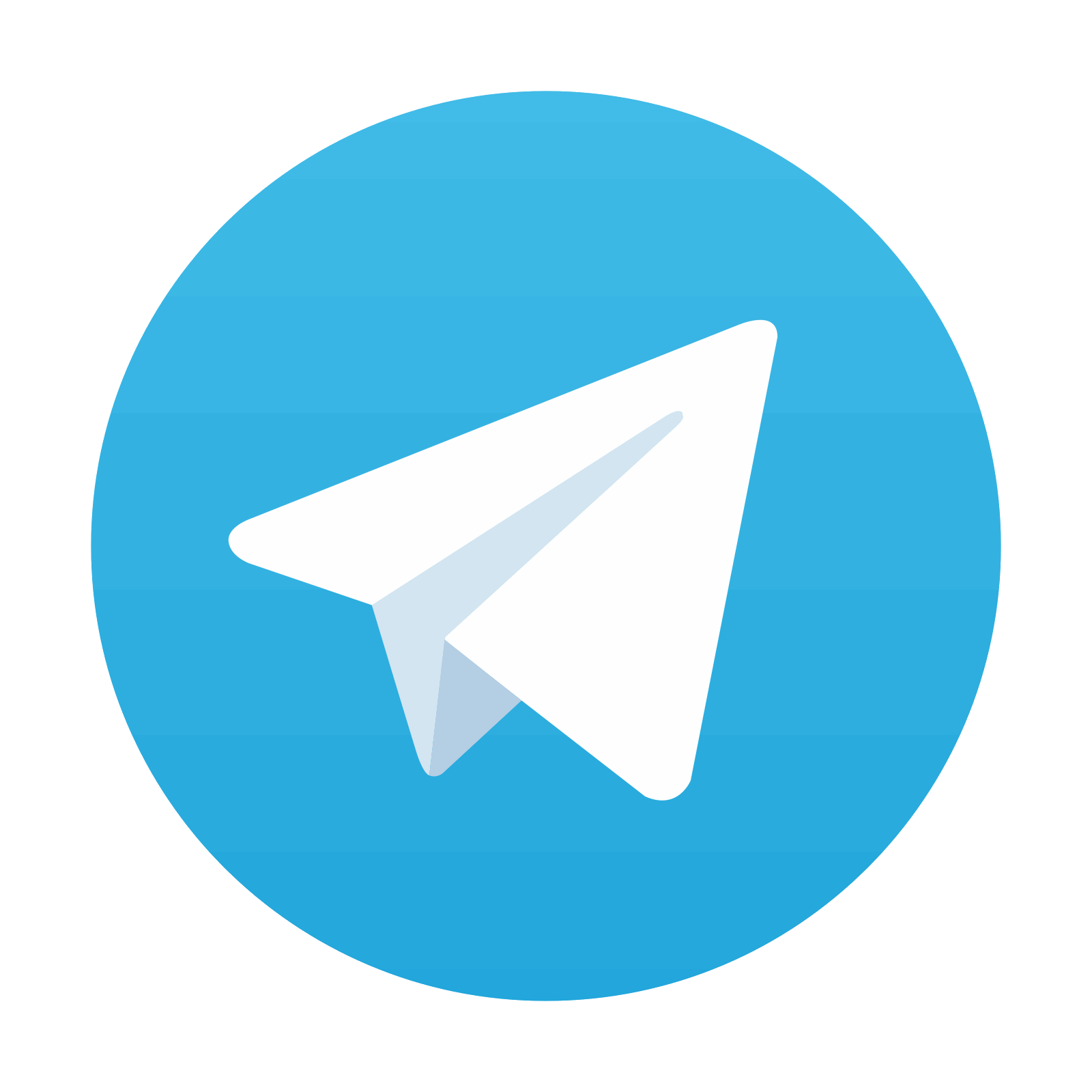
Stay updated, free articles. Join our Telegram channel

Full access? Get Clinical Tree
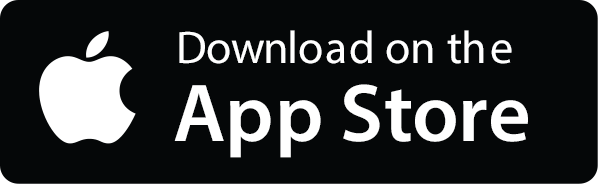
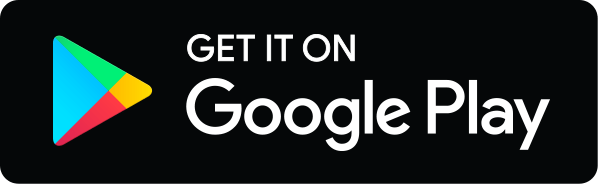