Thyroid Radionuclide Uptake and Imaging Studies
Richard L. Wahl
Introduction
Radionuclide imaging and uptake studies of the thyroid were once the most important procedures performed in nuclear medicine due to the early availability of radioactive iodine isotopes produced by cyclotrons. Further, thyroid scans were once the first step in evaluating a palpable thyroid nodule. Those days are well past, at least in the United States and other parts of the world where dietary iodine intakes are sufficient. While still very important, radionuclide imaging of the thyroid is done less commonly than before, due to the use of thyroid ultrasound and needle biopsies, which usually obviate the need for a thyroid radionuclide scan. Through the use of blood tests such as sensitive serum TSH assays, radionuclide imaging of thyroid nodules is typically limited to situations where there is concern for overactivity or autonomy of nodules. In some parts of the world, typically with borderline or low iodine nutrition, there is a high prevalence of autonomous thyroid nodules, and the thyroid scan is often done to help select the proper nodules for biopsy, even if the serum TSH is not suppressed. Even in the follow-up of known thyroid cancer, the availability of stimulated thyroglobulin blood tests has limited the number of thyroid whole body iodine scans. However, there has been considerable growth in the use of PET imaging to improve the detection and follow-up of thyroid cancers of a variety of types, especially PET/CT imaging with fluoro-2-deoxy-D-glucose (FDG). With the proliferation of imaging tests such as CT, MRI, and carotid ultrasound, as well as greater availability of diagnostic ultrasound devices in the offices of primary care providers and endocrinologists, there has been an increase in the detection of incidental, non-palpable, thyroid nodules. A small, but significant fraction of these nodules are malignant. The possibility exists that some of these smaller cancers may not be clinically relevant. The high cure rates for thyroid cancer versus its prevalence could suggest such a conclusion. Thyroid cancer incidence rates have increased nearly threefold in the past two decades with very much, though not all, of the increase in incidence among cancers less than 1 cm in diameter (1,2). In 2012, there will be an estimated 56,180 new cases of thyroid cancer diagnosed in the United States, and approximately 1,780 deaths. Cure rates have significantly increased from 93% in 1975–1977 to 97% from 1999–2005 (3). There are a variety of interesting controversies regarding radionuclide imaging in thyroid disease, and it should be realized that the field of thyroid radionuclide imaging continues to evolve, and that consensus on best practices has not yet been achieved in all settings.
Anatomy and Physiology
Thyroid Physiology
The anatomy of the thyroid, and its development and physiology are discussed elsewhere in this text. However, several elements of thyroid anatomy and physiology are relevant for an understanding of thyroid and thyroid cancer imaging, and will be reviewed here. The thyroid is located in the mid to lower neck and normally consists of two lobes. It descends from the foramen cecum during development and is formed substantially from the third and fourth branchial pouches. The descent of the developing thyroid from the foramen cecum can leave remnants of tissue along the path of descent, sometimes representing the only remaining thyroid tissue. It is the largest endocrine organ in the body and typically weights 10 to 20 g in adulthood. For radionuclide imaging, the pathways of iodine transport and organification are most important.
Table 12.1 Radionuclides Used for IN VIVO Thyroid Studies | ||||||||||||||||||||||||||||||||||||||||||||||||||||||||||||||||||
---|---|---|---|---|---|---|---|---|---|---|---|---|---|---|---|---|---|---|---|---|---|---|---|---|---|---|---|---|---|---|---|---|---|---|---|---|---|---|---|---|---|---|---|---|---|---|---|---|---|---|---|---|---|---|---|---|---|---|---|---|---|---|---|---|---|---|
|
As an essential part of thyroid hormone, iodine is a critical molecule to human growth, development, and to ongoing well-being in adulthood. The human body is extremely proficient at absorbing iodine from the gut and then storing it in the thyroid as iodinated thyroglobulin for eventual conversion to thyroid hormones. Iodine cannot be generated endogenously and all iodine in the body is from exogenous sources. Iodine absorption is rapid from the bowel, especially the small bowel (4). The Sodium/Iodide symporter (NIS) is key to iodine trapping by the thyroid, and also appears to play an important role in the absorption of iodine from the small bowel (5). This transporter is present on the apical surface of the entire small bowel. Iodine is transported across the small bowel epithelium, and then transported through the blood to the thyroid, where it is transported across the basal membrane of thyrocytes. In the normal thyroid, organification promptly follows trapping. The iodide is oxidized by thyroid peroxidase at the follicular cell colloid interface, and then binds to specific tyrosine residues in thyroglobulin. These mono- and diiodinated tyrosines (MIT, DIT) couple to form T3 and T4, which are stored in the colloid-filled follicular lumen as discussed in detail elsewhere in this book. In the United States, where diets are fairly high in iodine content, typically, 10% to 30% of ingested iodine is accumulated in the thyroid over a 24-hour period. Trapping, or “uptake”, can be blocked competitively by selected monovalent anions, e.g., perchlorate, thiocyanate, or nitrate. Organification can be blocked by the thionamide antithyroid drugs, propylthiouracil and methimazole.
Of particular interest to nuclear medicine is that NIS expression in the stomach is located at the basolateral surface of the gastric mucosa, and thus iodine is transported from the blood stream into the gastric lumen. This can result in profound iodine activity in the stomach with passage into the small bowel on radionuclide imaging studies (5). A basolateral location of NIS is also the case for the salivary glands and lactating breast tissue, which concentrate radioiodine from the blood, and which can also result in excretion (and contamination in some instances) from excreted saliva and milk.
The major radionuclide imaging agents for thyroid disease have typically been the radioactive iodine radioisotopes I-131, I-123, and more recently I-124 (a PET tracer). Tc99m pertechnetate (TcO4-) has also been used extensively. The iodine isotopes are well absorbed following oral ingestion, and while TcO4 is also absorbed after oral dosing, it is more commonly given intravenously for rapid accumulation in the thyroid. Because of the mechanisms of iodine absorption, transport into the thyroid, and organification, radioiodine species are accumulated in the thyroid to a much greater extent than is TcO4-. Although Tc pertechnetate is trapped via NIS, it washes out of the thyroid quite quickly, since it is not organified and bound to thyroglobulin. As noted above, NIS is distributed in several tissues in the body, and it is quite normal to have radioiodine uptake and some excretion by the stomach, breast tissue (especially during lactation), and salivary glands. This normal excretion of radioiodine can result in visualization of these normal tissues on imaging of the body, as well as artifacts from excreted activity contaminating normal tissues. From a practical standpoint, thyroid nodules that have increased transport and organification capabilities relative to normal thyroid are almost always benign.
Uptake of radioiodine by the normal thyroid is maximized by the sustained exposure of thyroid tissue to TSH, which increases NIS expression by thyrocytes. TSH is produced by the pituitary and elevated in hypothyroidism or by the administration of recombinant human TSH. Therefore, serum TSH levels can be very important in determining whether a thyroid scan should be done at all, and if a thyroid scan is likely to be successful in detecting benign or malignant thyroid tissue.
Radiopharmaceuticals (See Table 12.1)
Iodine
Iodine-131: There are three main radioisotopes of iodine used in thyroid imaging; they have the same biologic behavior and are typically administered orally (Table 12.1). Historically, I-131 was used most often. It has an approximate 8-day half-life, as well as a substantial beta particle emission. While I-131 can be used for imaging, currently it has a larger role in therapy of hyperthyroidism and thyroid cancer, due to its beta emission. I-131 is rarely used for diagnostic imaging of the thyroid. Its radiation dose to the thyroid is unnecessarily large, and the images are suboptimal, as the 364 keV photon emitted by I-131 has suboptimal detection by the thin crystals used in many gamma cameras. However, when I-131 is used for
therapy, posttreatment images can be of very high quality due to high photon flux and suitable collimation. Similarly, I-131 in relatively low doses, such as 2 mCi and less, can be used in thyroid cancer surveillance. Doses of 5 to 10 mCi of I-131 were used in the past for detection of thyroid cancer recurrence, but there is evidence that these doses may “stun,” or at least partly treat, the thyroid tissue, and can reduce radiotracer uptake in subsequent therapy doses. While controversial, these concerns appear to be borne out by radiation biology of irradiated thyroid cancer cells, and lower doses of I-131 should be used, if used at all, to minimize stunning (6).
therapy, posttreatment images can be of very high quality due to high photon flux and suitable collimation. Similarly, I-131 in relatively low doses, such as 2 mCi and less, can be used in thyroid cancer surveillance. Doses of 5 to 10 mCi of I-131 were used in the past for detection of thyroid cancer recurrence, but there is evidence that these doses may “stun,” or at least partly treat, the thyroid tissue, and can reduce radiotracer uptake in subsequent therapy doses. While controversial, these concerns appear to be borne out by radiation biology of irradiated thyroid cancer cells, and lower doses of I-131 should be used, if used at all, to minimize stunning (6).
Iodine-123: This isotope of iodine is increasingly used in many centers for imaging the thyroid and for imaging metastatic cancer. It has an approximately 13-hour half-life and mainly emits a 159 keV gamma photon, which is well suited to gamma camera imaging. The half-life is long enough for both membrane transport and organification of the radiotracer, resulting in a high target/background ratio. I-123 has been used less frequently than I-131 in the setting of suspected cancer recurrence. With both I-123 and I-131, given as diagnostic scans in patients with thyroid cancer, there will be a number of lesions detected only on the post-therapy scan obtained 3 to 7 days after high dose (i.e., 30–200 mCi) I-131 therapy.
Iodine-124: This isotope of iodine has both positron and gamma ray emissions. While considered a positron emitter, only about 23% of the decays are positron, and there are a large number of high-energy gamma emissions, including about 63% 603 keV gamma rays. Of particular note is that the 603 keV gamma photon is emitted simultaneously with one of the positron emissions, which serves to degrade the quality of PET images, as the energy of the photon is sufficiently close to that of a 511 keV annihilation photon from positron annihilation (annihilation of the positron upon interaction with an electron) (7,8). Despite this, excellent images can be obtained using I-124. I-124 is not yet FDA approved in the United States, but has been used in a variety of settings instead of I-123 or I-131.
TcO4-: Technetium pertechnetate is transported by the NIS and quickly accumulates in follicular cells of the thyroid following intravenous injection. Imaging with this agent is typically at 20 minutes post-injection. Because TcO4 is not organified, it rather quickly washes out from the thyroid, and therefore does not develop high target/background uptake ratios. The absolute uptake of the radiotracer is typically 1% to 2%, much lower than the levels seen with radioactive iodine. While Tc99m images suffer from rather low thyroid/background uptake ratios, they still can be quite useful, as the photon flux from an injected dose of 5 to 10 mCi of TcO4 is quite high. Some institutions use TcO4 routinely for thyroid imaging, as it is less expensive and more readily available than I-123. However, more centers in the United States now use I-123 for routine thyroid imaging, as costs have become competitive. Even if TcO4– is used, it is sometimes necessary to additionally use radioiodine, as radioiodine can have better ability to characterize occasional lesions that are Tc99m avid but Iodine non-avid, and which may potentially be malignant.
FDG: 18-F fluoro-2-deoxy-D-glucose: 18F is a positron emitter that does not have contaminating gamma emissions in the 511 keV range, and it is the mainstay of clinical PET imaging for cancer. It accumulates in tissues that preferentially use glucose and which do not have high levels of glucose 6-phosphatase activity. Many thyroid cancers will accumulate FDG in the form of FDG-6-phosphate, especially the more aggressive thyroid cancers. As some thyroid cancers become less iodine avid over time, they can become more FDG avid. FDG has also been evaluated as a diagnostic agent for thyroid nodules, where increased FDG avidity suggests a lesion as more likely to be malignant (9,10).
MIBI: (99m)Tc-methoxyisobutylisonitrile (99m)Tc-MIBI) Tc99m sestamibi is an agent mainly used to assess myocardial perfusion. However, thyroid cancers can have high perfusion and can have high accumulation of this radiotracer, in part through increased perfusion and, in part, through increased accumulation and retention in mitochondria in cells lacking surface drug resistance proteins (11). Thyroid cancers of several histologies, including medullary thyroid cancers, can be imaged with this agent in a substantial fraction of cases.
Somatostatin Receptor Binding Reagents: Both single photon and PET emitting radiotracers for detection of somatostatin receptor–expressing tumors have been utilized. In practice, In-111 pentetreotide is quite widely available. In some centers, Ga-68 labeled somatostatin receptor binding peptides such as Ga68 DOTATOC (1,4,7,10-tetraazacyclodecane-1,4,7,10-tetraacetic acid (DOTA) complexed with Tyr3 octreotide (TOC) have been applied for thyroid cancer imaging, most commonly for medullary carcinoma of the thyroid (12).
Other agents: A variety of other tracers have been used in limited studies in neuroendocrine tumors including medullary thyroid cancer (13). Thallium 201 has been used in thyroid cancer imaging in the past. It has quite unfavorable dosimetry and is not routinely used in current practice in most centers.
Instrumentation
Imaging and Detection Devices
Thyroid probe: The thyroid uptake probe is a very simple radiation detection device that quantifies thyroid radiotracer uptake, but does not provide an image. This is typically a sodium iodide crystal housed at the end of a lead lined cylinder with an open end opposite the crystal. This is coupled to an electronics module and typically to a computer. This device can detect radioactivity in a region of the body, such as the neck, but provides no anatomic information, other than that the radioactive counts come from that region of the body which is positioned directly in front of the probe system. Such systems typically are used to determine the fractional uptake of radioactive iodine in the neck, as a percentage of the administered dose. An example of a modern sodium iodide crystal probe system is shown in Figure 12.1. Factors that can have an impact on the uptake of radioiodine are shown in Table 12.2.
Rectilinear scanner: This type of scanner is now rarely used in most developed parts of the world. However, in some areas with less technologic advancement, this device, in essence a probe system which systematically scans back and forth over the body produces planar counts so that images can be obtained. Such devices can be used to depict the thyroid gland or the total body distribution of radioactive iodine, for example.
Table 12.2 Potential Interfering Conditions/Drugs which Decrease/Increase the %Raiu | ||||||||||||||||||||||||||||||||||||||||||||
---|---|---|---|---|---|---|---|---|---|---|---|---|---|---|---|---|---|---|---|---|---|---|---|---|---|---|---|---|---|---|---|---|---|---|---|---|---|---|---|---|---|---|---|---|
|
Gamma camera: A gamma camera is the most widely used imaging device for thyroid and whole body imaging. This device typically has at least one “head” that is composed of a very large radiation-sensitive crystal made of sodium iodine that is 30 cm or more in diameter. Such crystals give off light when they absorb gamma photons. Such devices have a series
of photomultiplier tubes located behind the crystal, and a lead “collimator,” a lead sheet with many parallel holes in front of the crystal to provide positioning information or magnification of the area imaged. The crystals are typically 1/4 to 5/8 inch in thickness, although crystals of 1 cm thickness are sometimes used, as they are better suited to the high energy of I-131 (364 keV). Collimators can include a pinhole collimator, typically with an aperture of 5 mm or less, to optimize resolution. Alternatively, a high energy or medium energy parallel hole collimator can be used. Such devices have inferior resolution compared to pinhole collimators, but are used for whole body or locoregional imaging, especially surveys for thyroid cancer. A modern dual head gamma camera is shown in Figure 12.2.
of photomultiplier tubes located behind the crystal, and a lead “collimator,” a lead sheet with many parallel holes in front of the crystal to provide positioning information or magnification of the area imaged. The crystals are typically 1/4 to 5/8 inch in thickness, although crystals of 1 cm thickness are sometimes used, as they are better suited to the high energy of I-131 (364 keV). Collimators can include a pinhole collimator, typically with an aperture of 5 mm or less, to optimize resolution. Alternatively, a high energy or medium energy parallel hole collimator can be used. Such devices have inferior resolution compared to pinhole collimators, but are used for whole body or locoregional imaging, especially surveys for thyroid cancer. A modern dual head gamma camera is shown in Figure 12.2.
Many modern gamma cameras are capable of rotation around patients with a single or two heads, and can acquire 32 to 64 or more projections of planar images from around the patient. The images can then be joined together into a
signogram, reconstructed using a variety of methods, and displayed as 3D images in the transverse, sagittal, or coronal views. Newer gamma cameras are integrated as hybrid devices with CT scanners as SPECT/CT cameras (Fig. 12.2). Such devices can combine images of CT and SPECT into hybrid images that can be very helpful for precisely defining the location of “hot spots,” such as those of thyroid cancer, that are iodine avid. They can also help separate excreted iodine in the bowel or bladder from malignant radiotracer uptake.
signogram, reconstructed using a variety of methods, and displayed as 3D images in the transverse, sagittal, or coronal views. Newer gamma cameras are integrated as hybrid devices with CT scanners as SPECT/CT cameras (Fig. 12.2). Such devices can combine images of CT and SPECT into hybrid images that can be very helpful for precisely defining the location of “hot spots,” such as those of thyroid cancer, that are iodine avid. They can also help separate excreted iodine in the bowel or bladder from malignant radiotracer uptake.
PET and PET/CT scanners: PET scanners are typically full ring devices through which a patient can pass for imaging. Imaging is typically performed over a large portion of the body by linearly moving the patient through the scanner in 10 to 20 cm increments. The PET scanners use “coincidence imaging” to allow determination of paired 511 keV annihilation photons which result from positron decay. Currently, 18 F fluoro-2-deoxy-D-glucose (FDG) is the most commonly used PET emitter for thyroid cancer imaging. Most modern PET scanners are combined with a CT scanner in the form of a PET/CT scanner. This device can quantitatively image the distribution of radioactivity throughout the body and localize it using the CT scan, which is obtained sequentially. An extensive set of computers is required to process the complex data sets arising from the PET/CT scan data acquisition. PET is increasingly quantitatively accurate, allowing a precise estimate of radioactivity concentration in various tissues, and providing an SUV (standardized uptake value) that reflects the metabolic activity of a tissue. A PET/CT scanner is pictured in Figure 12.3.
Table 12.3 Differential Diagnosis of Thyrotoxicosis—Increased or Decreased Raiu | |
---|---|
|
Nuclear Medicine Studies
Indications, Interpretation, and Methods
Thyroid Uptake Measurement
A very simple, but very important, non-imaging study is the determination of the fraction of ingested iodine reaching the thyroid gland 4 to 24 hours following oral administration. This test is typically performed to determine the cause of thyrotoxicosis. Patients with Graves’ disease or autonomous nodules typically have elevated radioiodine tracer uptake values. In contrast, thyrotoxic patients with subacute or painless thyroiditis have low thyroid radiotracer uptake, as do patients who are ingesting excessive quantities of thyroid hormone (Table 12.3). Thus, some patients can be thyrotoxic without being hyperthyroid, where “hyperthyroidism” is defined to mean increased thyroidal hormone synthesis and secretion. Such patients would not benefit from radioiodine therapy or antithyroid agents. The fractional uptake is also used in many centers to help determine the dose of I-131 for therapy of hyperthyroidism. It is not uncommon to perform a thyroid scan and an uptake together, the former requiring a gamma camera and a higher ingested dose, the latter requiring only the probe and a lower ingested dose. There is little reason to perform this test to assess hypothyroidism, although it is possible to diagnose congenital or acquired iodine organification defects using uptake assays before and after perchlorate administration (a perchlorate discharge test). However, sodium and potassium perchlorate are no longer available in the United States. While both I-131 and I-123 can be used, I-123 is more popular in the United States due to its more favorable dosimetry for imaging, though low doses of I-131 can be used for uptake studies without imaging.
It is also possible to perform a thyroid uptake study using Tc99 pertechnetate, but this only measures early uptake, and does not assess organification-related accumulation of the radiotracer. The lower absorbed radiation dose from Tc99m is an attractive alternative in children. Also, since the Tc99m uptake is available within 20 to 30 minutes of injection, the information can be used to help determine whether Graves’ disease or thyroiditis is present, treatment can then be given expeditiously on the same day as the uptake study. Some would argue that no uptake study is needed to treat classic Graves’ disease with radioiodine (14), but this could lead to the occasional patient with thyroiditis receiving radioiodine erroneously. With an ultrasound (including Doppler) showing a homogeneous, hyperemic, enlarged gland, a very good specificity for Graves’
disease can be present, but not quite as good as with radioiodine uptake measurements.
disease can be present, but not quite as good as with radioiodine uptake measurements.
The 2011 American Thyroid Association clinical practice guidelines on hyperthyroidism state, “A radioactive iodine uptake should be performed when the clinical presentation of thyrotoxicosis is not diagnostic of GD (Graves Disease); a thyroid scan should be added in the presence of thyroid nodularity” (15). However, it can be quite difficult to distinguish painless thyroiditis from mild Graves’ disease in the absence of eye findings. Furthermore, if radioactive iodine therapy is planned, the administered activity may be better informed by an uptake measurement. Certainly, unexpected localization of radioiodine to the breasts is an important observation which would only be detected by imaging, and is one which can change the management plan drastically, but a clinical history for lactation is usually adequate to exclude breast uptake without the need for a diagnostic scan.
Thyroid Scan (non-PET)
Thyroid scans can be performed using Tc99m pertechnetate or I-123. This author prefers I-123, but practice patterns vary. In the United States, thyroid imaging using I-131 is no longer performed, due to the high radiation dose to the thyroid and the poor imaging quality. Thyroid scans are done with a gamma camera, typically with a pinhole collimator with a 5 mm or smaller aperture. Sometimes thyroid imaging can be done with a parallel hole collimator in a magnification mode. This does not produce as high a quality an image set as can be obtained by using the pinhole collimator system, as the pinhole system allows for true magnification. The collimator aperture size limits the detection ability for hypofunctioning nodules, which is typically 1 cm. Thus, ultrasound can routinely detect smaller nodules than can be visualized by radionuclide imaging, especially nodules that are hypofunctioning. For I-123 scans, a typical dose is in the 200 to 400 uCi range, with gamma camera images obtained at 24 hours post-ingestion. Some centers routinely use Tc99m pertechnetate for scanning. It has lower cost, delivers less radiation to the thyroid gland, and quickly provides imaging, within 20 to 30 minutes of injection (16). It also is a very convenient isotope, especially in centers that have a Tc99m generator which provides the radiotracer at most any time of the day. While count rates can be high with a 10 mCi (370 MBq) dose of Tc99m, many of the counts are not from the thyroid itself, as the target to background ratio is not as high as with I-123, limiting its usefulness in patients with substernal thyroid glands or goiters. Tc99m is trapped but not organified, by the thyroid. This means there can be discrepant nodules which are “hot” or warm with Tc99m, but which are nonfunctioning or “cold” with I-123. Thus some “hot” or warm nodules on Tc99m will require follow up I-123 scans to exclude the possibility of a cancer which transports but does not progressively concentrate Tc99m.
The indications for thyroid scintigraphy (imaging) are several. In thyrotoxicosis a scan can determine if the gland has increased tracer uptake in a uniform or nonuniform pattern. In general, a higher administered activity is required to ablate a gland which has nonhomogeneous radiotracer uptake. It is our current recommendation that a thyroid uptake and radionuclide scan be routinely performed before radioiodine treatment of a patient with presumed Graves’ disease. While ultrasound has a diagnostic accuracy in the mid-90% range, there are patients in whom the diagnosis of Graves’ disease versus toxic multinodular goiter (MNG) remains uncertain. It is recognized, however, that practice patterns change slowly, and that there are likely some cases of Graves’ disease where a thyroid uptake alone, without a thyroid scan, may suffice. There are, of course, rare causes of hyperthyroidism in which imaging of the neck can help clarify the etiology. For example, sometimes the source of the thyroid hormone is ectopic, as in patients with struma ovarii.
The assessment of thyroid nodules has historically been the major indication for thyroid scintigraphy. Practice patterns and guidelines differ on the appropriateness of a thyroid scintigram as a first test in the diagnostic evaluation. In areas with fairly high dietary iodine or iodine supplementation of dietary salt, most thyroid nodules are cold on thyroid scans. While most thyroid nodules are cold, most (95%) cold thyroid nodules are benign. As indicated earlier, thyroid nodules that accumulate iodine more avidly than the surrounding normal tissue are consistently benign. However, such nodules represent only a few percent (<5%) of all nodules. Thus, in the United States, the recommended evaluation for a thyroid nodule is to obtain a serum TSH level first, and if it is subnormal, a radionuclide thyroid imaging study is generally recommended as the next diagnostic test.
The 2009 American Thyroid Association (ATA) clinical practice guidelines recommend “Measure serum TSH in the initial evaluation of a patient with a thyroid nodule. If the serum TSH is subnormal, a radionuclide thyroid scan should be performed using either technetium 99mTc pertechnetate or 123I” (17). The ATA also recommends: “Thyroid sonography should be performed in all patients with known or suspected thyroid nodules.” In fact, since many supposed solitary nodules are found to be multiple on ultrasound, biopsy of one or more suspicious-appearing, incidentally discovered nodules may be performed, even before the TSH and thyroid scans are completed. Thus, real world practice is, absent overt hyperthyroidism, to secure a biopsy very early in the work up of a patient with a palpable thyroid nodule.
Of course, fine needle aspiration (FNA) is not a perfect method. In a few percent of both “benign” and “malignant” cytology readings, there can be false positive and negative interpretations. Thus observation of patients is essential even if biopsies are negative. In addition, there are some cytologies that are indeterminate. The ATA 2009 document recommends, “If the cytology reading reports a follicular neoplasm, a 123I thyroid scan may be considered, if not already done, especially if the serum TSH is in the low-normal range. If a concordant autonomously functioning nodule is not seen, lobectomy or total thyroidectomy should be considered.” (17).
The ATA also recommends that “A low or low-normal serum TSH concentration may suggest the presence of autonomous nodule(s). A technetium 99mTc pertechnetate or 123I scan should be performed and directly compared to the US images to determine functionality of each nodule >1–1.5 cm. FNA should then be considered only for those isofunctioning (“Warm”) or nonfunctioning nodules, among which those with suspicious sonographic features should be aspirated preferentially”.
These ATA recommendations indicate that patients with thyroid nodules and normal TSH levels do not need a thyroid
scan. However, these recommendations are based on the American experience, where there is relatively high dietary iodine and a relatively low frequency of multinodular goiters. In areas of relative iodine deficiency, there is a higher proportion of functioning nodules in nodular goiters. As biopsy of these nodules could result in a large number of indeterminate results, practice recommendations with more input from European countries [e.g., American Association of Clinical Endocrinologists, Associazione Medici Endocrinologi, and European Thyroid Association (AACE/AME/ETA) Guidelines] are somewhat more liberal in their recommendations for the use of thyroid scintigraphy (17). While they acknowledge that ultrasound, ultrasensitive TSH, and FNA are the main tools for diagnosis of thyroid nodules, scintigraphy may be highly appropriate in patients with a multinodular gland and normal or mildly decreased TSH levels, especially in iodine deficient areas (18).
scan. However, these recommendations are based on the American experience, where there is relatively high dietary iodine and a relatively low frequency of multinodular goiters. In areas of relative iodine deficiency, there is a higher proportion of functioning nodules in nodular goiters. As biopsy of these nodules could result in a large number of indeterminate results, practice recommendations with more input from European countries [e.g., American Association of Clinical Endocrinologists, Associazione Medici Endocrinologi, and European Thyroid Association (AACE/AME/ETA) Guidelines] are somewhat more liberal in their recommendations for the use of thyroid scintigraphy (17). While they acknowledge that ultrasound, ultrasensitive TSH, and FNA are the main tools for diagnosis of thyroid nodules, scintigraphy may be highly appropriate in patients with a multinodular gland and normal or mildly decreased TSH levels, especially in iodine deficient areas (18).
Perhaps the greatest difference in opinion among the guidelines is the recommendation by AACE/AME/ETA that thyroid scanning has a role in the assessment of multinodular glands that are not yet causing suppression of serum TSH levels. This recommendation is based on the understanding that nodules in such glands can begin to develop autonomy over time, and be in a state where one or a few nodules may have increased function, while much of the rest of the gland is in a state of decreased function. It has been suggested that thyroid imaging under conditions of thyroid hormone dosing (which can suppress TSH-dependent nodules) can be used to diagnose “euthyroid autonomy.” Thus, the paraphrased recommendations of the AACE/AME/ETA for thyroid scintigraphy are largely similar to those of the ATA and include:
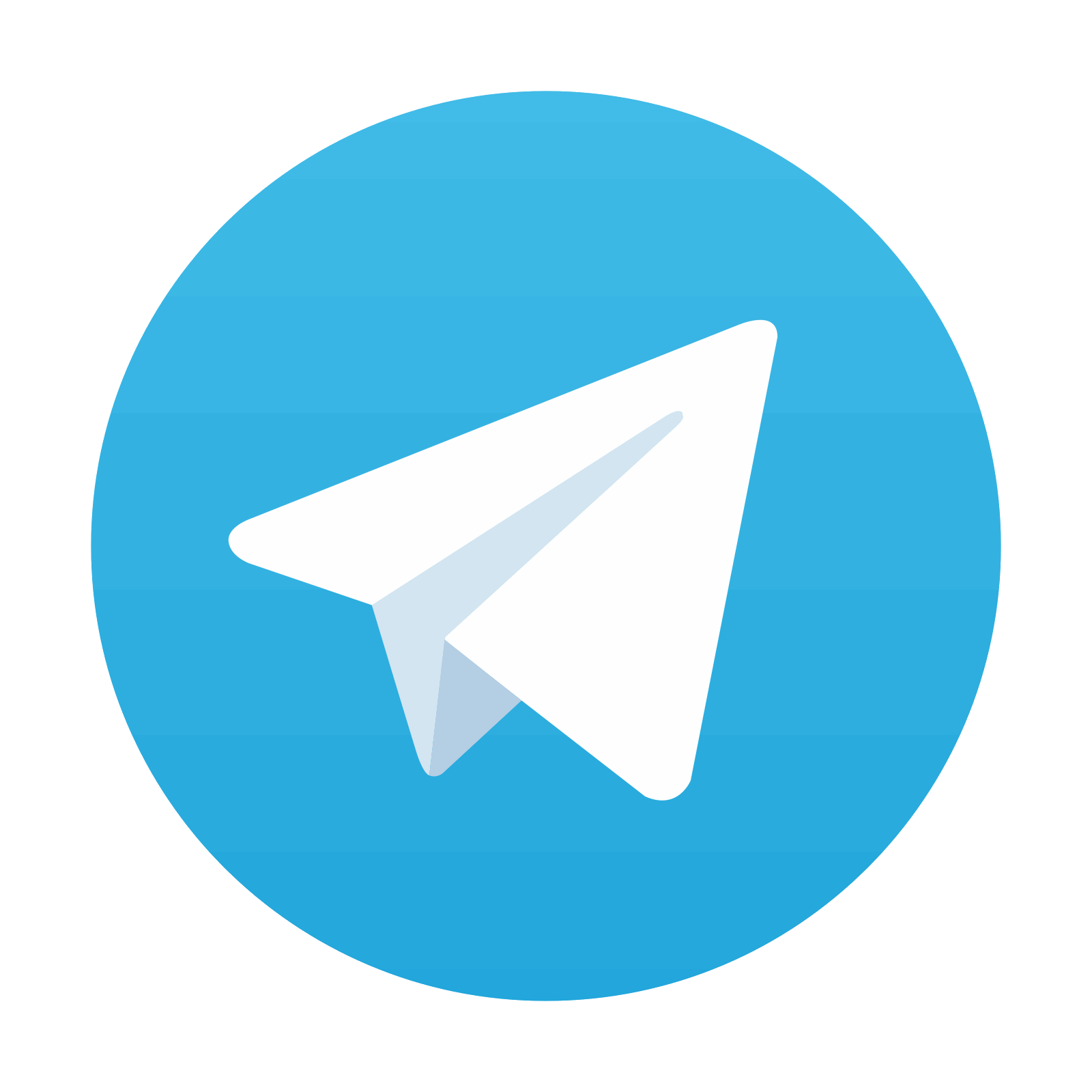
Stay updated, free articles. Join our Telegram channel

Full access? Get Clinical Tree
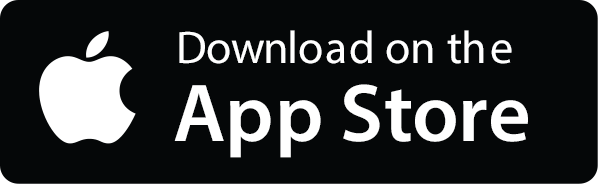
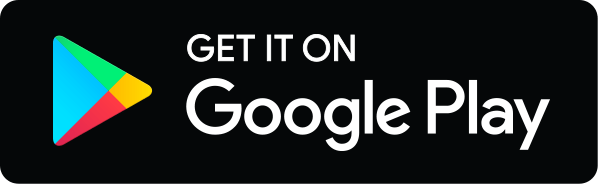
