Fig. 1
Impact of cancer on risk of venous thrombosis. Adjusted odds ratios for venous thrombosis in patients with different cancer types, with distant metastasis (mets), and at different times post-cancer diagnosis (versus non-cancer sufferers). Abbreviations: GI gastrointestinal, Haem haematological
Mechanisms that regulate cancer-induced thrombogenesis are multiple and interacting, and have been described in detail by others (Table 1) [11, 15, 24–30]. Aberrantly glycosylated mucins, for example, are pro-coagulant glycoproteins secreted into the blood by tumour epithelial cells, which act as selectin ligands, and mediate the interaction between platelets, white cells, and endothelial cells [16]. Distinct selectin binding sites are present on these mucins, and regulate adhesion between circulating tumour cells and other vascular cells known to contribute to thombosis (e.g. platelets) [16].
Table 1
Mechanisms that regulate cancer-induced thrombus formation
Mechanism | Effect | Refs. | |
---|---|---|---|
Acute | Malignancy leads to increases in the expression and activation of inflammatory molecules in cancer and endothelial cells | Inflammatory molecules such as TNF activate the coagulation pathway via increased TF expression or suppression of the anti-coagulant protein C pathway | |
Systemic | Cancer cells release glycosylated mucins and TF-expressing microvesicles | Mucins act as ligands for adhesive selectin molecules; TF triggers the coagulation cascade resulting in formation of cross-linked fibrin | |
Local | Tumour hypoxia increases the expression of pro-coagulant factors such as TF and PAI1 and supresses anti-coagulant factors such as thrombomodulin | Increased expression of coagulant factors or suppression of anti-coagulant factors stimulate thrombus formation and impair thrombolysis | |
Genetic | Coagulants such as TF and PAI1, and the thrombin receptor PAR1, are induced following activation of oncogenes or inactivation of tumour suppressor genes | Binding of thrombin to PAR1 stimulates the formation of cross-linked fibrin from fibrinogen |
Other potent pro-coagulant factors are also upregulated in the circulation of cancer patients compared with healthy individuals. Tissue factor (TF) and plasminogen activator inhibitor 1 (PAI1), for example, are increased by reductions in oxygenation (hypoxia) that commonly occur in propagating tumours [19, 20, 31]. Hypoxia has been shown to activate the early growth response 1 transcription factor, which leads to increased tissue factor expression in macrophages and smooth muscle cells, and subsequent fibrin deposition [20]. It has also been shown that cancer cells release TF-expressing microvesicles, and that these cellular fragments are activated under hypoxia [17, 18]. In one such study, for example, hypoxia led to increased expression of protease-activated receptor 2, which regulates coagulation-dependent signalling in endothelial cells, and in turn, an increase in heparin-binding EGF-like growth factor expression [18]. Furthermore, endothelial coagulant function is stimulated by hypoxia, e.g. via suppression of the anti-coagulant factor, thrombomodulin [21]. In this study, reduced oxygen levels resulted in increases in Factor X activation and endothelial cell size, as well as reductions in endothelial intracellular gaps [21].
Activation of oncogenes (such as K-ras and MET), or inactivation of tumour suppressor genes (such as p53 or PTEN) can also increase the expression of pro-coagulants PAI1, and the expression and activity of TF [15, 22, 23]. The elevated inflammatory status in cancer patients also potentiates thrombus formation [11]. Pro-thrombotic cytokines such as tumour necrosis factor are highly expressed by cancer cells and acutely elevated in activated endothelial cells. The roles of this and other cancer-induced and pro-coagulant inflammatory mediators in thrombus formation, however, warrant further investigation [11].
2.1 Clinical and Future Perspectives
Most DVT patients are currently treated with low molecular weight heparin and warfarin, with few receiving additional treatment to remove the thrombus by thrombolysis or thrombectomy. Anticoagulation allows slow natural resolution of the thrombus, but does not reduce the incidence of post-thrombotic syndrome or accelerate resolution, and may result in excessive bleeding [32–34]. Thrombolytic agents are contraindicated in some cases, and this treatment also increases the risk of bleeding [35]. Recent advances in catheter-directed thrombolysis with or without pharmacomechanical adjuncts may increase the efficacy of lysis, reduce the amount of lytic agent used, and with it, the risk of bleeding. Catheter-directed or pharmacomechanical thrombolysis has been used in selected patients with symptomatic iliofemoral DVT [36]. There is, however, a paucity of data regarding their effectiveness in patients without cancer, and currently no study examining their effect in this group of patients.
Although therapies under development for the treatment of DVT often aim to target specific molecular pathways, it may be that complex pathologies (such as Trousseau’s syndrome) would be better combatted using treatments with multiple actions [15]. Heparin, for example, is the preferred treatment for patients with Trousseau’s syndrome, while vitamin K antagonists, or thrombin inhibitors, appear insufficient in many cases [15]. The primary actions of heparin are to irreversibly inactivate coagulant factor Xa and thrombin, which impairs platelet activation and thrombus formation. Heparin may also reduce cancer-induced thrombus formation by: (i) activating heparin cofactor II and protein C [37, 38]; (ii) inhibiting the adhesion molecules L- and P-selectin [39, 40]; and (iii) stimulating the release of TF pathway inhibitor [41]. Low molecular weight heparin, which can be administered in a single dose, is another treatment option for Trousseau’s syndrome patients; the CLOT trial, for instance, showed that low molecular weight heparin (versus warfarin) reduced the risk of recurrent thromboembolism in cancer patients, without increasing the risk of bleeding [42]. Novel oral anti-coagulants are under active investigation in clinical trials [43]. Such agents may ultimately prove effective in the prevention and treatment of venous thrombosis in cancer patients, but the effects of such agents should first be thoroughly investigated in large-scale randomised clinical trials, and compared with established treatments [44]. Combination therapies may also eventually come to fruition, although these may carry an increased incidence of side effects [15]. Future studies could investigate therapeutic targeting of the multiple pathways that regulate cancer-induced thrombus formation.
3 Cancer-Associated Thrombus Resolution
Venous thrombi resolve naturally with time, but this is a slow process that takes more than 6 months in man [45]. Veins that recanalise rapidly have preserved valve integrity and a lower incidence of venous reflux [46, 47]. Rapid recanalisation times are consequently associated with favourable clinical outcome [48]. Thrombus resolution is a complex process of intravascular remodelling, similar to the formation of granulation tissue that is crucial for wound healing [49]. Natural resolution involves recruitment of white cells (initially neutrophils then monocytes), endothelial cells and their progenitors, and myofibroblasts [49]. Vein recanalisation is achieved through a combination of new blood vessel formation in the thrombus and thrombus contraction from the vein wall [49]. The endothelial-lined channels that form within and around the thrombus coalesce and form larger vascular channels, which together with thrombus contraction help to restore blood flow [49]. Vein recanalisation and thrombus resolution are accelerated when the angiogenic response to thrombus formation is stimulated in the thrombus and surrounding vein [50–52].
3.1 Clinical and Future Perspectives
Chemotherapy increases the risk of venous thrombosis in cancer patients, but the mechanisms responsible for this observation are unclear [11]. Different types of chemotherapy carry varying thrombotic risks. For example, the addition of cyclophosphamide, methotrexate, and fluoracil to tamoxifen treatment is associated with an increased the incidence of venous thromboembolism in breast cancer patients, compared with tamoxifen alone [53]. Similarly, addition of a dexamethasone and a multi-agent chemotherapy cocktail to thalidomide treatment alone increases venous thromboembolism rate in myeloma patients [54].
Anti-angiogenic drugs have also been implicated in both thrombus formation and resolution (Fig. 2) [10, 11, 55, 56]. Treatment with bevacizumab, an antibody against vascular endothelial growth factor (VEGF) is associated with increased risk of venous thromboembolism [57–59]. Treatment with sorafenib in conjunction with another VEGF pathway inhibitor, axitinib, also increases the incidence of venous thromboembolism in patients with metastatic renal cell carcinoma compared with sorafenib treatment alone [60]. Such anti-angiogenic agents could activate endothelial cells and platelets, and thereby increase thrombus formation [11, 61]. The anti-angiogenic SU5416, for example, increases the expression of endothelial TAT complexes, F1 and 2, and E-selectin [61]. It has subsequently been suggested that VEGF-depleted endothelial cells are susceptible to chemotherapy-induced activation of the coagulation [11, 61]. Anti-angiogenic therapies also prevent thrombus resolution [55, 56]. Treatment with axitinib or 2-methoxyestradiol inhibits venous thrombus resolution in a mouse model of vena cava thrombosis [55, 56]. Both agents impair thrombus organisation, and neovascularisation, reducing vein recanalisation [55]. The ingrowth of blood vessels from the vein wall is an important part of the angiogenic response following thrombus formation [49]. Anti-angiogenic agents also inhibit the expression of angiogenic factors such as VEGF (known to accelerate resolution) [52, 62] in the surrounding vein wall, suggesting that the deleterious effect of such treatments on resolution could occur partly through suppression of angiogenic drive in the local vein [56].


Fig. 2
Potential effects of anti-angiogenic agents on cancer-associated thrombosis. Anti-angiogenic cancer agents may increase the incidence of thrombosis in cancer patients through: (a) activation of endothelial cells and platelets, which stimulates thrombus formation (e.g. via increased expression of coagulant factors including E-selectin and the thrombin/antithrombin complex); and (b) inhibition of the angiogenic response to thrombogenesis, which impairs subsequent resolution (e.g. via reduced growth factor expression in cells within and surrounding the thrombus)
Both the prothrombotic and anti-resolution side effects of anti-angiogenic treatments that could contribute to the increased thrombotic complications seen in cancer patients treated with these agents should be carefully considered when managing venous thrombosis (and subsequent complications) in cancer patients. Large-scale clinical studies of the effect of approved cancer chemotherapies on thrombus propagation are warranted. Future studies should continue to investigate pro-thrombotic mechanisms by which cancer chemotherapies (including anti-angiogenics) could enhance venous thrombosis. These studies could ultimately lead to the development of novel cancer treatments that are not associated with increased thrombotic risk.
4 Conclusions
Mechanisms that regulate cancer-associated thrombus formation are complex and multi-faceted. Cancer chemotherapies can increase the incidence of venous thrombosis, but the mechanisms responsible for this effect are not fully characterised. Although priority should be given to prevention of disease progression and increased survival in cancer patients, potentially pro-thrombotic effects of cancer treatments should be carefully considered in the clinic. A better understanding of the mechanisms that regulate cancer-induced thrombus formation and resolution may lead to the development of novel treatments for patients such as those with Trousseau’s syndrome.
References
1.
Fowkes FJ, Price JF, Fowkes FG (2003) Incidence of diagnosed deep vein thrombosis in the general population: systematic review. Eur J Vasc Endovasc Surg 25(1):1–5CrossRefPubMed
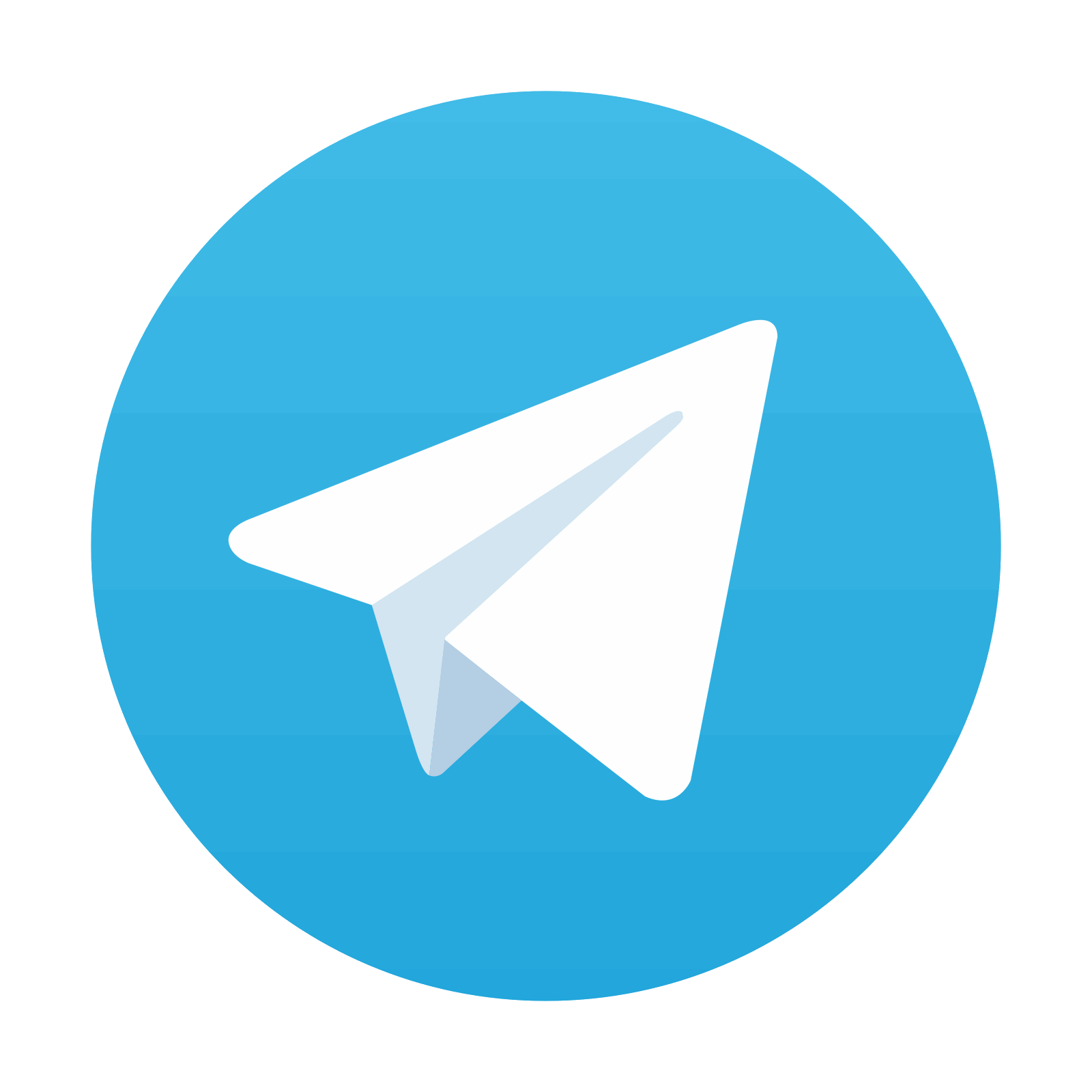
Stay updated, free articles. Join our Telegram channel

Full access? Get Clinical Tree
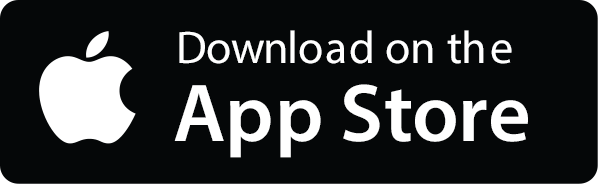
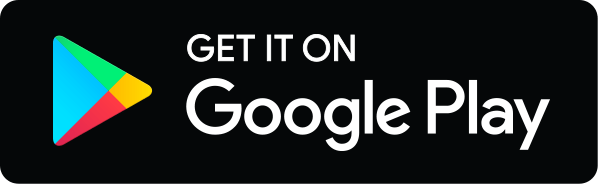