This review summarizes the currently available data concerning risk, diagnosis, and management of venous thromboembolism (VTE) in pregnant women. While the actual incidence of VTE in pregnancy remains low, the hypercoagulable state and increased risk requires clinicians to be aware of the specific aspects of presentation, diagnosis, and treatment in this population. The authors outline the strategies, efficacy, and safety of the diagnostic approach, as well as the available data concerning treatment. Considerations in treatment include maternal and fetal bleeding risk, as well as management of labor and delivery. The optimal approach to treatment requires collaboration between obstetrics, hematology, and anesthesia.
Incidence
The likelihood for venous thromboembolism (VTE) in pregnancy is increased fourfold to fivefold, with an estimated incidence of 0.76 to 1.72 per 1000 pregnancies. Two thirds of deep vein thromboses (DVT) occur antepartum and are distributed evenly between trimesters, while approximately 50% of pulmonary emboli (PE) occur postpartum. Estimates of death for PE are 1.1 to 1.5 per 100,000 deliveries in the United Sates and Europe. While there is decreased blood flow velocity in the distal legs as pregnancy progresses, the fact that DVTs are evenly distributed between trimesters argues that hormonal or coagulation changes inherent to pregnancy are the primary drivers of increased VTE risk.
Coagulation changes during pregnancy
Changes during normal pregnancy promote coagulation, decrease anticoagulation, and inhibit fibrinolysis. Specifically there is a marked increase in coagulation factors II, VII, VIII, IX, and X, as well as von Willebrand factor. There is also a decrease of physiologic anticoagulants, with protein S levels falling to 40% to 60%, starting in the first trimester and remaining decreased for 3 months postpartum. This change is attributable to an estrogen-induced decrease in total protein S and an increase in C4b, which binds protein S. The combined impact of decreased protein S and increased Factor VIII leads to increased resistance to activated protein C.
Coagulation changes during pregnancy
Changes during normal pregnancy promote coagulation, decrease anticoagulation, and inhibit fibrinolysis. Specifically there is a marked increase in coagulation factors II, VII, VIII, IX, and X, as well as von Willebrand factor. There is also a decrease of physiologic anticoagulants, with protein S levels falling to 40% to 60%, starting in the first trimester and remaining decreased for 3 months postpartum. This change is attributable to an estrogen-induced decrease in total protein S and an increase in C4b, which binds protein S. The combined impact of decreased protein S and increased Factor VIII leads to increased resistance to activated protein C.
Diagnosis: laboratory analysis
As pregnant women are at increased risk, clinicians should exercise a heightened suspicion for VTE. Making the diagnosis, however, can be complicated by the fact that reasonable alternatives to VTE also exist at higher frequencies in pregnant women. The traditional signs of VTE, such as an edematous or tender extremity, chest wall pain, shortness of breath, tachypnea, or tachycardia can occur in healthy pregnancies. To complicate matters further, the D-dimer test, which is commonly used in nonpregnant patients to indicate VTE probability, is often elevated in healthy pregnancies. Therefore, as highlighted in the following two studies, the D-dimer is not a reliable marker for determining the likelihood of gestational VTE.
In a prospective study of 800 women, who had 391 with uncomplicated pregnancies, vaginal deliveries and uneventful postpartum periods were analyzed. The D-dimer concentration increased progressively throughout gestation, peaking on postpartum day 1 and decreasing starting on postpartum day 2. A D-dimer level of 0.5 mg/L or greater is the generally accepted threshold for determining high probability versus low probability for VTE in nonpregnant patients. Between gestational weeks 13 and 20, however, more than 25% of included pregnant patients had D-dimer levels that exceeded 0.5 mg/L. After week 36, virtually all pregnant patients had D-dimer levels greater than 0.5 mg/L. These patients had no complaints consistent with symptoms of VTE.
Similar findings were seen by Kovac and colleagues, who collected D-dimer values prospectively in 89 healthy pregnancies and compared these with the D-dimer values of 12 pregnant women clinically suspected to have VTE. Among the women with healthy pregnancies, 84% had normal D-dimer levels in the first trimester. The number of women with normal D-dimer values decreased to 33% in the second trimester and was only 1% in the third trimester. Women with positive ultrasound findings for VTE, however, had a statistically significant elevation in the D-dimer level compared with controls. Thus, the investigators proposed new values for D-dimer thresholds in pregnancy that should vary with trimesters: 286, 457, and 644 ng/mL in the first, second, and third trimesters, respectively. This increased D-dimer threshold is intended to reflect the natural increase in D-dimer during gestation. This approach has not yet been validated.
Diagnosis: radiologic studies
Given the lack of reliability in pregnancy, D-dimer levels should not be used independently to diagnose or exclude VTE. The clinician therefore must also be aware of the risks and sensitivity of radiographic studies used to assess VTE in pregnancy.
Compression duplex ultrasonography of the legs is noninvasive and considered safe in pregnancy. Given the safety profile, it should be the first test obtained for any pregnant woman presenting with symptoms suggestive of VTE. Its limitations are that efficacy has not been clearly defined in the pregnant population. In addition, pregnant women may also develop iliac vein thrombosis, which would not be detected on traditional compression duplex ultrasonography. These women typically present with back pain and swelling of the entire lower limb. If a DVT is diagnosed, treatment with therapeutic anticoagulation should be started. If clinical suspicion is high for DVT but the initial ultrasound result is negative, the ultrasound scan should be repeated in 1 week. Ultrasonography should also be repeated in 1 week if a superficial thrombosis is present but the ultrasonogram is negative for DVT. If there is concern for iliac vein thrombosis, magnetic resonance venography or pulsed Doppler study is recommended.
If a PE is suspected clinically, examination of the lower extremities with duplex ultrasonography should still be the first diagnostic test performed. A definitive DVT is treated with the same intensity and duration of anticoagulation as a PE, and therefore radiologic confirmation of a PE is not required. Alternatively, if a PE is suspected but the ultrasonogram is negative for DVT, further testing is required.
The majority of trials establishing the use of radionuclide imaging to diagnose PE excluded pregnant subjects. One small study examined 120 pregnant patients with ventilation perfusion scans (VQ scans) to evaluate symptoms suggestive of PE. Eight of these women were already receiving anticoagulation at the time of the study. Of the remaining cases, 73.5% of the VQ scans were normal, 24.8% were nondiagnostic, and 1.8% were of high probability for PE. Images were evaluated by 2 independent experts. The women with normal or nondiagnostic images were not treated with anticoagulation, and the patients were followed for a mean of 20.6 months. During this follow-up interval, there was no subsequent diagnosis of PE.
This study also examined pregnancy outcomes in the women who had undergone VQ scanning. A low number of adverse pregnancy outcomes such as early or late pregnancy loss were reported for all stages of pregnancy. A low incidence of congenital structural anomalies and developmental disorders in early childhood were noted. None of these were felt to be attributable to exposure from VQ scanning. There were no childhood cancers or leukemia reported in this small study. Therefore, use of VQ scanning in these pregnant subjects was not associated with any increased risk of poor fetal outcome.
While VQ scanning has an acceptable safety profile in pregnancy, this same study highlights that one-fourth of cases will be nondiagnostic. In fact, VQ scans are known to be negative in 73% to 92% of pregnant women suspected to have PE. When a VQ scan is nondiagnostic a computed tomography (CT) scan is often required, which ultimately exposes the patient to more total radiation than if the CT scan was the first diagnostic test performed. Another advantage of the CT scan is that unlike the VQ scan, CT provides alternative diagnostic information, such as evidence of pneumonia, pulmonary edema, or aortic dissection, when PE is not confirmed. Therefore, the CT scan is the preferred tool for evaluating respiratory complaints or diagnosing PE in pregnancy.
One noninferiority randomized trial directly compared CT and VQ scans in assessing patients with high clinical suspicion for PE, based on the Wells Clinical Criteria and a positive D-dimer test. Patients with high clinical suspicion for PE but with negative or nondiagnostic images also underwent ultrasound evaluation of the legs. Although pregnant women were excluded, these data demonstrate that CT is not inferior to VQ scanning for ruling out PE. Of the 701 patients randomized to undergo CT, 19.2% were diagnosed with PE or DVT, versus 14.2% of the 716 patients randomized to the VQ arm. The difference in the percentage of VTE diagnosis was statistically significant between the two groups, raising some concern that the CT scan may be diagnosing clinically insignificant events. The primary outcome of this study was the subsequent development of symptomatic PE or proximal DVT among the patients with an initial negative image and an excluded PE diagnosis. Of these patients, 1.0% of the VQ scan patients versus 0.4% of the CT scan patients were ultimately diagnosed with VTE in the 3-month follow-up period, including one fatal PE in the VQ scan group. This difference was not statistically significant. Of note, 54.2% of patients in the VQ scan arm had nondiagnostic images. Among this group, 7.0% were ultimately diagnosed with VTE after further testing (initial ultrasound scans of the lower extremities, CT scan, or conventional pulmonary angiography), suggesting that clinicians in the study lacked confidence in a nondiagnostic VQ scan when there was high clinical suspicion of PE. Alternatively, in only 3 cases was a VQ scan ordered following a negative CT scan in a patient with high clinical suspicion of PE; in all of these cases, the VQ scan was also negative. Although these results support that fact that the VQ scan and CT scan are equivalent in diagnosing PE in the appropriate high-risk clinical group, the assignment of high risk was determined based on D-dimer testing and the Wells Clinical Criteria for PE. As previously described, these two metrics are different in the pregnant and nonpregnant populations, and therefore the authors’ recommendation favoring CT over VQ scan when chest imaging is required to assess for PE in pregnancy.
Despite this recommendation, there are no published data documenting the specificity and sensitivity of diagnosing PE by CT in pregnant patients. There is also theoretical concern that the hyperdynamic circulation and increased plasma volume of pregnancy may decrease the sensitivity. In addition, there is concern that ionizing radiation may increase the risk of fetal malignancy or other birth defects; therefore, appropriate radiation reduction strategies should be exercised. There are insufficient data on the safety of gadolinium in pregnant patients to recommend magnetic resonance angiographic techniques in assessing gestational VTE.
Despite the theoretical concern of using ionizing radiation in pregnant women, the 2004 American Congress of Obstetricians and Gynecologists (ACOG) committee guidelines state that fetal risks from radiation exposure are negligible when doses are less than 0.05 Gy. Doses of 0.1 Gy and higher were determined to result in a combined increased risk of organ malformation or childhood cancers of approximately 1%. The guidelines further estimate that the combined radiation from chest radiography, CT, and pulmonary angiography expose the fetus to around 1.5 mGy of radiation, which is well below the 0.05 Gy dose recommendation.
Other published estimates of fetal exposure from maternal CT scanning when dose reduction methods are employed confirm that the overall dose is low. Most of the literature on childhood cancers and in utero radiation exposure are case reports. These reports were reviewed by Ginsberg and colleagues who found a small, but statistically significant increased relative risk of childhood cancer when exposed to 0.05 Gy. In the cases reviewed, however, there was no increase in pregnancy loss or growth retardation.
Another study measured the amount of radiation to which a fetus would be exposed during CT pulmonary angiography scans by using an anthropomorphic phantom to represent the chest and gravid abdomen of a woman in late gestation. Three different helical scanners were studied, and estimated fetal doses ranged from 60 to 230 mGy. Strategies for reducing fetal exposure were variably effective: milliampere modulation, shielding with a lead coat, and using a 5-cm shorter scan length. These strategies reduced fetal exposure by 10%, 35%, and 56% respectively. Decreasing scan length by 5 cm excludes the bases of the lungs and therefore prevents examination of the subsegmental arteries. This strategy is predicated on the notion that thrombosis of the subsegmental vessels is associated with less morbidity and mortality.
In a 2009 quality initiatives report, The Radiological Society of North America lists methods to reduce the radiation dose of the maternal breasts and fetus at CT pulmonary angiography (CTA). These methods include lead shielding, a thin-layer bismuth breast shield, reduction in tube current and voltage, increase in pitch, increase in detector collimation thickness, reduction of Z-axis, oral barium preparation, elimination of lateral scout image, fixed injection timing rather than test run, and elimination of any additional CT sequences. Although there is less breast radiation exposure during VQ scans than with CTA, as discussed earlier VQ scans yield more nondiagnostic results. Using VQ scans as the initial diagnostic approach may therefore necessitate a second diagnostic scan using CTA, which results in more overall radiation. The chest CTA results in 0.02 to 0.06 Gy breast radiation, which can be reduced by 50% with use of breast shields. There have been no studies that document whether the breast tissue in pregnant women is more vulnerable to radiation damage than in nonpregnant women of the same age.
In summary, the risk of an undiagnosed and therefore untreated VTE in pregnancy is much higher than the risk introduced by using appropriate diagnostic tools in the pregnant patient. Clinical judgment is of chief importance and, when necessary, appropriate expert consultation should be sought to evaluate a pregnant woman presenting with signs suggestive of VTE. Obvious care should be taken to minimize the risks and exposure involved in diagnostic testing. Pregnant women suspected to have VTE should first undergo compression ultrasonography of the lower extremities to assess for DVT. If the ultrasonogram is negative in a patient with suspected DVT or superficial thrombophlebitis, the ultrasound scan should be repeated in 1 week. If the ultrasonogram is negative for DVT and PE is suspected, pulmonary imaging with CTA should be performed with radiation-minimizing practices. If VTE is diagnosed by positive extremity ultrasonogram but respiratory symptoms persist after appropriate treatment with anticoagulation, diagnostic chest imaging should be performed to investigate for alternative diagnoses. Our strategy for evaluation of symptoms suggestive of VTE in a pregnant patient is summarized in Fig. 1 .
Treatment guidelines
The most recent guidelines from the American College of Chest Physicians (ACCP) and the American Journal of Obstetrics and Gynecology Consensus Report outline treatment recommendations for VTE in pregnancy. Women who develop VTE at any point during gestation require anticoagulation for the remainder of the pregnancy and for 6 to 8 weeks postpartum. Treatment should be continued beyond 2 months postpartum if necessary to complete a minimum of 6 months’ total anticoagulation. Treatment for VTE is full-intensity anticoagulation, usually with low molecular weight heparin (LMWH), adjusted for weight changes throughout pregnancy. Fondaparinux, a pentasaccharide anticoagulant, has also been used in pregnancy, although the published case reports are limited to a small number of women unable to tolerate LMWH. Although there have been no adverse outcomes, more data are needed to document safety and efficacy of fondaparinux in pregnancy. Direct thrombin inhibitors have been demonstrated to cross the placenta in animal models, albeit with low transference, and therefore are not used in pregnant humans. Similarly, the new oral Xa inhibitor agents available for use in treating VTE in Europe and Canada have not been used in pregnant women.
The majority of pregnant patients with VTE can be safely treated at home. Their weight needs to be regularly monitored and the LMWH dose should be adjusted accordingly. The pharmacokinetics of LMWH have not been clearly defined in pregnancy, and may be variable between women. The half-life of LMWH has been demonstrated to be shorter in pregnancy, likely due to increased renal clearance. Routine monitoring of anti-Xa levels, however, is not usually performed. Exceptions are made for patients with renal insufficiency or obesity. In such patients anti-Xa levels should be checked for each dose change, change in creatinine clearance, or other parameters affecting levels, and at least once each trimester to ensure that the patient is in the desired therapeutic range. Additional information on treatment in patients with renal insufficiency is given later in a separate section. Compression stockings are recommended to prevent postphlebitic syndrome in any DVT diagnosis.
While many studies have supported the safety of both unfractionated heparin (UFH) and LMWH in pregnancy, LMWH is preferred because it requires less monitoring and is associated with less bone loss, and there have been no documented cases of heparin-induced thrombocytopenia when used in pregnancy.
Warfarin
Women receiving warfarin for VTE diagnosed before pregnancy should be transitioned to a heparin product once pregnancy is confirmed. Women receiving warfarin for reasons other than VTE (such as mechanical heart valves) are outside the scope of this review and are not necessarily transitioned to heparin for the entire pregnancy. Warfarin is not used for treatment of VTE in pregnancy because birth defects have been reported in 5% to 10% of children exposed to warfarin in utero between weeks 6 and 12. Warfarin also crosses the placenta and may therefore anticoagulate the fetus, posing the potential risk of fetal intracranial hemorrhage during delivery. Women taking warfarin for VTE who desire pregnancy should be advised to check pregnancy tests frequently, and contact their medical team with a positive test to discontinue warfarin and start heparin at that time. These women do not require transitioning from warfarin to heparin prior to a confirmed pregnancy. Women can safely receive warfarin postpartum.
LMWH Versus Heparin: Osteopenia
Long-term use of UFH at treatment doses is known to cause osteopenia. There is less risk associated with use of LMWH. One study examined bone health in 184 pregnant women who received long-term subcutaneous prophylaxis with UFH twice daily because of increased risk of VTE. The mean heparin dose was 19,100 IU in a 24-hour period, and the average duration of exposure was 25 weeks. Symptomatic osteoporotic fractures of the spine occurred in 4 women postpartum, which represented 2.2% of the total group studied. The mean heparin dose for these 4 women was 24,500 IU in 24 hours.
It is possible that the osteopenia secondary to UFH is reversible. In one study, 70 pregnant women received UFH subcutaneously for either treatment of or thromboprophylaxis against VTE. Sixty-eight of these women underwent spine and hip radiographs in the first week postpartum. Twelve of these women (17%) had osteopenia; 2 (3%) had multiple fractures. Reexamination 6 to 12 months later showed that the osteopenia was reversed in most cases. A second part of this study repeated the radiographs 3 years after gestational UFH exposure. Among the 18 women included in this delayed investigation, there was no osteopenia documented.
There is less concern for osteopenia when using LMWH as compared with UFH. One larger study undertaken to determine the effectiveness of antepartum LMWH on pregnancy outcomes included a substudy of 77 patients to evaluate the rate of osteoporosis associated with gestational LMWH exposure. The women received dalteparin and underwent bone mineral density testing. In total, 62 patients were analyzed. The intervention group received dalteparin antepartum and postpartum (mean of 212 total days); the control group received dalteparin only postpartum (mean of 38 days). There was no difference in mean bone mineral densities between the groups. Similar findings were seen in a trial of 44 pregnant women randomized to receive LMWH (dalteparin) once daily or UFH twice daily because of history of previous or current VTE. Bone mineral density was collected from each group, as well as from pregnant women who did not receive any anticoagulation. The mean bone mineral density was significantly lower in the UFH group than in the LMWH and non-anticoagulated groups. A large meta-analysis including 64 studies and 2777 total pregnancies in which LMWH was used reported one case of documented osteoporosis and postpartum vertebral fracture. This woman had received high-dose dalteparin for 36 weeks.
Bleeding
The more commonly cited concern for use of heparin products in pregnancy is bleeding. Neither UFH nor LMWH cross the placenta. Anti-Xa levels have been measured in fetal blood from mothers receiving LMWH, and showed no activity. A meta-analysis frequently cited in support of thromboprophylaxis included 64 studies and 2777 total pregnancies in which LMWH was used, for both treatment and prophylaxis. The prophylaxis group had widely variable indications for use, but in total there was a reported VTE rate of 0.84%. Given the heterogeneous diagnoses of included women, this study does not support the efficacy of LMWH for prophylaxis; however, it can be used to assess the safety profile of LMWH in pregnancy. The overall hemorrhage rate was 1.99%: 0.42% antenatal, 0.92% postpartum, and 0.65% wound hematomas.
Thrombolytic Therapy
As in nonpregnant patients, the use of thrombolytic drugs in pregnancy should be reserved for treatment of those patients with significant hemodynamic instability or compromise. In a review of 28 cases using thrombolysis in pregnancy, 7 women received treatment for PE and 3 women for DVT. The complication rate was similar to that of nonpregnant patients. Another publication reviewed use of thrombolytic medications in 172 pregnant women, with varied indications. In total there were 1.2% maternal deaths, 5.8% pregnancy losses, and 8.1% hemorrhagic complications. It has yet to be determined whether the observed pregnancy specific complications such as preterm labor, pregnancy loss, or placental abruption were caused by underlying PE, thrombolytic therapy, both, or neither.
Special Circumstances: Renal Insufficiency and Obesity
Pregnant women with renal insufficiency require close monitoring with anti-Xa levels when using either UFH or LMWH. The trough anti-Xa level should be collected just before the next dose is administered. Once a stable dosing schedule is determined by this method, anti-Xa levels should be repeated for any change in weight, anticoagulant dose, or creatinine clearance.
Anti-Xa level is preferred over partial thromboplastin time (PTT), as the PTT in pregnancy is influenced by factors other than anticoagulation alone. PTT can be attenuated by increased fibrinogen and Factor VIII activity. Thus, a pregnant patient would require a higher heparin dose to achieve the same PTT level, which may result in overdosing. This process was demonstrated in one study where known concentrations of UFH were added to the in vitro plasma samples of 13 pregnant women in their third trimesters and compared with plasma samples of 15 nonpregnant women. The PTT and anti-Xa activities were measured at increasing UFH concentrations. The anti-Xa levels more accurately correlated with change in heparin concentrations. Therefore, the anti-Xa level is preferred for monitoring UFH.
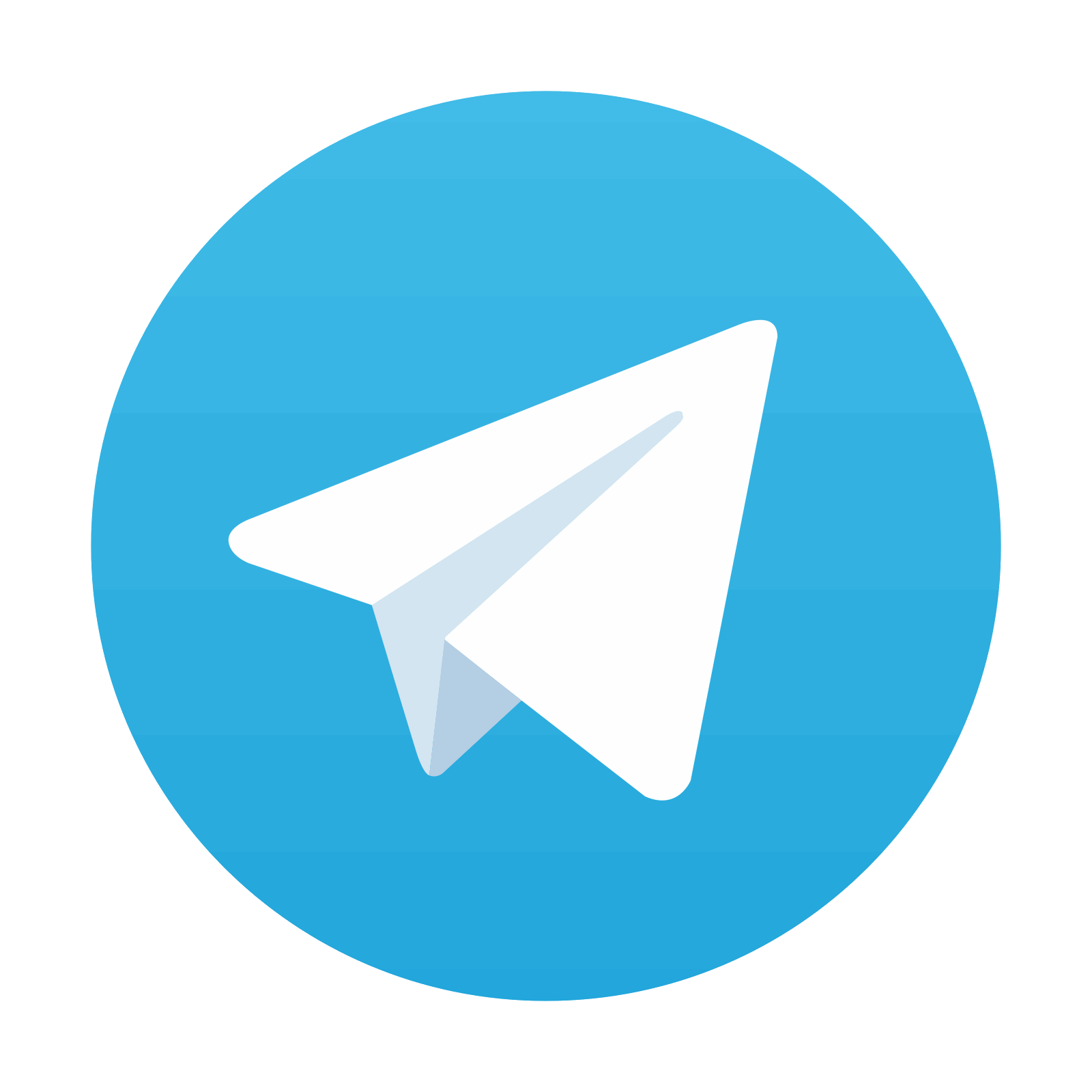
Stay updated, free articles. Join our Telegram channel

Full access? Get Clinical Tree
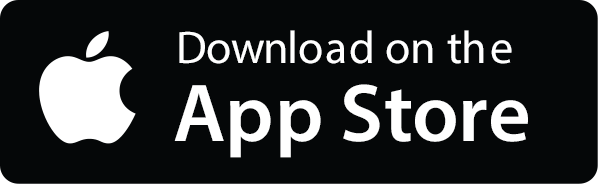
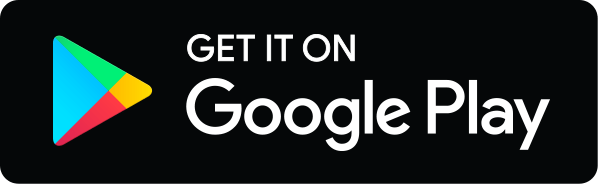