Cancer type/Site
Diagnoses/100 hospitalizations
VTE
PE
DVT
Pancreas
4.3
1.2
3.5
Brain
3.5
1.0
2.8
Myeloproliferative, other lymphatic/hematopoetic
2.9
b
2.5
Stomach
2.7
0.7
2.3
Lymphoma, lymphosarcoma, reticulosarcoma
2.5
0.6
2.0
Uterus
2.2
0.5
1.8
Trachea, bronchus, and lung
2.1
0.6
1.6
Esophagus
2.0
b
1.3
Prostate
2.0
0.6
1.6
Rectum, rectosigmoid junction, anus
2.1
0.7
1.4
Kidney
2.0
0.5
1.6
Colon
1.9
0.6
1.4
Ovary
2.0
0.5
1.6
Liver, gallbladder, intra- and extrahepatic ducts
1.8
0.9
1.1
Leukemia
1.7
0.4
1.4
Breast (female)
1.7
0.4
1.3
Cervix
1.6
b
1.4
Bladder
1.0
0.3
0.8
Lip, oral cavity, pharynx
<0.6
b
b
No cancer
1.0
0.3
0.8
3 Pathogenesis of VTE in Patients with Brain Tumors
Virchow’s triad delineates the three most commonly cited pathogenic mechanisms for the development of VTE: venous stasis, a hyper-coaguable state, and endothelial damage. Most patients who develop VTE have at least one of these typical risk factors, and many have two or more.
Beyond Virchow’s triad, recent literature has suggested that a wide range of additional factors are related to the development of VTE in cancer patients [30]. Data on VTE development in brain tumor patients, however, are limited. When discussing the pathogenesis of VTE in brain tumor patients, it is convenient to categorize the risk factors for VTE development by dividing them into patient-related, tumor-related, and host tissue-related mechanisms [5, 31, 32].
VTE is far more common during the post-operative period than it is in the general population [33–41]. Proposed explanations for this phenomenon include limited post-operative mobility, which can promote venous stasis, and damage to endothelial tissue. So from one stand point, brain tumor patients are at risk for VTE simply by undergoing an operation. Additionally, certain procedures confer more VTE risk than others. Orthopedic procedures, for example, increase the risk of VTE development: Day et al. recently reported a VTE rate of 1.2 % for lower extremity arthroplasties, and 0.53 % for shoulder arthroplasties [42]. Craniotomy, the most common operation for brain tumor resection, has also been implicated in increasing VTE risk compared to other operations [43]. Several studies have also shown that patients harboring brain tumors develop DVT at a higher rate than patients with cancer at other sites or patients undergoing procedures for diseases other than cancer (Table 1) [10, 29].
Patient with brain tumors are also more commonly afflicted with motor deficiencies that can render them bed ridden or immobile [44]. This limited mobility can result in relatively slower venous blood flow, one of the chief risk factors for VTE development [32, 45]. The use of indwelling central venous catheters for delivery of chemotherapeutic agents involved in the treatment of brain tumors can also contribute to the development of DVTs and PEs [46]. Patient specific genetic factors, such as factor V Leiden or an excess of pro-thrombotic factors can also be implicated in VTE development in brain tumor patients [24].
Many other risk factors have been reported as potential contributors to the development of VTE [44] (Table 2). Incidence of DVT and PE almost double when comparing those aged 65–69 to those above 85 years of age [47]. A similar trend is seen in a study comparing those aged 30–39 to younger patients with a twofold increase in the risk of VTE [48]. Traditionally, patients above the age of 40 are considered at a higher risk of VTE development [49]. A BMI >25–35 has also been implicated as a risk factor for VTE in other studies, along with increased operative duration, increased post-operative complications (all-types), and increased hospital stay [50, 51]. Gender, post-operative ICU days, anti-coagulation state, and blood type have also been studied as potential factors related to VTE in cancer patients [24, 42, 49]. Steroids and some chemotherapy protocols, often prescribed after cranial surgeries for brain tumors, have also been reported to contribute to VTE formation [44, 51].
Table 2
Factors associated with VTEs in patients with brain tumors and their corresponding odds ratios
Factora | Odds Ratio | 95 % Confidence Interval | P-Value |
---|---|---|---|
Increase the likelihood | |||
Intraluminal thrombosis (ILT) [11] | 17.8 | [4–79.3] | <0.0001 |
Sex (Female) [24] | 14.2 | [3.3–62.0] | <0.001 |
Perivascular Lymphocytic infiltrates (moderate – severe) [11] | 12.0 | N/R | <0.05 |
Prior history of DVT/PE [24] | 7.6 | [1.6–35.8] | 0.01 |
Prior history of DVT/PE [20] | 7.1 | N/R | <0.001 |
Necrosis (moderate – severe) [11] | 4.4 | N/R | <0.05 |
Seizure [21] | 2.4 | N/R | 0.005 |
Decrease the likelihood | |||
Ethnicity (Caucasian) [24] | 0.5 | [0.3–0.9] | 0.04 |
Post-operative ICU days (1 Day earlier exit) [24] | 0.2 | [1.1–1.4] | 0.003 |
Beyond these general risk factors for patients harboring brain tumors, there are many mechanisms specific to brain tumors that increase the risk of VTE development, most notably increased production of tissue factor (TF) and other pro-coagulants [21, 24, 52]. A study by Bastida et al. demonstrated that micro-vesicles from the cell-free supernatant of U-87 MG (a cell line of human glioblastoma cells) results in platelet aggregation and coagulation [52]. This is clinically correlated with a higher rate of VTE in patients with gliomas, one of the most common types of brain tumor [21, 24]. Khorana et al., in a series of pancreatic cancer patients, demonstrated that increased plasma TF correlates with the formation of VTE [53]. This has been suggested as a possible mechanism through which malignancies increase the risk of VTE [54].
Other studies have investigated this, but found negative immuno-histochemical staining for TF in a glioma specimen. Thus the models supporting this hypothesis (linking the increase of TF in brain tumors to VTE) remain limited to cell cultures, and are not in-vivo models [52, 55].
Another brain tumor-specific risk factor for VTE development are TF-bearing micro-particles (TFMP). In a group of 96 patients with advanced malignancies including glioblastoma, increased levels of TFMP tissue factor antigens were found to be 3.72 times more likely to result in VTE [56]. In 2011, Sartori et al. studied the pro-coagulant activity of circulating MPs in patients harboring glioblastoma multiforme (GBM). They found that MP activity levels increased in 63.6 % of 61 patients who underwent resection of GBM, a statistically significant association (chi2 = 4.93, p = 0.026) [57] (Table 3).
Table 3
DVT development by brain tumor typea
Tumor type | DVT+/total patients (%) |
---|---|
Metastasis | 44/185 (23.8) |
High Grade Glioma | 53/248 (21.4) |
Low Grade Glioma | 5/28 (17.6) |
Meningioma | 16/196 (8.2) |
High Grade Oligodendroglioma | 3/15 (20.0) |
Low Grade Oligodendroglioma | 2/16 (12.5) |
Mixed | 3/9 (33.3) |
Sarcoma | 0/3 (0.0) |
Schwanomma | 4/22 (18.2) |
Acoustic Neuroma | 0/1 (0.0) |
Medulloblastoma | 0/6 (0.0) |
Lymphoma | 8/27 (29.6) |
Pituitary Adenoma | 0/10 (0.0) |
Ependymoma | 0/6 (0.0) |
Hemangiopericytoma | 1/4 (25.0) |
Choroid | 0/3 (0.0) |
Hemangioblastoma | 2/9 (22.2) |
Other | 15/88 (17.0) |
Host tissues also contribute to the formation of VTE in patients with brain tumors. Collagen-rich endothelial membranes stimulate platelet aggregation, and thus the collection of white blood cells and ultimately VTE formation [31, 58]. Animal models also demonstrate that tumor interaction with platelets can lead to aggregation [59]. Both local and systemic tissue inflammation are also thought to take part in development of VTE through inflammatory markers, such as C-reactive protein (CRP), which is considered a potential indicator of the presence of VTE [60]. Evidence also suggests the involvement of tumor necrosis factor (TNF), a known inflammatory mediator, in the process of VTE development [61]. In addition to inflammation, disruption of the endothelium during operations leads to a pro-thrombotic state via the release of pro-thrombotic factors [31]. Cell adhesion molecules like P-selectin and E-selectin also promote leukocyte margination and adhesion [31]. P-selectins found in alpha granules of platelets and Weibel-Palade bodies of endothelial cells increase tissue factor expression and were found to be pro-thrombotic in animal models [62]. This has been clinically correlated by a study that displayed their increased presence in patients with cancer who developed VTE [63].
4 Presenting Signs and Symptoms
VTE presentation in afflicted patients can vary widely depending on the region involved. In some patients, VTE (especially DVT) can be asymptomatic. The most common presenting symptoms for DVT include pain and edema of the affected limb [64–66]. Symptoms can also include severe tenderness of the extremity, a palpable mass, blanching of the skin, and excessive warmth or redness in the extremity [23, 64, 67, 68]. PE, on the other hand, commonly present with signs and symptoms that include dyspnea, pleuritic pain, cough, hemoptysis, and palpitation [64, 69]. The location of these presenting symptoms can greatly aid in diagnosis and localization of the thrombus.
Definitive diagnosis of VTE often requires radio-graphical investigation. A low threshold of suspension should provoke the work up for DVT, which typically involves an upper/lower extremity ultrasound to locate the embolus [38, 69]. Well’s score is the most widely used tool for assessment of likelihood of VTE occurrence, despite the availability of other scoring systems [70]. A systematic review of 17 articles evaluating the use of such scores reported a median positive likelihood ratios of 6.62, 1, and 0.22 when patients had a high, moderate, and low pretest probability for the use of Well’s score, respectively [71]. Another study showed that the use of compression ultrasonography had a negative predictive value of 97–98 % after a normal result, which rose to 99 % after serial testing in an outpatient setting [72]. In a recent report, basing the choice of investigation on symptoms and clinical signs in such clinical scores was found to be less accurate than the use of biomarkers. For instance, clinical signs were found to be statistically less useful in aiding the diagnosis of DVT (Area under the curve (AUC): 0.69), as compared to serum protein S (AUC: 0.82) and albumin (AUC: 0.80) [73].
In the case of PE, however, workup is more complex and should include radiographic imaging techniques such as chest CT scans and V/Q scans, depending on the risk and the profile of the patient [21, 66, 67, 74]. The use of Well’s score was similarly useful for detection of PE as DVT, with median positive likelihood ratios of 6.75, 1.82, and 0.13 when patients had a high, moderate, and low pretest probability, correspondingly [71]. Helical spiral computed tomography (CT) has a negative prediction value of >99 % to diagnosing PE, and can thus safely replace the gold standard of angiography [72].
5 Prophylaxis and Treatment
There are multiple modalities available for physicians to achieve thrombo-prophylaxis in their patients. The methods of achieving thrombo-prophylaxis can be broadly divided into two categories: pharmacological or mechanical. The ideal method depends on multiple factors, and physicians should always aim to achieve thrombo-prophylaxis without conferring additional health risks to patients. Viewing the patient holistically, physicians can determine how to tailor the ideal method of thrombo-prophylaxis based on several factors, including prior history of VTE, presence of a malignancy, pertinent contraindications to anti-coagulation, and the overall type of patient (e.g. medical vs. surgical; neurosurgical vs. general surgical).
These factors, in addition to the risk factors mentioned earlier, may guide physicians towards optimal prophylaxis. For general surgical patients, the Modified Caprini risk assessment model for VTE can be used to categorize patients by risk from very low to high [75, 76]. Patients with a high Modified Caprini risk (≥5) are estimated to have a six percent chance of developing VTE if left untreated. Based on this risk score, an appropriate method of prophylaxis is then prescribed.
A scoring system like the Modified Caprini scale has not been developed or validated specifically for neurosurgical patients. Based on evidence from observational studies, however, the nature of neurosurgical procedures and central nervous system malignancy are both significant contributors to risk of VTE [54]. Craniotomy patients are thus considered at high risk for acquiring VTE. Furthermore, brain tumor patients undergoing craniotomy have a higher risk for developing VTE compared to patients undergoing craniotomy for other pathologies (e.g., elective aneurysm surgery).
An important complicating factor in thromboprophylaxis for neurosurgical patients is the risk of intracranial hemorrhage (ICH). Patients undergoing transcranial operations for tumors or vascular lesions are already at an increased risk for hemorrhage. The addition of anti-coagulant medication has been proposed as a potential risk factor for higher rates of hemorrhage among neurosurgical patients. Data on this association have been mixed. Some studies have demonstrated an increased rate of intracranial hemorrhage when VTE chemoprophylaxis is initiated pre-operatively [77]. In contrast, other studies have failed to demonstrate any evidence of increased hemorrhage risk with VTE chemoprophylaxis [78–81]. One study estimated that craniotomy patients have approximately 1 % risk for developing ICH in the absence of thromboprophylaxis [82]. Other studies report even a lower risk [83]. Despite this relatively low risk, the consequences of ICH are great (e.g., disability, paresis or paralysis, speech impediment, diminished neurological function, or death), emphasizing the need to approach medical prophylaxis thoughtfully.
5.1 Pharmacological Prophylaxis
The use of anticoagulants in the prophylaxis of VTE is extremely common. Several medical options are available and involve the administration of one of the following drugs:
Low-Dose Unfractionated Heparin
Low-dose unfractionated heparin interrupts the coagulation cascade through augmenting the function of antithrombin III, which in turn affects the activity of factor Xa and IIa [84]. In a meta-analysis of general surgical patients, Clagett et al. demonstrated that the administration of low-dose unfractionated heparin was effective in preventing the formation of DVT [84, 85]. Another study, however, has demonstrated that heparin is ineffective in preventing VTE in critically injured patients [86]. Overall, evidence from meta-analyses shows significant reduction of VTE risk, with some studies reporting a decrease of fatal PE by 60 % compared to no prophylaxis [87]. Patients given unfractionated heparin should have their platelets monitored in order to detect heparin-induced thrombocytopenia (HITT), a complication that presents as a consumptive coagulopathy [88]. In case of overdose, protamine sulfate is used as an antidote to unfractionated heparin [89].
Low Molecular Weight Heparin (LMWH)
LMWH has been studied extensively for reducing the rate of VTE development. It has a similar mechanism of action as unfractionated heparin, working to interrupt the coagulation cascade through antithrombin III. It is however different in that it mainly inhibits the action of factor Xa. Meta-analyses have shown that LMWH is at least as efficacious as unfractionated heparin for reducing VTE [75]. Advantages LMWH has over unfractionated heparin is that can be administered subcutaneously, and has a much lower incidence of HITT. This makes it generally more effective in the clinical setting.
In brain tumor patients, what is more important is not choosing between LMWH or unfractionated heparin, but whether the method of chemoprophylaxis should be used at all. Several trials have compared the efficacy of the two drugs, showing similar results [83] (Table 4). As we have discussed previously, these agents will reduce the risk of VTE at the cost of potentially increasing the risk of ICH. In brain tumor patients undergoing craniotomy, administering LMWH or unfractionated heparin is safe and indicated, as the benefit of reducing the already much higher VTE risk outweighs the increase in risk for developing ICH. Other pharmacological alternatives exist, some of which are becoming more commonly used. These include fondaparinux, warfarin, asprin, and novel direct thrombin/factor Xa inhibitors.
Table 4
Occurrence of hemorrhagic complications with various pharmacological VTE prophylactic regimens in patients with brain tumors
Pharmacological drug | Hemorrhagic complications % in treatment group | Hemorrhagic complications % controls group | P-value |
---|---|---|---|
Heparin (5000 units, BD, SC) / Enoxaparin (40 mg, OD) [21] | 0.00 % | 1.30 % | – |
Heparin (5000 units, BD, SC) [103] | 2.00 % | N/R | – |
Heparin (5000 units, BD, SC) | 3.78 % | N/R | – |
Dalteparin (2500 units, OD) [103] | 4.00 % | N/R | – |
Nadroparin [104] | 2.50 % | 0.80 % | 0.87 |
CY 216 (Fraxiparin) [105] | 19.8 % | N/R | – |
Pooled data analysisa | RR | 95 % CI | P-Value |
UFH vs Control [83] | 0.50 | (0.11–2.38) | – |
LMWH vs UFH [83] | 1.46 | (0.61–3.51) | – |
5.2 Mechanical Prophylaxis
The second approach available to achieve thrombo-prophylaxis is through mechanical methods. The two most common forms of mechanical prophylaxis for VTE are intermittent pneumatic compression (IPC) and compression stockings [90].
It is thought that IPC not only reduces venous stasis, but also stimulates endogenous fibrinolytic activity [91]. This is likely because of a reduction in plasminogen activator inhibitor-1 (PAI-1), resulting in an increased fibrinolytic activity through tissue plasminogen activator (tPA) activity [91]. In a systematic meta-analysis, Vanek et al. demonstrated that IPC decreased the risk of DVT by 62 % compared to a placebo, 47 % compared to high-pressure stockings, and 48 % compared to low molecular weight heparin [92]. In a randomized trial of neurosurgery patients, Turpie et al. corroborated this data by reporting the incidence of DVT at 8.8 % in patients using high-pressure stockings, 9 % in patients using IPC, and 19.8 % in an untreated control group [93]. Kurtoglu et al. similarly reported that IPC was as effective as low molecular weight heparin in DVT prophylaxis following head and spinal trauma [84]. Although effective at preventing DVT, Vanek et al. report that IPC and compression stockings have no effect on the rate of PE formation [92]. Overall, IPC has limited adverse effects, and is an alternative for patients who are contra-indicated to receive anticoagulants.
Compression stockings have been studied less than IPC, but nevertheless have been demonstrated to be effective in preventing DVT. One meta-analysis showed that compression stockings reduced the risk of developing DVT by 65 % compared to no prophylaxis [94]. Most often, compression stockings are combined with other thrombo-prophylactic measures.
Because paresis and limited post-operative mobility are often reported as risk factors for the development of DVT, post-operative ambulation is encouraged for prophylactic purposes. Intervention with physical therapy for patients who are not ambulatory is often employed as an additional prophylactic. Post-operative ambulation and exercise can help prevent venous stasis, which contributes to the development of VTE as described above [34, 36, 49, 85, 95].
5.3 Combined Prophylaxis
Because both mechanical and chemical prophylaxis are effective at reducing the rate of VTE development during the post-operative period, they are frequently prescribed simultaneously. Several studies have compared the effectiveness of chemical anticoagulation with the effectiveness of mechanical prophylaxis [14, 40, 77, 84, 86, 96, 97]. Many of these studies show that combined prophylaxis has a higher effectiveness in preventing VTE than either method alone, without increasing risk to the patient (Table 5) [97].
Table 5
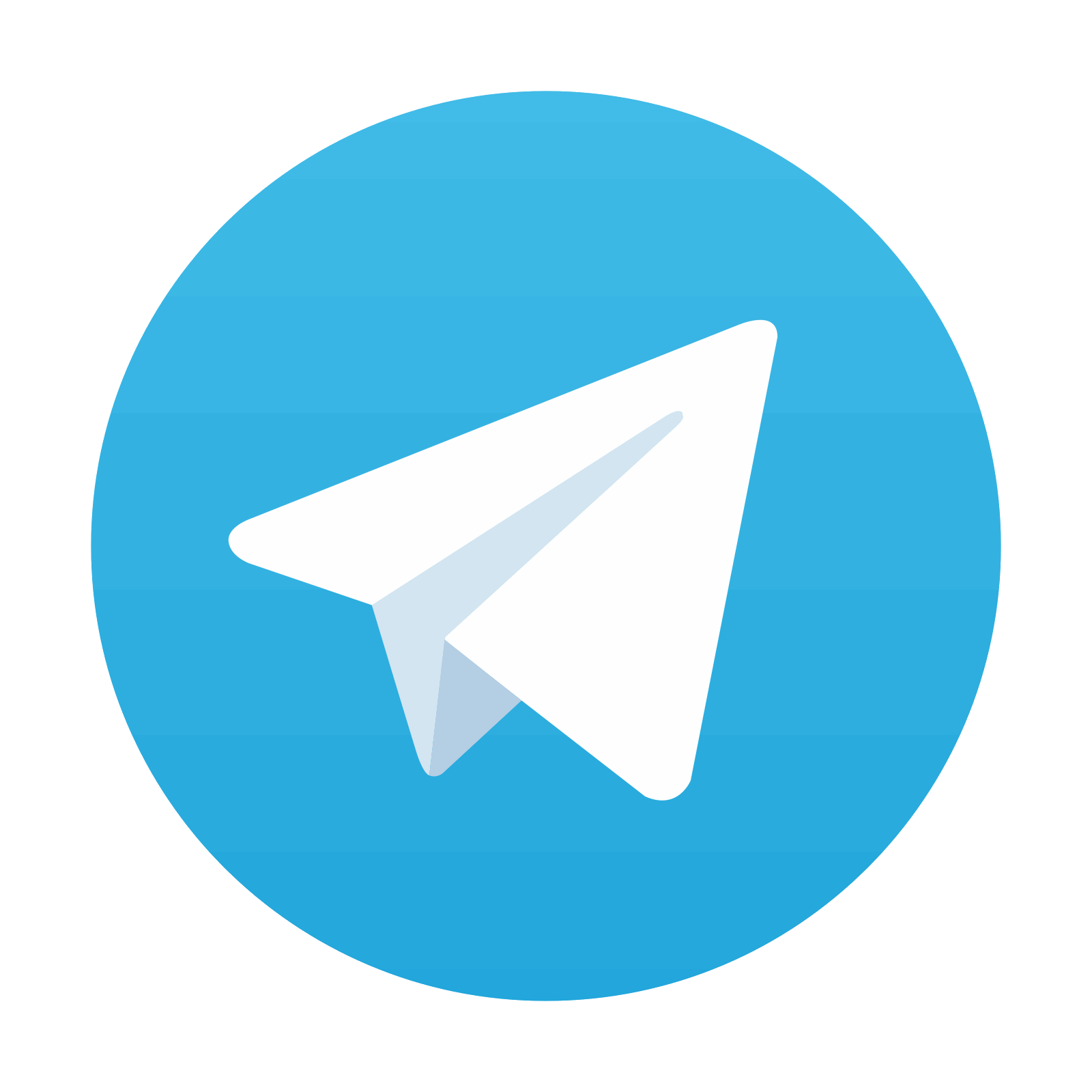
Mechanical prophylaxis efficacy compared to pharmacological prophylactic regimens for VTEs in patients with brain tumors
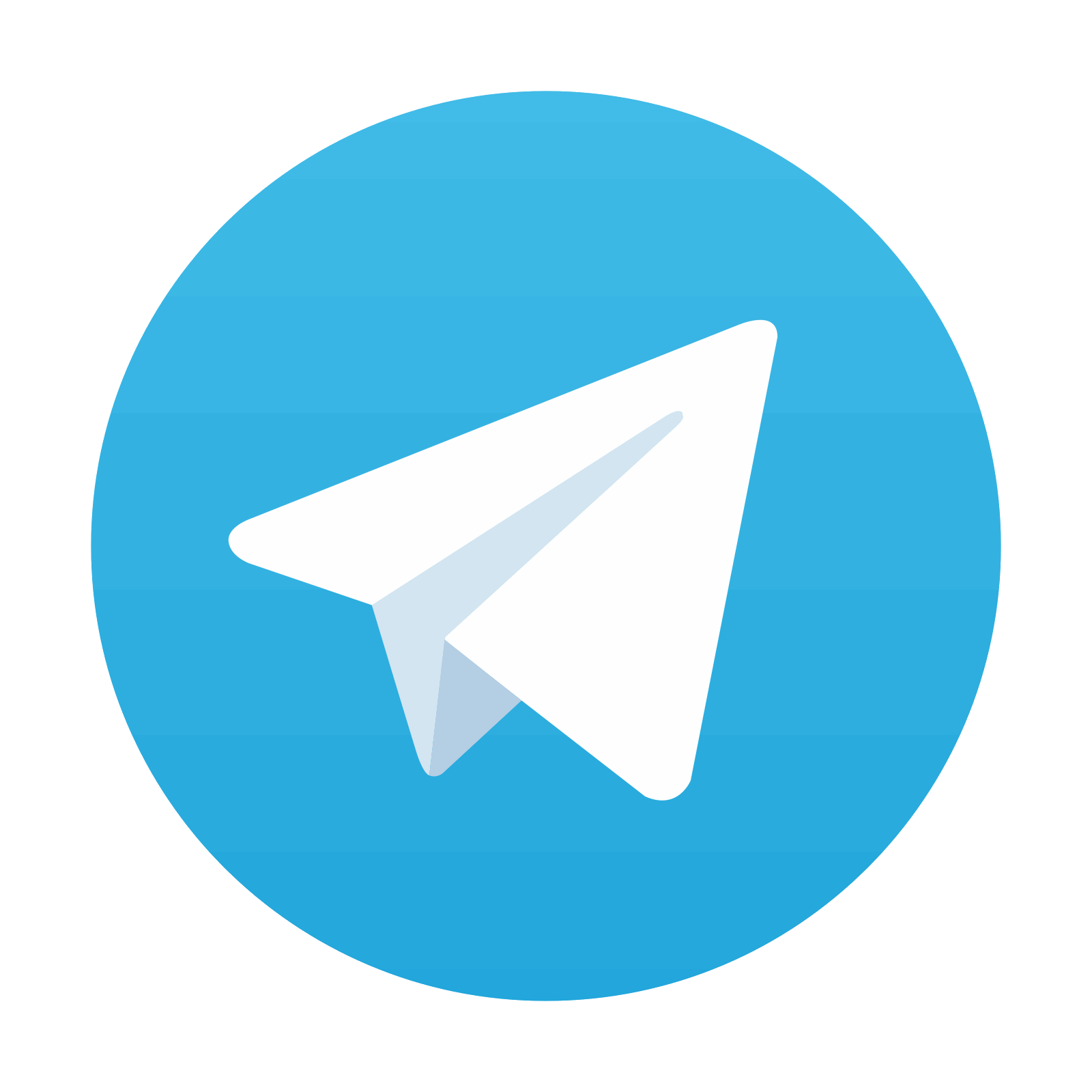
Stay updated, free articles. Join our Telegram channel

Full access? Get Clinical Tree
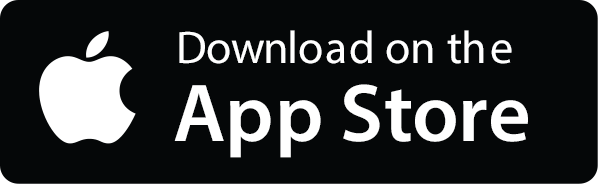
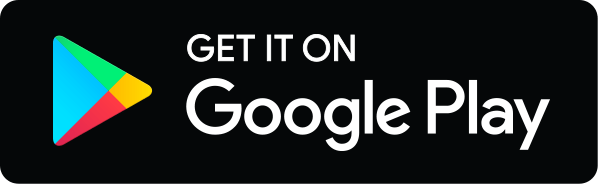
